Echogenicity
Size
Diabetic nephropathy
Normal
Normal or increased
Obesity
Normal
Increased
Acromegaly
Normal
Increased
Lymphoma/leukaemia
Normal
Increased
Glomerulonephritis
Normal or increased
Increased
Vasculitis
Normal or increased
Increased
Tubulointerstitial disease
Normal or increased
Increased
Amyloid
Normal or increased
Increased
Multiple myeloma
Normal or increased
Increased
HIV nephropathy
Normal or increased
Increased
Pre-eclampsia
Normal or increased
Increased
Pyelonephritis
Normal or decreased
Increased (often unilateral)
Renal vein thrombosis
Normal or decreased
Increased (often unilateral)
Urinary tract obstruction
Normal or decreased
Increased (often unilateral)
Medullary nephrocalcinosis
Increased (medulla)
Normal
Urate nephropathy
Increased (medulla)
Normal
Sickle cell disease
Increased (medulla)
Normal or decrease
Sjögen syndrome
Increased (medulla)
Normal or decrease
Medullary sponge kidney
Increased (medulla)
Normal
Oedema
Decreased (cortex)
Increased
Acute cortical necrosis
Decreased (cortex)
Normal or decrease
Of note, chronic kidney disease can lead to decrease in cortical thickness although this sign lacks sensitivity and no clear cut-off exists. Several kidney diseases are more specifically associated with changes in medulla echogenicity. Nephrocalcinosis is characterized by increased medullary echogenicity due to calcium deposit, as well as sickle cell disease and gout.
While normal kidney length is about 11 cm (the left kidney being about 0.3 mm longer than the right kidney), there is an expected atrophy with ageing. It should be mentioned as well that height and weight also positively correlate with kidney size.
A goal of ultrasonography examination in B-mode is also to detect urinary tract obstruction as the cause of AKI. Urinary tract obstruction is involved in 1–15 % of cases of AKI, although it remains a relative rare cause of AKI in ICU patients. It should be especially suspected when a clinical suspicion exists (such as flank pain, urolithiasis, neurogenic bladder, benign prostatic hyperplasia, pelvic cancer, single functional kidney, pelvic surgery), or when the clinical course of AKI is not rapidly favourable despite treatment. While caliceal dilatation suggests urinary tract obstruction, false-negative findings on echo can be observed especially in hypovolemic patients or in patients with retroperitoneal tumours or fibrosis or with early obstruction. Repeated exams can help in detecting such patients especially after volume repletion (except for retroperitoneal fibrosis or tumours in which alternative methods must be used, i.e. CT scan or MRI).
False-positive findings include pregnancy, diabetes insipidus, vesicoureteral reflux, after relief of obstruction, megacystic-megaureter syndrome, full bladder, urinary tract infection. All these conditions are often associated with caliceal dilation.
Finally, ultrasound examination with Doppler can be used by experienced laboratory personnel to screen for renal artery stenosis or to detect vascular abnormalities (arterial stenosis or vein thrombosis), e.g. in renal transplants recipients.
10.1.2 Doppler-Based Resistive Index (RI)
Renal Doppler has also been suggested as a useful tool in evaluating intra-renal perfusion in various settings [2–8]. Hence, intra-renal Doppler-based renal resistive index (RI) has been tested to assess renal allograft status [9, 10] and changes in renal perfusion in critically ill patients [11–13] and for predicting the reversibility of an acute kidney injury (AKI) [14, 15].
10.1.2.1 Methods
Although 2- to 5-MHz transducers are optimal to measure RI [16, 17], various transducers may be successfully used for this purpose, including small phased array transducer. The first step is a B-mode US with a postero-lateral approach allowing location of the kidneys and detection of signs of chronic renal damage. Subsequently, colour Doppler or power Doppler US allows vessels’ localization (Fig. 10.1a) [16] and may allow a semi-quantitative evaluation of renal perfusion (Table 10.2) [18].
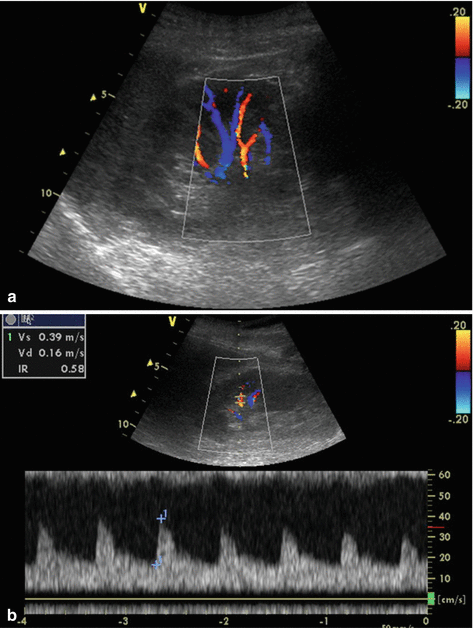
Fig. 10.1
Results of a renal colour Doppler ultrasonography showing renal vascularization (a). RI measurement using pulsed wave Doppler (b)
Table 10.2
Colour Doppler for a semi-quantitative evaluation of intra-renal vascularisation [18]
Stage |
Quality of renal perfusion by colour Doppler |
---|---|
0 |
Unidentifiable vessels |
1 |
Few vessels in the vicinity of the hilum |
2 |
Hilar and interlobar vessels in most of the renal parenchyma |
3 |
Renal vessels identifiable until the arcuate arteries in the entire field of view |
Either the arcuate arteries or the interlobar arteries are then insonated with pulsed wave Doppler using a Doppler gate as low as possible between 2- and 5-mm [16, 17]. In order to obtain repeatable measures, the waveforms should be optimized for the measurements using the lowest pulse repetition frequency (usually 1.2–1.4 kHz) without aliasing (to maximize waveform size), the highest gain without obscuring background noise, and the lowest wall filter [16, 17]. A spectrum is considered optimal when three to five consecutive similar-appearing waveforms are noted [16, 17]. To characterize the intra-renal Doppler waveform, most investigators have used the resistive index (RI) so-called Pourcelot Index (Fig. 10.1b).
Three to five reproducible waveforms are obtained, and RIs from these waveforms are averaged to compute the mean RI for each kidney. This easily calculated parameter is defined as:
RI = [peak systolic shift – minimum diastolic shift]/peak systolic shift
Renal pulsatility index may also be calculated:
PI = [peak systolic velocity – minimum diastolic velocity]/mean velocity
10.1.2.2 Normal Values, Feasibility and Reproducibility
RI can theoretically range from 0 to 1. RI is normally lower than 0.70. In several studies, mean RI (±SD) in healthy subjects ranged from 0.58 (±0.05) to 0.64 (±0.04) [20, 21]. The normal RI range is, however, age dependent. Thus, RI values greater than 0.70 have been described in healthy children younger than 4 years [22] and in individuals older than 60 years and considered healthy [23]. When the RI is measured for both kidneys, the side-to-side difference is usually less than 5 % [24].
Renal RI is a simple and non-invasive tool easy to use at the patient bedside. Feasibility of the measure has been showed to be good, even in the settings of critically ill patients. A recent study suggested a half-day course to be sufficient to allow inexperienced operators in successfully measuring RI [25]. Inter-observer reproducibility of RI measurement by senior radiologist or senior intensivist is considered excellent [14, 26]. In critically ill patients, the inter-observer reproducibility between senior and inexperienced operator is good and measures seem accurate (absence of systematic bias) although associated with a lack of precision (wide 95 % confidence interval of ±0.1) [25].
10.1.2.3 Significance and Usual Confounders
Both physiological and clinical significance of the RI remains debated. Initially considered an indicator of renal vascular resistance and blood flow [7], both experimental and clinical studies have demonstrated correlation of RI with vascular resistance and blood flow to be weak [27, 28]. Thus, observed RI changes in response to supra-physiological pharmacologically induced changes in renal vascular resistance are modest (RI changes of 0.047 IU (±0.008) per logarithmic increase in renal resistances) [29]. Both in vitro and ex-vivo studies however demonstrated a strong relationship between vascular compliance (vascular distensibility) and RI [27–29]. This strong relationship between vascular compliance and RI has been confirmed in a recent large cohort of renal allograft [10]. In this line, age-related arterial stiffening may explain the progressive increase in RI with age [30]. Similarly, elevated RI observed in several pathological states such as diabetes mellitus and hypertension may also be related to the influence of these diseases on arterial stiffness and to sub-clinical vascular changes related to the underlying disease [31, 32].
Macrovascular hemodynamic changes also influence RI. Hence, pulse pressure index [(systolic pressure – diastolic pressure/systolic pressure)] had direct and dramatic effects on RI values [29]. Additionally, since RI depends in part on the minimum diastolic shift, it may be influenced by the heart rate [33]. According to observations performed by Mostbeck and colleagues regarding RI changes as consequences of heart rate variations, a formula has been developed to correct the RI value for heart rate: [Corrected RI = observed RI −0.0026 × (80-heart rate)] [33]. This formula has, however, never been validated in clinical studies.
In addition to these factors, both oxygen and carbon dioxide levels can affect RI. Several studies have demonstrated that RI varies according to PaO2 and PaCO2 levels [34–36]. These studies performed in healthy subjects, patients with chronic obstructive respiratory disease, renal transplant recipients or patients with acute respiratory distress syndrome suggest that hypoxemia and hypercapnia may increase RI [34–36].
Besides vascular and hemodynamic factors, kidney interstitial pressure has been shown to be associated with RI in ex vivo studies [28]. An increase in interstitial pressure reduces the transmural pressure of renal arterioles, thereby diminishing arterial distensibility and, consequently, decreasing overall flow and vascular compliance. Similarly, intra-abdominal pressure may affect RI via the same mechanisms. Thus, incremental changes in intra-abdominal pressure correlated linearly with RI in a porcine model [37], and reduction in intra-abdominal pressure with paracentesis was followed by a decrease in RI in cirrhotic patients with tense ascites [38]. Finally, ureteral pressure, likely acting via interstitial pressure, also affects RI [6].
These numerous confounders suggest RI to be an integrative parameter rather than reliable tool to assess renal perfusion or a substitute for renal biopsy.
10.1.2.4 Clinical Relevancy in ICU
Doppler-based RI has been suggested to monitor renal perfusion in critically ill patients, detect early renal dysfunction in severe sepsis patients or in assessing prognosis of AKI.
Renal Doppler has also been proposed to monitor renal perfusion in critically ill patients [12]. In recent studies, RI was used to assess the impact on renal perfusion of low-dose dopamine infusion and gradual changes in mean arterial pressure in response to norepinephrine infusion in critically ill patients [11, 13]. Despite significant results, the observed RI variations were modest and their real impact on renal perfusion and moreover on renal function remains unclear. Assuming that RI may reflect renal perfusion, it was recently proposed for the early detection of occult hemorrhagic shock in a small study conducted in normotensive trauma patients [39]. If patients with occult hemorrhagic shock had higher RI, they also had higher lactate levels and lower base excess. Although these findings are promising, the exact significance remains uncertain. Hence, as mentioned above, RI is influenced not only by vascular resistance but also by many other parameters such as age, heart rate, mean arterial pressure, changes in renal perfusion, vascular compliance, and renal interstitial oedema and interstitial pressure [27–29]. A study is currently ongoing in way to more clearly underline potential interest of Doppler-based RI in assessing renal perfusion (DORESEP; NCT01473498).
Additionally, several studies assessed interest of Doppler-based RI in detecting early renal dysfunction or in predicting short-term reversibility of AKI [14, 15, 25, 40, 41]. In a study conducted in septic critically ill patients, RI measured at admission was higher in patients who developed subsequently AKI [14]. This finding was recently confirmed in the post-operative setting of cardiopulmonary bypass [42]. Additionally, several cohort studies suggest Doppler-based RI to be differentiating transient from persistent AKI in selected critically ill patients [15, 41, 43]. Interestingly, semi-quantitative renal perfusion assessment seems to be correlated with Doppler-based RI and associated with reversibility of renal dysfunction [25]. Despite these promising results, most of these studies were performed in limited patient samples which may have overestimated diagnostic performance [15, 43–45]. Additionally, a recent study has identified discrepant results regarding RI diagnostic performance in this setting [44]. Therefore despite the promising preliminary reports, we still lack adequately powered study validating performance of RI in both early detection of renal insult or AKI prognostic assessment.
10.1.3 Contrast-Enhanced Ultrasonography
Contrast-enhanced US (CEUS) relies on the intravascular injection of specific contrast agents that create a signal of high echogenicity thus allowing macro and microvascular structure visualization when using specific imaging techniques. These specific contrast agents consist in gas-filled microbubbles that oscillate in response to US waves therefore creating a non-linear signal of high echogenicity (Fig. 10.2) [46]. This technique is believed to allow an accurate quantification of regional and global renal blood flow [47]. It has been validated in humans to evaluate coronary blood flow [48], and its safety has been largely documented in this context [49]. When adding this technique to recently developed softwares, this technique is believed to allow an accurate quantification of regional blood flow, such as renal blood flow [47]. A recent study has confirmed feasibility of this technique in cardiac surgery patients [50]. The clinical interest of this technique remains however theoretical and validation studies are needed. A recent study raised doubt regarding the interest of CEUS in estimating renal perfusion [51]. Hence, in this study, noradrenaline-induced increases in mean arterial pressure were not associated with a change in overall CEUS derived mean perfusion indices [51]. Additionally, an important heterogeneity in responses was noted among the 12 included patients. Additional studies are ongoing and should help in more clearly assessing input of this technique in clinical setting and reliability of CEUS in assessing renal perfusion.
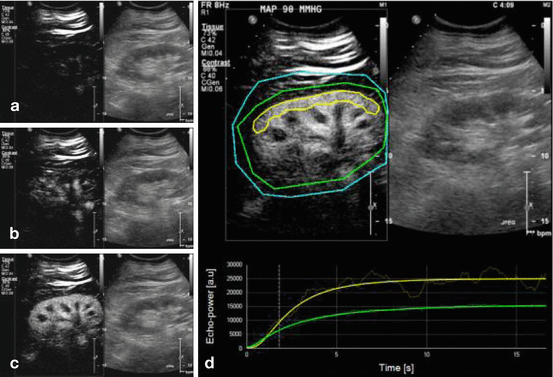
Fig. 10.2
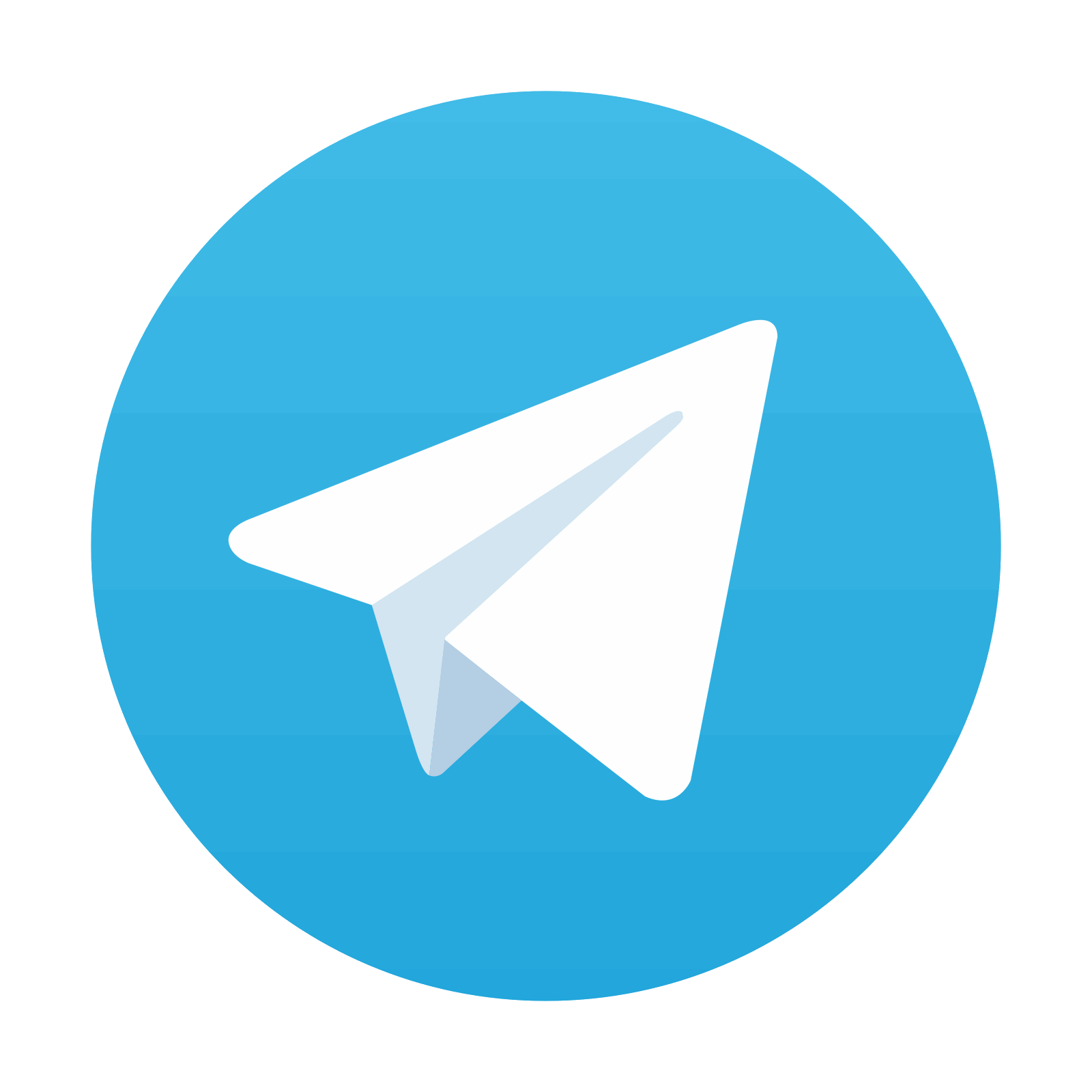
Illustration of contrast-enhanced ultrasonography. During continuous infusion of the contrast agent, microbubble destruction is obtained by applying pulses at high mechanical index (high ultrasound intensity). Microcirculation replenishment is then observed. All images represent renal contrast-enhanced ultrasonography (CEUS), the left part of the image shows contrast-image mode imaging and the right part the standard (B-mode) image. (a) Immediately after the flash; (b) during replenishment (2 s after the flash); (c) at full replenishment (6 s after the flash); (d) sequence analysis with Sonotumor®: a region of interest was drawn (yellow line) in the largest possible area of renal cortex closer to the ultrasound probe. The software generates a time intensity curve. This curve is used to generate CEUS-derived parameters (Reproduced from Schneider et al. Crit Care 2013 [50] with permission)
Only gold members can continue reading. Log In or Register to continue
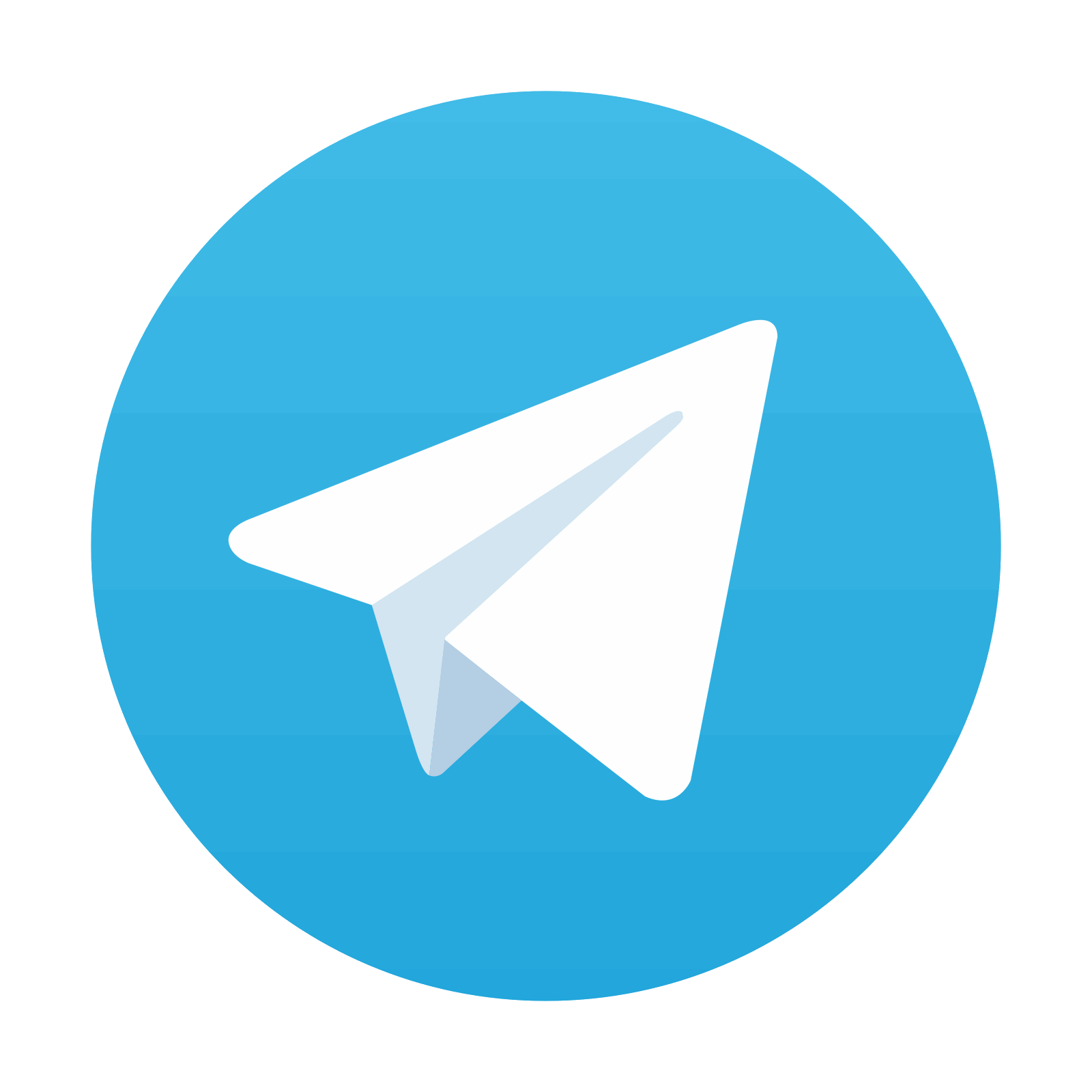
Stay updated, free articles. Join our Telegram channel

Full access? Get Clinical Tree
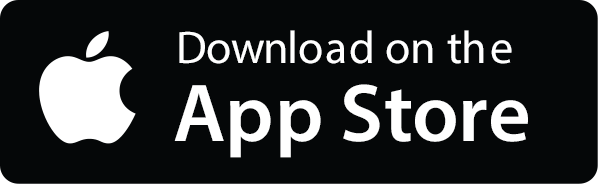
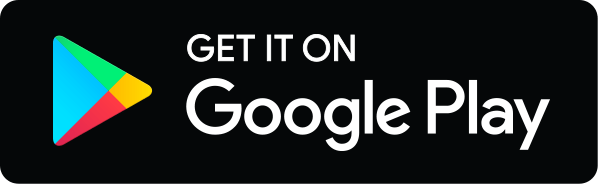