Objectives
- •
Describe the four major functions of the gastrointestinal (GI) tract.
- •
Understand the differences between and significance of endocrine, paracrine, and neurocrine agents.
- •
Identify the major GI hormones, their functions, sites of release, and stimuli for release.
- •
Identify the important neurocrines and their functions in the GI tract.
- •
Identify the important paracrines and their functions in the GI tract.
- •
Understand the causes and resulting physiology of gastrinoma (Zollinger-Ellison syndrome) and pancreatic cholera (Werner Morrison syndrome).
The functions of the gastrointestinal (GI) tract are regulated by peptides, derivatives of amino acids, and a variety of mediators released from nerves. All GI hormones are peptides, but it is important to realize that not all peptides found in digestive tract mucosa are hormones. The GI tract peptides can be divided into endocrines, paracrines, and neurocrines, depending on the method by which the peptide is delivered to its target site.
Endocrines , or hormones , are released into the general circulation and reach all tissues (unless these substances are excluded from the brain by the blood-brain barrier). Specificity is a property of the target tissue itself. Specific receptors, which recognize and bind the hormone, are present on its target tissues and absent from others. There are five established GI hormones; in addition, some GI peptides are released from endocrine cells into the blood but have no known physiologic function. Conversely, several peptides have been isolated from mucosal tissue and have potent GI effects, but no mechanism for their physiologic release has been found. Members of these latter two groups are classified as candidate hormones.
Paracrines are released from endocrine cells and diffuse through the extracellular space to their target tissues. Their effects are limited by the short distances necessary for diffusion. Nevertheless, these agents can affect large areas of the digestive tract by virtue of the scattered and abundant distributions of the cells containing them. A paracrine agent can also act on endocrine cells. Thus a paracrine may release or inhibit the release of an endocrine substance, thereby ultimately regulating a process remote from its origin. Histamine, a derivative of the amino acid histidine, is an important regulatory agent that acts as a paracrine.
Some GI peptides are located in nerves and may act as neurocrines or neurotransmitters. A neurocrine is released near its target tissue and needs only to diffuse across a short synaptic gap. Neurocrines conceivably may stimulate or inhibit the release of endocrines or paracrines. Acetylcholine (ACh), although not a peptide, is an important neuroregulator in the GI tract. One of its actions is to stimulate acid secretion from the gastric parietal cells.
General Characteristics
The GI tract is the largest endocrine organ in the body, and its hormones were the first to be discovered. The word hormone was coined by W. B. Hardy and used by Starling in 1905 to describe secretin and gastrin and to convey the concept of bloodborne chemical messengers. The GI hormones are released from the mucosa of the stomach and small intestine by nervous activity, distention, and chemical stimulation coincident with the intake of food. Released into the portal circulation, the GI hormones pass through the liver to the heart and back to the digestive system to regulate its movements, secretions, and growth. These hormones also regulate the growth of the mucosa of the stomach and small and large intestines, as well as the growth of the exocrine pancreas.
The GI peptides have many different types of actions. Their effects on water, electrolyte, and enzyme secretion are well known, but they also influence motility, growth, and release of other hormones, as well as intestinal absorption. Many of these actions overlap; two or more GI peptides may affect the same process in the same direction, or they may inhibit each other. Many of the demonstrated actions of these peptides are pharmacologic and do not occur under normal circumstances. This chapter is concerned primarily with the physiologic effects of the GI peptides.
The actions of the GI peptides also may vary in both degree and direction among species. The actions discussed in the remainder of this chapter are those occurring in humans.
Discovery
Four steps are required to establish the existence of a GI hormone. First, a physiologic event such as a meal must be demonstrated to provide the stimulus to one part of the digestive tract that subsequently alters the activity in another part. Second, the effect must persist after all nervous connections between the two parts of the GI tract have been severed. Third, from the site of application of the stimulus a substance must be isolated that, when injected into the blood, mimics the effect of the stimulus. Fourth, the substance must be identified chemically, and its structure must be confirmed by synthesis.
Five GI peptides have achieved full status as hormones. They are secretin, gastrin, cholecystokinin (CCK), gastric inhibitory peptide (GIP), and motilin. There is also an extensive list of “candidate” hormones whose significance has not been established. This list includes several chemically defined peptides that have significant actions in physiology or pathology but whose hormonal status has not been proved. These are pancreatic polypeptide, neurotensin, and substance P. In addition, two known hormones, glucagon and somatostatin, have been identified in GI tract mucosa; their possible function as GI hormones is currently being investigated. Some of these peptides function physiologically as paracrines or neurocrines. Another GI peptide, Ghrelin , is released from the body of the stomach and functions as a hormone to regulate food intake. This topic is covered in Chapter 13 .
Secretin, the first hormone, was discovered in 1902 by Bayliss and Starling and was described as a substance, released from the duodenal mucosa by hydrochloric acid, that stimulated pancreatic bicarbonate and fluid secretion. Jorpes and Mutt isolated it and identified its amino acid sequence in 1966. It was synthesized by Bodanszky and coworkers later the same year.
Edkins discovered gastrin in 1905, stating to the Royal Society that “in the process of the absorption of digested food in the stomach a substance may be separated from the cells of the mucous membrane which, passing into the blood or lymph, later stimulates the secretory cells of the stomach to functional activity.” For 43 years investigators were preoccupied by the controversy over the existence of gastrin. The debate intensified when Popielski demonstrated that histamine, a ubiquitous substance present in large quantities throughout the body (including the gastric mucosa), was a powerful gastric secretagogue. In 1938 Komarov demonstrated that gastrin was a polypeptide and was different from histamine. By 1964 Gregory and his colleagues had extracted and isolated hog gastrin; Kenner and his group synthesized it the same year. After 60 years all of the criteria for establishing the existence of a GI hormone had been satisfied.
In 1928 Ivy and Oldberg described a humoral mechanism for the stimulation of gallbladder contraction initiated by the presence of fat in the intestine. The hormone was named cholecystokinin after its primary action. The only controversy involving CCK was a mild one over nomenclature. In 1943 Harper and Raper described a hormone released from the small intestine that stimulated pancreatic enzyme secretion and accordingly named it pancreozymin. As Jorpes and Mutt carried out the purification of these two substances in 1968, it became obvious that both properties resided in the same peptide. For the sake of convenience and because it was the first action described, this hormone is called CCK .
In 1969 Brown and his coworkers described the purification of a powerful enterogastrone from intestinal mucosa. Enterogastrone literally means a substance from the intestine (entero-) that inhibits (-one) the stomach (gastr-). By 1971 this peptide had been purified, isolated, sequenced, and named gastric inhibitory peptide for its ability to inhibit gastric secretion. Released from the intestinal mucosa by fat and glucose, GIP also stimulates insulin release. Following proof that the release of insulin was a physiologic action of the peptide, GIP became the fourth GI hormone. The insulinotropic effect of GIP requires elevated amounts of serum glucose. For this reason, and because it is doubtful whether the inhibitory effects of the peptide on the stomach are physiologic, it has been suggested that its name be changed to glucose-dependent insulinotropic peptide . In either case, it is still referred to as GIP .
Brown and his coworkers also described the purification of motilin in the early 1970s. Motilin is a linear 22–amino acid peptide purified from the upper small intestine. During fasting it is released cyclically and stimulates upper GI motility. Its release is under neural control and accounts for the interdigestive migrating myoelectric complex.
Chemistry
The GI hormones and some related peptides can be divided into two structurally homologous families. The first consists of gastrin ( Fig. 1.1 ) and CCK ( Fig. 1.2 ). The five carboxyl-terminal (C-terminal) amino acids are identical in these two hormones. All the biologic activity of gastrin can be reproduced by the four C-terminal amino acids. This tetrapeptide, then, is the minimum fragment of gastrin needed for strong activity and is about one-sixth as active as the whole 17–amino acid molecule. The sixth amino acid from the C-terminus of gastrin is tyrosine, which may or may not be sulfated. When sulfated, the hormone is called gastrin II . Both forms occur with equal frequency in nature. The amino-terminus (N-terminus) of gastrin is pyroglutamyl, and the C-terminus is phenylalamide (see Fig. 1.1 ). The NH 2 group following Phe does not signify that this is the N-terminus; rather, it indicates that this C-terminal amino acid is amidated. These alterations in structure protect the molecules from aminopeptidases and carboxypeptidases and allow most of them to pass through the liver without being inactivated.


Gastrin is first synthesized as a large, biologically inactive precursor called progastrin. A glycine-extended ( G-Gly ) form of gastrin is then formed by endoproteolytic processing within the G cells. G-Gly is the substrate for an amidation reaction that results in the formation of the mature, amidated gastrin. The C-terminal amide moiety is required for full biologic activity mediated by gastrin/CCK-2 receptors. Receptors for CCK and gastrin were originally called the CCK-A and CCK-B receptors, respectively; the nomenclature has been changed to CCK-1 and CCK-2. G-Gly does not stimulate the gastrin/CCK-2 receptor but instead activates its own receptor, thus leading to the activation of Jun kinase and trophic effects.
CCK, which has 33 amino acids, contains a sulfated tyrosyl residue in position 7 from the C-terminus (see Fig. 1.2 ). CCK can activate gastrin receptors (e.g., those for acid secretion, also called CCK-2 receptors); gastrin can activate CCK receptors (e.g., those for gallbladder contraction, also called CCK-1 receptors). Each hormone, however, is more potent at its own receptor than at those of its homologue. CCK is always sulfated in nature, and desulfation produces a peptide with the gastrin pattern of activity. The minimally active fragment for the CCK pattern of activity is therefore the C-terminal heptapeptide.
In summary, peptides belonging to the gastrin/CCK family having a tyrosyl residue in position 6 from the C-terminus or an unsulfated one in position 7 possess the gastrin pattern of activity and act on CCK-2 receptors—strong stimulation of gastric acid secretion and weak contraction of the gallbladder. Peptides with a sulfated tyrosyl residue in position 7 act on CCK-1 receptors, have cholecystokinetic potency, and are weak stimulators of gastric acid secretion. Obviously the tetrapeptide itself and all fragments less than seven amino acids long possess gastrin-like activity.
The second group of peptides is homologous to secretin and includes vasoactive intestinal peptide (VIP), GIP, and glucagon , in addition to secretin ( Fig. 1.3 ). Secretin has 27 amino acids, all of which are required for substantial activity. Pancreatic glucagon has 29 amino acids, 14 of which are identical to those of secretin. Glucagon-like immunoreactivity has been isolated from the small intestine, but the physiologic significance of this enteroglucagon has not been established. Glucagon has no active fragment, and, like secretin, the whole molecule is required before any activity is observed. Evidence indicates that secretin exists as a helix; thus the entire amino acid sequence may be necessary to form a tertiary structure with biologic activity.

GIP and VIP each have nine amino acids that are identical to those of secretin. Each has many of the same actions as those of secretin and glucagon. This group of peptides is discussed in greater detail later in the chapter.
Most peptide hormones are heterogeneous and occur in two or more molecular forms. Gastrin, secretin, and CCK all have been shown to exist in more than one form. Gastrin was originally isolated from hog antral mucosa as a heptadecapeptide (see Fig. 1.1 ), which is now referred to as little gastrin (G 17). It accounts for 90% of antral gastrin. Yalow and Berson demonstrated heterogeneity by showing that the major component of gastrin immunoactivity in the serum was a larger molecule that they called big gastrin. On isolation, big gastrin was found to contain 34 amino acids; hence it is called G 34. Trypsin splits G 34 to yield G 17 plus a heptadecapeptide different from G 17. Therefore G 34 is not simply a dimer of G 17. An additional gastrin molecule (G 14) has been isolated from tissue and contains the C-terminal tetradecapeptide of gastrin. Current evidence indicates that most G 17 is produced from pro G 17 and most G 34 is derived from pro G 34. Thus G 34 is not a necessary intermediate in the production of G 17.
During the interdigestive (basal) state, most human serum gastrin is G 34. Unlike those of other species, the duodenal mucosa of humans contains significant amounts of gastrin. This is primarily G 34 and is released in small amounts during the basal state. After a meal, a large quantity of antral gastrin, primarily G 17, is released and provides most of the stimulus for gastric acid secretion. Smaller amounts of G 34 are released from both the antral and the duodenal mucosa. G 17 and G 34 are equipotent, although the half-life of G 34 is 38 minutes and that of G 17 is approximately 7 minutes. The plasma concentration of gastrin in fasting humans is 10 to 30 pmol/L, and it doubles or triples during the response to a normal mixed meal.
Distribution and Release
The GI hormones are located in endocrine cells scattered throughout the GI mucosa from the stomach through the colon. The cells containing individual hormones are not clumped together but are dispersed among the epithelial cells. The nature of this distribution makes it virtually impossible to remove the source of one of the GI hormones surgically and examine the effect of its absence without compromising the digestive function of the animal.
The endocrine cells of the gut are members of a widely distributed system termed amine precursor uptake decarboxylation (APUD) cells. These cells all are derived from neuroendocrine-programmed cells originating in the embryonic ectoblast.
The distributions of the individual GI hormones are shown in Fig. 1.4 . Gastrin is most abundant in the antral and duodenal mucosa. Most of its release under physiologic conditions is from the antrum. Secretin, CCK, GIP, and motilin are found in the duodenum and jejunum.

Ultrastructurally, GI endocrine cells have hormone-containing granules concentrated at their bases, close to the capillaries. The granules discharge, thereby releasing their hormones in response to events that are either the direct or the indirect result of neural, physical, and chemical stimuli associated with eating a meal and the presence of that meal within the digestive tract. These endocrine cells have microvilli on their apical borders that presumably contain receptors for sampling the luminal contents.
Table 1.1 lists the stimuli that are physiologically important releasers of the GI hormones. Gastrin and motilin are the only hormones shown to be released directly by neural stimulation. Protein in the form of peptides and single amino acids releases both gastrin and CCK. Fatty acids containing eight or more carbon atoms or their monoglycerides are the most potent stimuli for CCK release. Fat must be broken down into an absorbable form before release of CCK, thus providing evidence that the receptors for release are triggered during the process of absorption.
Hormone | |||||
---|---|---|---|---|---|
Releaser | Gastrin | CCK | Secretin | GIP | Motilin |
Protein | S | S | 0 | S | 0 |
Fat | 0 | S | S- | S | S- |
Carbohydrate | 0 | 0 | 0 | S | 0 |
Acid | I | S- | S | 0 | S- |
Distention | S | 0 | 0 | 0 | 0 |
Nerve | S | 0 | 0 | 0 | S |
Evidence indicates that intestinal releasing factors secreted into the intestine of certain species, including humans, stimulate the release of CCK. Pancreatic enzymes inactivate these releasing factors. The ingestion and presence of a meal in the intestine result in temporary binding of trypsin and other proteases and allow the releasing factors to remain active and stimulate CCK secretion. Thus this mechanism acts as a negative feedback control on pancreatic enzyme secretion.
Carbohydrate, the remaining major foodstuff, does not alter the release of gastrin, secretin, or CCK to a significant extent but does stimulate GIP release. GIP also is released by fat and protein. The strongest stimulus for secretin release is H + . Secretin is released when the pH in the duodenum falls below 4.5. Secretin also is released by fatty acids. This may be a significant mechanism for secretin release because the concentration of fatty acids in the lumen is often high. CCK can also be released by acid, but except during hypersecretion of acid, the physiologic significance of this mechanism of release has not been established. The purely physical stimulus of distention activates antral receptors and causes gastrin release; for example, inflating a balloon in the antrum releases gastrin. During a meal the pressure of ingested food initiates this response. The magnitude of the response is not as great as originally believed, however, and the contribution of distention to the total amount of gastrin released in humans probably is minor. Gastrin also can be released by calcium, decaffeinated coffee, and wine. Pure alcohol in the same concentration as that of wine does not release gastrin but does stimulate acid secretion. Motilin is released cyclically (approximately every 90 minutes) during fasting. This release is prevented by atropine and ingestion of a mixed meal. Acid and fat in the duodenum, however, increase motilin release.
In addition to releasing secretin, acid exerts an important negative feedback control of gastrin release. Acidification of the antral mucosa below a pH of 3.5 inhibits gastrin release. Patients with atrophic gastritis, pernicious anemia, or other conditions characterized by the chronic decrease of acid-secreting cells and hyposecretion of acid may have extremely high serum concentrations of gastrin because of the absence of this inhibitory mechanism.
Hormones alter the release of GI peptides in several instances. Both secretin and glucagon, for example, inhibit gastrin release. CCK has been shown to stimulate glucagon release, and four GI hormones (secretin, gastrin, CCK, and GIP) increase insulin secretion. Elevated serum calcium stimulates both gastrin and CCK release. It is doubtful whether any of these mechanisms, with the exception of release of insulin by GIP, play a role in normal GI physiology. Some mechanisms, however, may become important when circulating levels of hormones or calcium are altered by disease.
Actions and Interactions
The effects of pure GI hormones have been tested on almost every secretory, motor, and absorptive function of the GI tract. Each peptide has some action on almost every target tested. Even though large doses of hormone sometimes are necessary to produce an effect, either stimulatory or inhibitory, these tests indicate that receptors for each hormone are present on most target tissues. The myriad activities possessed by these peptides are summarized in Table 1.2 .
Hormone | |||||
---|---|---|---|---|---|
Action | Gastrin | CCK | Secretin | GIP | Motilin |
Acid secretion | S | S | I | I | |
Gastric emptying | I | I | I | I | |
Pancreatic <SPAN role=presentation tabIndex=0 id=MathJax-Element-1-Frame class=MathJax style="POSITION: relative" data-mathml='HCO3−’>HCO−3HCO3− HCO 3 − secretion | S | S | S | 0 | |
Pancreatic <SPAN role=presentation tabIndex=0 id=MathJax-Element-2-Frame class=MathJax style="POSITION: relative" data-mathml='HCO3−’>HCO−3HCO3− HCO 3 − enzyme secretion | S | S | S | 0 | |
Bile <SPAN role=presentation tabIndex=0 id=MathJax-Element-3-Frame class=MathJax style="POSITION: relative" data-mathml='HCO3−’>HCO−3HCO3− HCO 3 − secretion | S | S | S | 0 | |
Gallbladder contraction | S | S | S | ||
Gastric motility | S | S | I | I | S |
Intestinal motility | S | S | I | S | |
Insulin release | S | S | S | S | |
Mucosal growth | S | S | I | ||
Pancreatic growth | S | S | S | ||
Pancreatic growth | S | S | S |
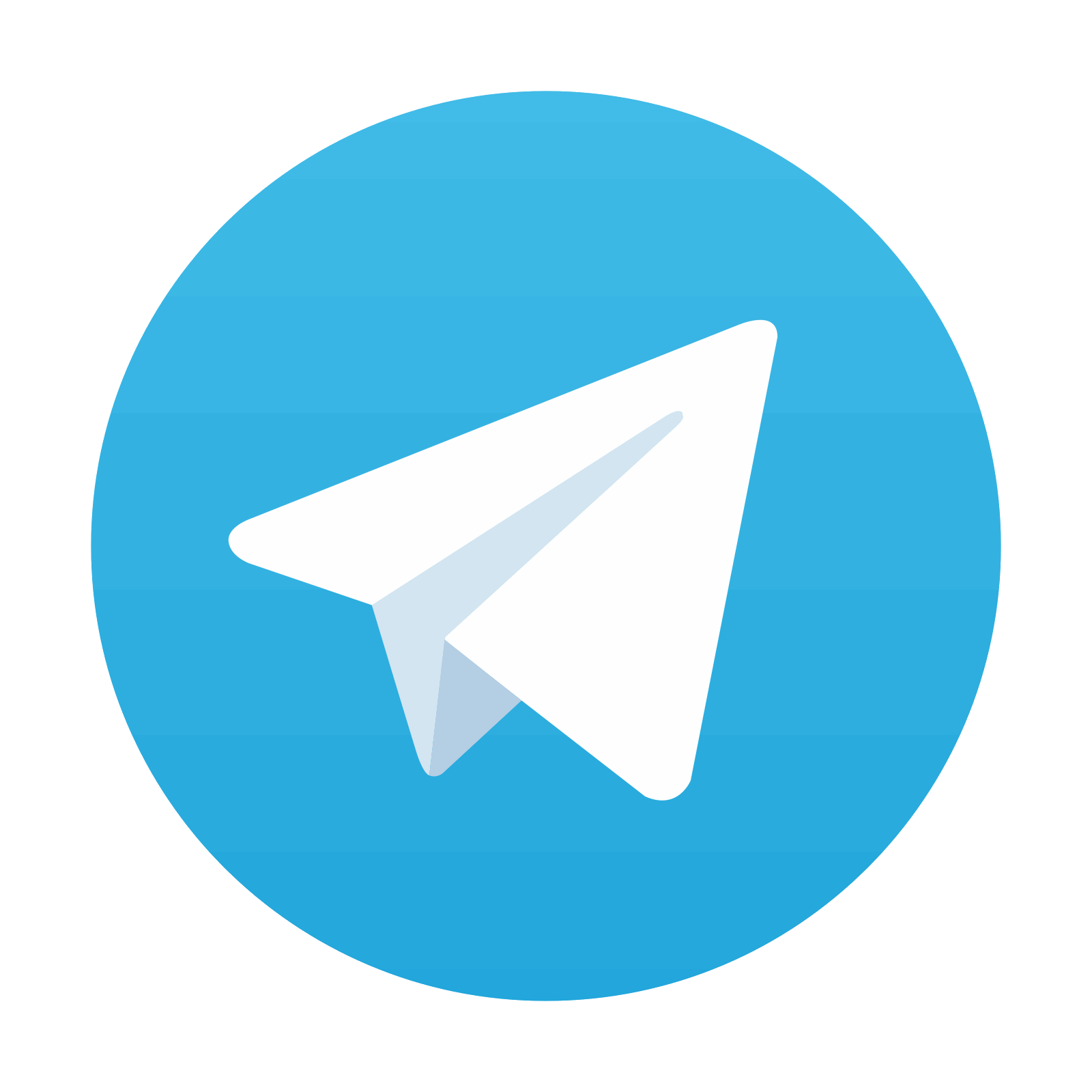
Stay updated, free articles. Join our Telegram channel

Full access? Get Clinical Tree
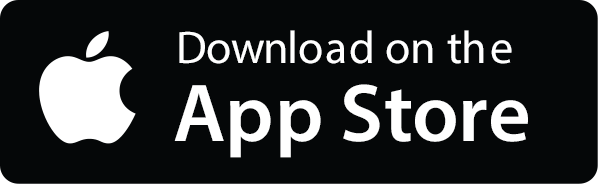
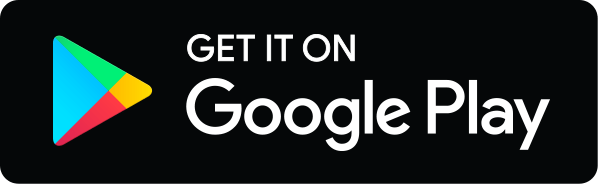
