Fig. 3.1
Cellular model for Na+ entry into the first half (early part) of the proximal tubule. Note that many of these mechanisms are also present in the second half (later part) of the proximal tubule. Passive transport of Na+ is indicated by broken arrow
Another active mechanism of Na+ entry is through the Na/H exchanger, which pumps Na+ into and H+ out of the cell. It is this exchanger that is responsible for most H+ secretion and Na+ reabsorption in the proximal tubule. During the process of H+ secretion, HCO3 − is generated and reabsorbed (see Fig. 3.1). Na/H exchanger is mediated by the NHE3 (sodium–hydrogen exchanger isoform 3) protein. Inhibition of Na/H exchanger by acetazolamide decreases NaCl reabsorption .
Na+ extrusion from the cell into the peritubular capillaries is accomplished by the Na/K-ATPase, which transports three Na+ ions out and two K+ ions into the cell. In this way, the intracellular Na+ concentration in the proximal tubule is maintained around 15–35 mEq/L. In addition, Na+ also exits with Na/HCO3 cotransporter. The organic solutes leave the cell by passive transport mechanisms .
Cl− Reabsorption
The second phase of NaCl reabsorption occurs in the late portion of the proximal tubule. As with Na+, reabsorption of Cl− occurs by both active and passive transport mechanisms. There are no Cl− transporters in the early proximal tubule. Therefore, the concentration of Cl− increases as the filtrate moves along the proximal tubule. Thus, the active transport of Cl− becomes dominant in the late proximal tubule. Cl− entry occurs via Na/Cl cotransport as well as an exchange between the luminal Cl− and cellular anions such as formate, oxalate, sulfate or HCO3 −. Although the tubular concentrations of either formate or oxalate are substantially low, they combine with the H+ secreted by the Na/H exchanger to form formic acid or oxalic acid in the lumen . These acids are converted into their bases inside the cell, which then return to the tubular lumen via Cl/formate or Cl/oxalate exchangers (Fig. 3.2). Thus, a substantial amount of Na+ and Cl− is reabsorbed through Na/H and other exchangers.

Fig. 3.2
Cellular model for Na+ coupled Cl− entry into the second half (later part) of the proximal tubule. Note that many of these transport mechanisms are also present in the first half of the proximal tubule. Broken arrow at the basolateral membrane indicates Cl− conductance channel
Passive Cl− reabsorption occurs via the tight junctions. Because of reabsorption of glucose and amino acids, the lumen becomes slightly negative with the development of an electrical gradient. This lumen-negative voltage favors passive Cl− reabsorption. Paracellular Cl− transport is a major mechanism for Cl− reabsorption. Cl− leaves the cell via Cl− conductance channel and K/Cl cotransporter.
Thin Limbs of Henle’s Loop
The fluid that leaves the proximal tubule and enters the thin descending limb (TDL) of Henle’s loop is isosmotic (about 300 mOsm/kg H2O) to plasma. The TDL is highly permeable to water and relatively less permeable to solutes (Na+, Cl−, K+, and urea). Water, therefore, moves out of the tubule into the surrounding interstitium. The TDL is abundant in aquaporin (AQP)-1 water channel proteins. As a result, the concentration of the tubular fluid increases. In long-looped nephrons, the tubular fluid osmolality increases gradually from 300 to 1,200 mOsm/kg H2O at the tip of the papilla. The transport characteristics of the thin ascending limb (TAL) of Henle’s loop are different from those of the TDL. The TAL is impermeable to water, moderately permeable to urea, and highly permeable to NaCl. Urea diffuses into and NaCl diffuses out of the tubular lumen. Since water does not diffuse out, the tubular fluid is diluted and the osmolality decreases. The decreased osmolality of the tubular fluid creates a large osmolality difference between the tubular lumen and the surrounding medullary interstitium, which has a high osmolality.
Thick Ascending Limb of Henle’s Loop
About 30 % of the filtered NaCl is reabsorbed into the thick ascending limb of the Henle’s loop (TALH) . The TALH is virtually impermeable to water but highly permeable to NaCl . This combination of virtually low permeability to water and high reabsorptive rates of NaCl makes the tubular fluid more dilute with a low NaCl concentration. Because the tubular fluid is diluted (hypotonic) in the TALH, it is often referred to as the diluting segment. The osmolality of the tubular fluid at the end of the TALH is about half (150 mOsm/kg H2O) of that of plasma. Both TAL and TALH lack aquaporins.
The reabsorption of NaCl at the apical membrane occurs by a secondary active transport process. The principal transporter, called the Na/K/2Cl cotransporter, transports one Na+, one K+and two Cl− into the cell (Fig. 3.3). Because of Cl− movement, the tubular lumen has a positive potential difference as opposed to the negative potential difference in the proximal tubule. Also, the K+ that enters the cells diffuses back into the tubular lumen via ROMK (renal outer medullary potassium) channels. It is important that K+ be returned to the tubular lumen for reabsorption of Na+ via the Na/K/2Cl cotransporter. When this recycling of K+ is inhibited by blocking ROMK channel, NaCl transport decreases substantially .

Fig. 3.3
Cellular model for Na/K/2Cl cotransport and for the transport of other cations in the thick ascending limb of Henle’s loop. Broken arrows indicate diffusion of respective ions through specific conductance channels. ROMK renal outer medullary potassium channel. Note that loop diuretics inhibit Na/K/2Cl cotransporter
Three types of K+ channels have been identified: a low conductance 30 pS (picosieman) channel, an intermediate 70 pS channel, and a high conductance calcium-activated maxi K+ channel; the latter participates little to the net K+ transport. Thus, 30 pS and 70 pS channels make up the ROMK and account for most of the K+ that diffuses into the lumen in the TALH.
The exit of Cl− at the basolateral membrane is accomplished by two mechanisms. One involves the exit through conductive Cl− channel, and this movement is facilitated by the negative intracellular voltage. The second mechanism involves coupling of Cl− with K+ and their exit as K/Cl cotransport (see Fig. 3.3). Several Cl− conductance (ClC) channels have been identified. Of these, ClC-ka and ClC-kb are expressed at the basolateral membranes of TALH and TAL. ClC-ks require another protein called barttin for their expression in these segments of the nephron. It is the ClC-kb/barttin channel that mediates most of the Cl− efflux across the basolateral membrane of the TALH.
In addition to NaCl, many cations such as Ca2 +, Mg2 + and NH4 + are reabsorbed in the TALH. This reabsorption occurs mainly through the tight junctions and intercellular pathways (see Fig. 3.3). The driving force for this paracellular pathway seems to be the positive transepithelial potential difference created by two Cl− and one Na+ transport and also by backleak of K+ into the tubular lumen. Blocking of this K+ recycling inhibits both Ca2 + and Mg2 + reabsorption. NH4 + can replace K+ and enter the cell through Na/K/2Cl cotransporter.
Another mechanism for Na+ transport is the Na/H exchanger (NHE3), which is located in the apical membrane of the TALH. As in the proximal tubule, this mechanism generates HCO3 − in the TALH and exits via Na/HCO3 cotransporter.
Distal Tubule
In this section, the early and late distal convoluted tubule (DCT) and the connecting tubule (CNT) are discussed together as the distal tubule . The DCT represents the segment of the nephron beyond the macula densa. Both DCT and CNT reabsorb approximately 10 % of the filtered load of NaCl. Na+ is actively transported across the apical membrane by three mechanisms (Fig. 3.4). First, NaCl reabsorption occurs by an electroneutral Na/Cl cotransporter, which is inhibited by a thiazide diuretic. Second, Na+ enters the cell by an electrogenic epithelial Na+ channel (ENaC). The ENaC consists of three homologous subunits (α, β, and γ). All three subunits are required for ENaC activity. This Na+ reabsorption via ENaC creates a negative potential difference in the lumen, which drives some Cl− across the paracellular pathway and K+ secretion via ROMK channel. Na+ transport through ENaC is inhibited by amiloride. Third, NaCl transport involves parallel Na/H exchanger and Cl/base exchanger with the recycling of H+ and base. The base exchanged with Cl− appears to be either formate or oxalate. Na+ leaves the cell via Na/K-ATPase. It is suggested that Na+ reabsorption in the early DCT is largely mediated by Na/Cl cotransporter, while Na+ reabsorption in the late DCT is largely mediated by the ENaC. Cl− and K+ are transported across the basolateral membrane by Cl− and K+ conductance channels. The DCT is impermeable to water. The mechanisms for NaCl transport in CNT seem to be similar to those of the DCT, except for the ENaC which is the primary Na+ transport pathway in CNT .
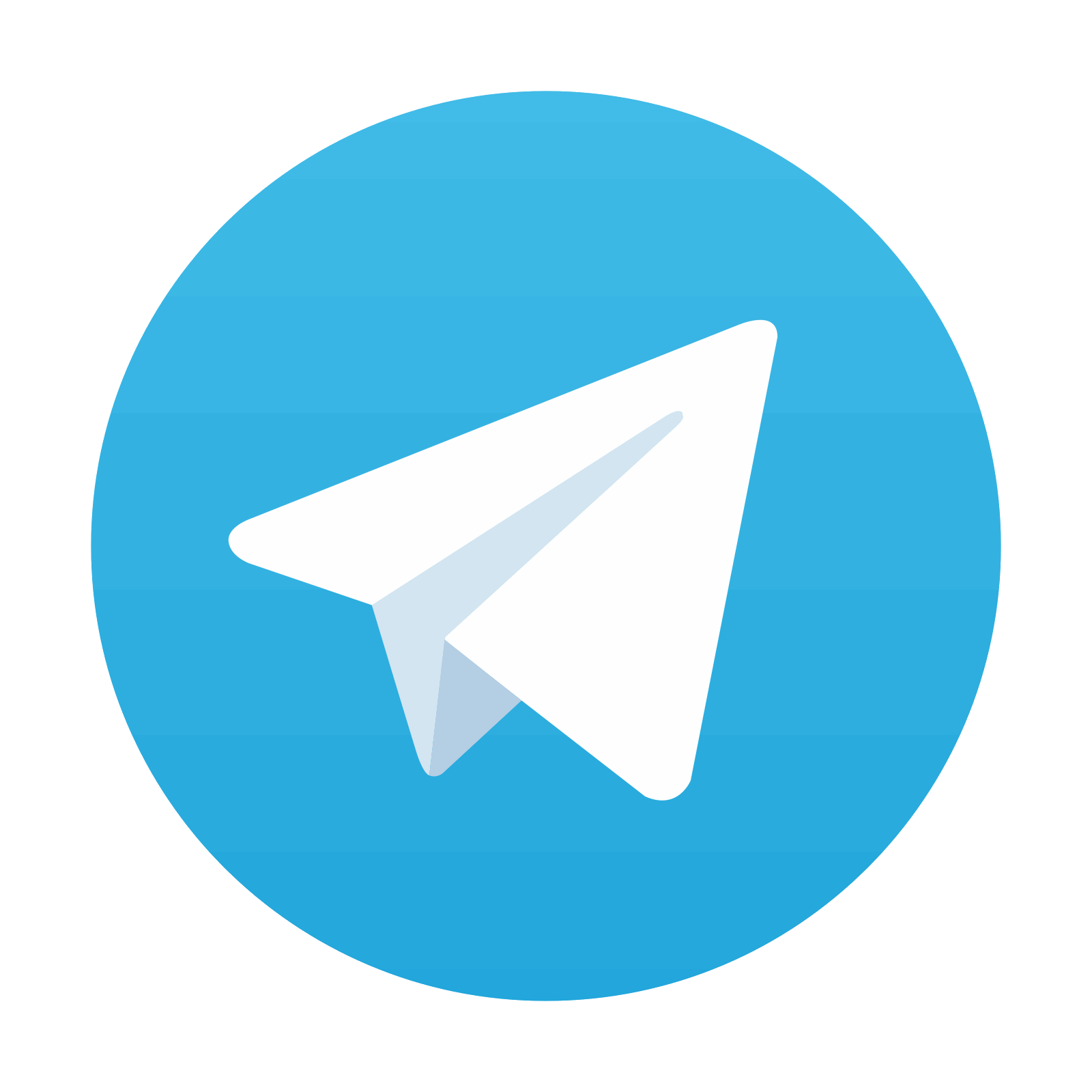
Stay updated, free articles. Join our Telegram channel

Full access? Get Clinical Tree
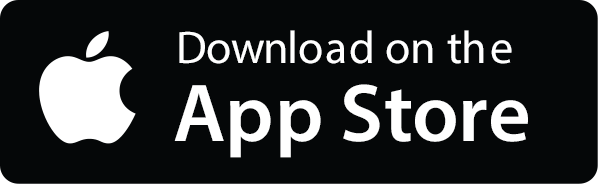
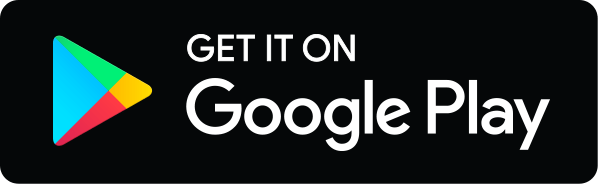