Abstract
The recruitment of leukocytes from peripheral blood into the intestine is required for the maintenance of intestinal health and protection from microbial insult. However, during immune-mediated gastrointestinal conditions (e.g., inflammatory bowel disease (IBD), celiac disease), the recruitment process becomes dysregulated. Several families of molecules regulate the influx of these cells into immune sites both during homeostasis and inflammation. Interference with some of these molecules has already shown efficacy in the clinics and antibodies that target the molecules involved have been approved by the FDA for use in IBD. In this chapter, we discuss basic aspects of the diverse families of relevant molecules that mediate the leukocyte adhesion cascade under physiological and inflammatory conditions to relevant immune compartments. Finally, we discuss the translational applications of targeting-specific steps of the leukocyte adhesion cascade for therapeutic purposes in human IBD.
Keywords
Selectins, Integrins, Chemokines, Rolling, Adhesion, Transmigration, Inflammation, Inflammatory bowel disease
65.1
Introduction
The intestinal mucosa is unique among other tissues of the human body, as it exists in close proximity to an enormous number of microorganisms and their products. Such factors constitute a potential trigger for proinflammatory responses by the gut-associated lymphoid tissue (GALT) and a constant challenge to mucosal homeostasis. The latter is typically preserved by the function of a dense network of diverse, although highly interconnected, immunological checkpoints which require the continuous presence of patrolling cells of the innate and adaptive immunity. This state of “subclinical inflammation” is a hallmark of the intestinal microenvironment and, despite its name, ensures the absence of deleterious inflammatory responses during homeostasis. Nevertheless, under unfavorable genetic and/or environmental pressures, this delicate balance is occasionally broken, resulting in the development of “overt” intestinal inflammation. This is most dramatically exemplified in ulcerative colitis (UC) and Crohn’s disease (CD), two major types of persisting chronic intestinal inflammatory processes, which are collectively referred to as the inflammatory bowel diseases (IBDs). A prominent characteristic of such conditions is the remarkable expansion of the cellular infiltrate within the bowel wall, which is indicative of accelerated recruitment and increased retention of immune cells within the inflamed bowel. The circulation of immunocytes to diseased gut regions is a complex process that involves a tightly coordinated sequence of adhesive interactions between those cells and the endothelial lining of local blood vessels (i.e., the postcapillary venules).
When trafficking of immune cells to the gastrointestinal (GI) tract is considered, in addition to the pathophysiological status of the target tissue (homeostatic vs acute injury vs chronic inflammation), several additional parameters should be taken into account. Included among such factors are the regional specialization along the GI tract (upper GI vs ileum vs colon), cellular diversity of the infiltrating population (lymphocytic as opposed to innate immune cell recruitment, effector vs regulatory cells), molecular components of the adhesion cascade (GI specific or universal trafficking signaling mediators), as well as the specific microenvironment associated with the particular immunological reaction (i.e., the dominant mucosal inflammatory/immunological mediators).
This chapter provides a detailed description of the basic cellular and molecular mechanisms that mediate trafficking of immune cells to the healthy and inflamed bowel. An overview of the various adhesion molecules that are involved in cell-cell interactions is presented, followed by in-depth analysis of the basic mechanisms that mediate the movement of immune cells in the various structures of GALT. As leukocyte trafficking has recently arisen as a major therapeutic target in IBD, specific emphasis is placed on those interactions that have been or are being tested as potential treatment targets for IBD.
65.2
Overview of the Leukocyte Adhesion Cascade
Migration of circulating leukocytes to peripheral tissues usually occurs across the walls of postcapillary venules. Specialized endothelial cells that line these venules may be coated with chemoattractants and bear surface adhesion molecules that serve as mechanical anchors, thus facilitating the exit of leukocytes from the blood and their entrance into the tissues. This cell-cell adhesion is accomplished through homotypic or heterotypic receptor-ligand interactions. At the same time, adhesion proteins confer tissue specificity to the recruitment process, via selective patterns of expression by local vascular beds. Major classes of adhesion molecules that are involved in leukocyte recruitment include selectins and their glycoprotein ligands, as well as integrins and immunoglobulin superfamily molecules. All of these are type I transmembrane glycoproteins that span the cell membrane only once. In addition, cadherin, occludin, and claudin family of proteins have all been implicated in the adhesion cascades that take place in inflammatory sites. Table 65.1 reports several of these adhesion molecules along with their molecular function, tissue expression, and respective ligands. Myeloid cells and lymphocytes employ unique trafficking pathways, although several steps are common. Although trafficking occurs during homeostatic conditions, leukocyte recruitment is amplified in the presence of inflammation, as the original pathophysiological function of immunocytes was protection from infection. In the presence of inflammation, an orderly and well-regulated process takes place that allows for both rapid and sustained accumulation of inflammatory cells in affected tissues.
Adhesion Molecule | Primary Tissue Expression | Major Ligands | Primary Function |
---|---|---|---|
P-selectin | Endothelial cells, platelets | PSGL-1, Sialylated Lewis X | Leukocyte capture and rolling, thrombosis |
E-selectin | Endothelial cells | ESL-1, sialyated Lewis X | Leukocyte rolling and firm adhesion |
L-selectin | Neutrophils, monocytes, most lymphocytes | CD34, GLYCAM-1, MadCAM-1 | Leukocyte rolling and homing |
ICAM-1 | Endothelial and epithelial cells, lymphocytes, fibroblasts, and others | LFA-1, Mac-1, p150/95, fibrinogen, fibronectin (connecting segment-1) | Leukocyte adhesion and extravasation, leukocyte and endothelial cell motility, cell activation |
ICAM-2 | Endothelial cells, lymphocytes | LFA-1 | Leukocyte adhesion and extravasation, cell activation |
ICAM-3 | Neutrophils, monocytes, lymphocytes | LFA-1 | Leukocyte adhesion, cell activation |
VCAM-1 | Endothelial cells | VLA-4 | Leukocyte adhesion and extravasation, cell activation |
PECAM-1 | Endothelial cells, neutrophils, platelets, lymphocytes | PECAM-1 | Leukocyte extravasation |
MadCAM | Endothelial cells | L-selectin, α 4 β 7 | Leukocyte homing and adhesion |
JAM | Endothelial and epithelial cells, neutrophils, monocytes, platelets | JAM, LFA-1, Mac-1, VLA-4 | Cell-cell adhesion, regulation of polarity, cell motility |
LFA-1 | Neutrophils, monocytes, lymphocytes, most other leukocytes | ICAM-1,2,3, JAM | Leukocyte adhesion and extravasation, cell activation, cell migration |
Mac-1 | Neutrophils, monocytes, platelets | ICAM-1, JAM, Factor X, iC3b, fibrinogen | Leukocyte adhesion and extravasation, cell activation |
VLA-1 | Neutrophils, lymphocytes, most other leukocytes | Collagen, laminin | Cell-matrix adhesion |
VLA-2 | Neutrophils, lymphocytes, most other leukocytes | Collagen, laminin | Cell-matrix adhesion |
VLA-3 | Neutrophils, lymphocytes, most other leukocytes | Fibronectin, collagen, laminin | Cell-matrix adhesion |
VLA-4 | Neutrophils, lymphocytes, most other leukocytes | VCAM-1, fibronectin | Leukocyte rolling, adhesion, extravasation, cell-matrix adhesion |
VLA-5 | Neutrophils, lymphocytes, most other leukocytes | Fibronectin | Cell-matrix adhesion |
E-cadherin | Epithelial cells | E-cadherin, α E β 7 | Leukocyte adhesion |
GPIb-IX-V | Platelets | vWF, Mac-1, thrombin, P-selectin | Platelet adhesion, thrombosis |
GPIIa-IIIb | Platelets | vWF, fibrinogen | Platelet adhesion, thrombosis |
vWF | Platelets | GPIIa-IIIb, collagen, P-selectin, GPIb | Platelet adhesion, thrombosis |
A multistep model of leukocyte binding to vascular endothelium ( Fig. 65.1 ) has been universally adopted to explain the sequence and time course of involvement of different adhesion molecules in the recruitment of leukocytes to inflamed tissue. The original model for leukocyte adhesion was composed of three major steps, each one of which is mediated by a specific class of proteins. The initial event is leukocyte rolling , whereby weak adhesive interactions result in rolling along the endothelial lining of blood vessels. This may occur within minutes of an inflammatory challenge and is largely mediated by selectins and their glycoprotein ligands. The second step is the exposure of leukocytes to low concentrations of chemoattractants/inflammatory mediators (mostly chemokines), bound to endothelial glycosaminoglycans, which results in leukocyte activation , rapidly leading to increased expression and/or activation of integrins on rolling leukocytes. The final event is integrin-mediated leukocyte arrest . In this phase, leukocyte integrins (e.g., CD11/CD18) bind to counter-receptors that are expressed on the surface of endothelial cells (e.g., VCAM-1), thereby allowing leukocytes to become firmly attached to the endothelium and remain stationary. The subsequent transendothelial migration of leukocytes into the interstitial compartment involves the participation of additional adhesion glycoproteins such as PECAM-1, MAdCAM-1, or JAM-A. In recent years, this three-step model has been proven oversimplistic, and new data have led to an expanded version of the leukocyte adhesion cascade, which now includes slow rolling, adhesion strengthening, intraluminal crawling, paracellular and transcellular migration, and migration through the basement membrane. In all, the actual sequence of movement from blood circulation to the extravascular space encompasses several major steps, that is, signaling and homing, tethering and rolling, arrest and adhesion, and transmigration.

65.2.1
Signaling and Homing
Local immune cells provide the appropriate signals to attract circulating leukocytes toward peripheral tissues, primarily through the secretion of chemokines. These are low-molecular-weight proteins, which are classified into four distinct families based on their aminoacid sequence (CC, CXC, C, and CX3C), that interact with specific chemokine G-protein-coupled receptors expressed on endothelial cells. Local concentrations of chemokines within the thymus maintain continuous circulation of lymphocytes from this maturation site to peripheral lymphoid sites. Chemokines also participate in migratory specialization via their selective tissue localization. In line with this paradigm, CCL25 is predominantly expressed in the small intestine and thymus; thus, it is believed to play an essential role in gut-specific lymphocyte trafficking. When inflammation occurs, the expression of additional “inflammatory” chemokines (CCL2, CCL3 and CCL5, CXCL1, CXCL2, and CXCL8) increases, further potentiating migration of leukocytes to the sites of inflammation.
65.2.2
Tethering and Rolling
This initial step is defined by the formation of the first molecular bonds between a circulating leukocyte and the vascular endothelium that have come in close proximity. Three types of selectins mediate this process (P, E, and L-selectins) by binding to their target ligands ( Fig. 65.2 ). Additionally, L-selectins can also bind directly to the mucosal addressin cell adhesion molecule (MAdCAM)-1 integrin. Under inflammatory conditions, other mechanisms may also participate, such as secondary capture that is achieved via leukocyte-leukocyte interaction. These mechanisms are also cell specific. In particular, neutrophils start rolling as they exit capillaries. On the other hand, naive lymphocytes capture in high endothelial venules (HEVs) of peripheral lymph nodes (PLNs) and other lymphoid tissues. Finally, little is known about the capture of effector/memory lymphocytes, although selectins and CD44 may be involved. Transient bonds form and break as a result of these molecular interactions that cause white cells to stumble along endothelial cell surfaces. As the process of “rolling” of leukocytes begins, endothelial cell attachment is accomplished by the tetrasaccharides sialyl Lewis X and A receptors. In all, this transient tethering of leukocytes is the first critical step in their migration from the vasculature to inflammatory areas. A stable rolling movement follows leukocyte capture if new molecular bonds are formed before the initial molecular bonds dissociate. In this step the leukocyte is in constant contact to the vessel wall and its movement is flow driven. Again, there are cell-specific mechanisms. Naive lymphocytes utilize L-selectin, which binds to sulfated carbohydrate-containing ligands on the surface of endothelial cells. Effector T cells can arrest through the chemokine receptor CXCR3 in vitro, but the molecules involved for in vivo arrest remain unknown. Neutrophils arrest by gradually slowing down, whereas naive lymphocytes arrest immediately upon encountering the appropriate chemokine gradients.

The aforementioned interactions between selectins and their ligands facilitate adherence of leukocytes to inflamed endothelia in the presence of blood flow. In fact, L- and P-selectins require shear stress for adhesion, which strengthens the molecular bond created by selectins; hence, rolling cells are detached when flow ceases. Rolling has at least two distinct consequences for the cell: first, it facilitates stable leukocyte arrest (firm adhesion); and second, it reduces leukocyte velocity drastically (to between 1 and 100 μm/s), thus increasing the duration of exposure to the endothelial surface and to chemokines and other activating signals that are present in the local environment. This facilitates the next step of migration, during which leukocytes overcome the shear forces of the bloodstream, arrest and firmly adhere to the endothelium.
65.2.3
Arrest and Adhesion
This step requires activation of the rolling lymphocyte, which is accomplished via binding of a chemokine to a heptahelical transmembrane chemokine receptor on the leukocyte surface. In flow chamber systems, this process is exceptionally rapid. Under inflammatory conditions, there are several sources for tissue chemokines. For example, endothelial cells synthesize chemokines under the influence of proinflammatory cytokines or transport chemoattractants from their abluminal surface. Additionally, mast cells and platelets also generate chemoattractants and deliver them to endothelial cells.
Firm adhesion requires binding of integrin receptors in their active conformation to their endothelial ligands. Integrins are heterodimeric proteins on cell surfaces that consist of two transmembrane glycoprotein subunits, α and β. During homeostasis, integrins are maintained in a nonactive form; nevertheless, in the presence of inflammatory mediators, integrins transition to their activated state and become capable of engaging their respective ligands (CAMs) that are present on endothelial surfaces. For example, very late antigen (VLA)-1 and lymphocyte function associated antigen (LFA)-1(CD11a/CD18) bind to intercellular cell adhesion molecule (ICAM)-1 (CD54) and ICAM-2, which are expressed on endothelial cells. Alternative pathways include the α 4 β 1 (VLA-4) and α 4 β 7 integrins, which mediate firm adhesion to their respective ligands vascular cell adhesion molecule (VCAM)-1 (CD106) and mucosal addressin cell adhesion molecule (MAdCAM)-1. Arrest enables leukocytes to firmly anchor to the mucosa. This is followed by postadhesion strengthening, which initiates the process of transmigration across the mucosal barrier, leading to the final entrance to the inflamed target tissue.
65.2.4
Transmigration
Firm attachment to the endothelium allows leukocytes to finally migrate from the intravascular space into the interstitium. This is also a complex process that develops through various steps, which may occur either sequentially or separately. Before actually crossing the walls of postcapillary venules, neutrophils and monocytes crawl inside blood vessels. This step is mediated by ICAM-1 and macrophage receptor-1 (MAC-1). Crawling helps cells to identify the best sites to transmigrate and also affects the way of exit. In fact, when crawling is disabled, transmigration is delayed and occurs preferentially through the transcellular, as opposed to the paracellular route. The paracellular migration pathway involves several adhesion molecules, mostly endothelial-expressed vascular endothelial cadherin (VE-cadherin), platelet/endothelial-cell adhesion molecule 1 (PECAM1), and junctional adhesion molecule A (JAM-A). In addition, endothelial cell-selective adhesion molecule (ESAM), ICAM2, and CD99 may also play a role in the paracellular migration pathway. Several of these molecules are involved in the transcellular route of migration. This type of migration includes the extension of membrane protrusions into endothelial cells, triggering of cytoplasmic signaling, translocation of apical ICAM1 to caveolae and F-actin-rich regions, transport to the basal plasma membrane, and the final formation of channels through which leukocytes can migrate. The final obstacles to leukocyte exit from the blood and into the interstitium are the endothelial basement membrane and pericyte sheath. This is probably accomplished through regions of low expression of matrix proteins.
These processes are affected by the function of multiple proinflammatory cytokines (TNF-α, IL-1, IL-3, IL-5), chemokines (IL-8 and MCP-1), and products of lipid metabolism (leukotriene β4 and lipopolysaccharide (LPS). These soluble mediators promote leukocyte adhesion and transmigration via various mechanisms. Certain factors induce the expression of ligands on endothelial cells, whereas others trigger integrin activation, the end product being an amplified capacity for cell-cell, ligand-receptor interaction.
65.2.5
Egress
Lymphocyte migration toward specific tissues and their draining lymph nodes is organized by the function of chemokines, as it is presented above. In contrast, the exodus of T cells from the lymph nodes back to the circulation (egress) is regulated by the natural bioactive lipid sphingosine-1-phosphate (S1P), which is formed upon phosphorylation of membrane-derived sphingosine by sphingosine kinase-1. There are several receptors for S1P (Sphingosine-1-Phosphate Receptors, S1P1-5), which are expressed in a cell-type specific manner within different tissues and play integral roles in lymphocyte trafficking. In particular, S1P1 is expressed on lymphocytes, in the vascular endothelium, brain, and lymph nodes. Lymphocytes migrate from the lymph node into blood following an S1P gradient that is maintained by the high levels of S1P in the blood and lymph, which far exceed those at tissues. Tissue levels of S1P are tightly controlled due to intracellular degradation by S1P lyase or dephosphorylation by S1P phosphatases. Pharmaceutical intervention via S1P receptor agonists induce internalization and degradation of S1P receptors, rendering the cells unable to follow the S1P gradient to migrate from tissues back to blood. This process that results in loss of lymphocyte receptors eventually leads to cell trapping in lymph nodes. Selective agonism of S1P1 is believed to regulate immune cell trafficking in tissues including the gut.
65.3
The Molecular Components of Intercellular Adhesion Cascade
65.3.1
Selectins
The selectin proteins were first discovered through the use of monoclonal antibodies that inhibited leukocyte homing (L-selectin) and leukocyte binding to endothelial cells (E-selectin). P-selectin was also found as a membrane protein within platelet granules with an unknown function, and also later identified on endothelial cells. Selectins are critically important for leukocyte-endothelial cell rolling interactions, particularly during inflammatory states. Selectin proteins consist of a short cytoplasmic domain, a transmembrane domain, a series of short consensus repeat domains (SCR domains), a single EGF like domain, and a Ca ++ dependent lectin binding domain at the amino terminus ( Fig. 65.2 ). The cytoplasmic domain can be phosphorylated on tyrosine, threonine, and serine residues; however, the importance of phosphorylation is unknown. It is believed that the SCR domains may be involved in maintaining protein structure and can possibly enhance ligand binding. Similarly, the EGF-like domain is quite homologous among all selectins and has been reported to be involved in cell adhesion. The most critical domain for selectin function is the Ca ++ -dependent lectin domain. X-ray crystallography studies have shown that Ca ++ binding induces a conformational change within this domain, which facilitates ligand binding.
The cellular distribution of selectins determines their physiological and pathophysiological roles. P-selectin is expressed by both platelets and endothelial cells, whereas E-selectin expression is only observed on endothelium. Both E- and P-selectins facilitate leukocyte rolling on the endothelial cell surface, the initial step in leukocyte recruitment from the vascular lumen to the extravascular tissue. P-selectin is also utilized for platelet adhesion. P-selectin is contained in preformed pools within either α-granules of platelets or Weibel-Palade bodies of endothelial cells. Cell activation in response to inflammatory signals leads to rapid mobilization of P-selectin to the surface of platelets or endothelial cells within a matter of minutes. In addition, the expression of both E- and P-selectins is transcriptionally induced by several inflammatory cytokines such as TNF-α, IL-1β, or bacterial LPS. Interestingly, platelet P-selectin does not affect leukocyte recruitment, whereas endothelial P-selectin is important for either leukocyte or platelet rolling on stimulated endothelium.
The role of selectins during an inflammatory response can be varied depending upon the type of inflammatory stimuli, the time course of inflammation (acute vs chronic), and the particular organ that is studied. Nonetheless, the overall interpretation of multiple studies suggests that P-selectin is important for immediate leukocyte rolling in response to inflammatory stimuli and trauma, and that the role of E-selectin may be important during longer periods of inflammation (> 4 h) and may also influence leukocyte firm adhesion. Initial reports of P-selectin knockout mice demonstrated decreased early leukocyte rolling in response to tissue injury and/or inflammation, increased leukocyte rolling velocities during longer periods of inflammation (> 1 h), and decreased leukocyte recruitment into the peritoneum over a 4-h period. It is interesting that the cytoplasmic domain of P-selectin is important for proper targeting to endothelial cell Weibel-Palade bodies in vivo and that mice containing P-selectin cytoplasmic domain deletions demonstrate many of the phenotypic features of P-selectin knockout mice.
Consistent with these observations, loss of P-selectin has been reported to be protective in several inflammatory settings, such as ischemia/reperfusion injury, peritonitis, sepsis, allergic inflammation, contact hypersensitivity, and atherosclerosis. Nevertheless, this is not a uniform association, as leukocyte adhesion in certain organ vascular beds or during specific disease conditions may be selectin independent. For example, in lung, brain, and liver inflammatory settings either P or E-selectin have been shown to be unnecessary or minimally involved in leukocyte recruitment. However, these findings may be stimulus- or injury specific, since an in vitro study using P-selectin mutant brain endothelial cells reported TNF-α and IL-1β-mediated neutrophil adhesion to be significantly influenced by P-selectin expression.
While the importance of P-selectin in inflammation has been well identified, the exact role of E-selectin has remained somewhat elusive. This may be due to different patterns of expression and modes of regulation. As mentioned earlier, P-selectin is synthesized and stored in Weibel-Palade bodies, which can be mobilized in minutes after an inflammatory response. In contrast, preformed pools of E-selectin do not exist and induction of this molecule requires transcriptional activation. However, these same signaling mechanisms also induce transcription of the P-selectin gene. Therefore, an increase in both selectin molecules can be observed simultaneously during a given inflammatory response. Therefore considerable functional overlap between the two molecules can be presumed. Detailed examination of E-selectin mutant mice has shown that this molecule is important for leukocyte slow rolling and mediates increased leukocyte transit times, thereby allowing adequate leukocyte sampling of the endothelial cell surface and facilitating interaction between these cell types. This notion is further supported by the observation that loss of E-selectin results in decreased firm adhesion of leukocytes and may be necessary for proper integration of signals involved in leukocyte arrest.
L-selectin expression is observed on most leukocytes including lymphocytes, granulocytes, and monocytes. L-selectin has been shown to be important mediator of lymphocyte homing to PLNs and Peyer’s patches in response to inflammatory stimuli. Engagement of ligands, such as PNAd or MAdCAM, stimulates L-selectin mediated outside-in signaling that can enhance additional adhesion interactions such as β 2 integrin/ligand association. Importantly, L-selectin is proteolytically cleaved from the leukocyte surface following activation, which has become a useful indicator in specific immune states.
65.3.2
Immunoglobulin Superfamily
The immunoglobulin superfamily proteins share a similar structural motif, in that they all contain Ig-like extracellular domains that are responsible for cell adhesion. Members of this family are intercellular adhesion molecule-1, 2, and 3 (ICAM-1-3), vascular cell adhesion molecule (VCAM), platelet endothelial cell adhesion molecule (PECAM), mucosal adressin cell adhesion molecule (MAdCAM), and junctional adhesion molecule (JAM) ( Fig. 65.3 ). These molecules are important for normal immune surveillance, leukocyte adhesion, and leukocyte emigration.

ICAM-1 was originally discovered through studies using blocking monoclonal antibodies against the integrin LFA-1 (CD11a/CD18). ICAM-1 also binds to integrins Mac-1 (CD11b/CD18) and p150/95 (CD11c/CD18). ICAM-1 is constitutively expressed at low levels and is transcriptionally induced by several inflammatory mediators. ICAM-1-ligand interactions are involved in leukocyte firm adhesion and emigration through engagement with the endothelium and are also involved in leukocyte-leukocyte aggregation and activation. These cellular events are facilitated by ICAM-1 mediated outside-in signaling through integrin binding. Cross-linking of ICAM-1 has been shown to stimulate many endothelial cell signal transduction cascades, such as MAP kinase, src kinase, and NOX4 pathways. Recent work has also revealed that ICAM-1-signaling-dependent responses regulate endothelial cell redox status by increasing intracellular glutathione levels and that this controls cellular activation and inflammatory responses.
Inhibition or genetic deficiency of ICAM-1 protects mice against ischemia-reperfusion injury in several organs, including the heart, kidney, and brain. ICAM-1 is also important during the progression of chronic inflammatory diseases, inasmuch its genetic loss ameliorates experimentally induced colitis, SLE-associated vasculitis and glomerulonephritis, and reduces susceptibility to collagen induced arthritis.
Given the diverse functions of ICAM-1 during immune responses, its precise role in mediating leukocyte-endothelial interactions has been examined. Interestingly, loss of ICAM-1 specifically influences the adhesion of certain leukocyte types in a stimulus-dependent manner. For example, in the absence of ICAM-1 signaling eosinophil adhesion in mesenteric venules in response to ragweed allergen challenge is decreased. However, TNF-α stimulation of neutrophil adhesion in ICAM-1 mutant mice has revealed that this protein is either not required for adhesion or is redundant with other adhesion molecules. Thus, ICAM-1 appears to be required for chemoattractant-mediated adhesion in resting venules, but not in TNF-α stimulated venules. In vitro studies with endothelial cells from ICAM-1-deficient mice showed that it is important for monocyte firm adhesion and T-cell emigration across endothelial monolayers. In addition, ICAM-1 was shown to be necessary for optimal selectin-mediated rolling, as leukocyte rolling velocities on TNF-α-stimulated vessels and endothelium were faster compared to wild-type controls. A previously unappreciated step of the leukocyte recruitment cascade involving intraluminal crawling after firm adhesion but before transmigration has been reported. ICAM-1 interaction with Mac-1 counter ligand appears to govern intraluminal neutrophil crawling on the endothelial cell surface perpendicular to the direction of blood flow thus allowing the leukocyte to locate cell-cell junction regions for transmigration. These findings highlight a complex role for ICAM-1 in regulating endothelial responses leukocyte-endothelial interactions during inflammation.
ICAM-2 was primarily described on endothelial cells. The structures of ICAM-1 and 2 are similar in that they consist of a cytoplasmic domain, a transmembrane domain, and Ig-like regions. However, they differ in that ICAM-1 contains 5 Ig-like domains compared to only 2 for ICAM-2. ICAM-2 is constitutively expressed on the cell surface and, unlike ICAM-1, it is not inducible by inflammatory mediators. This suggests a role for ICAM-2 in normal immune surveillance. Gene-targeted loss of ICAM-2 does not alter lymphocyte homing or development of leukocytes but it reduces eosinophil transmigration across endothelial monolayers and prolongs allergic eosinophil accumulation in certain organs.
ICAM-3 is expressed on all resting leukocytes and, similar to ICAM-1, contains five Ig domains. ICAM-3 is important for leukocyte aggregation and activation through its interaction with the counter-ligand LFA-1.
Vascular cell adhesion molecule (VCAM-1) is a cytokine-inducible surface molecule that mediates adhesion of various leukocyte types, such as lymphocytes, monocytes, and eosinophils. VCAM-1 was identified through direct expression techniques using a cell-cell adhesion-based screening procedure with cytokine-induced cDNA genes. Candidate genes were transfected into epithelial cells and examined for adhesion to lymphocytic cell lines. Since its original characterization, VCAM-1 has been shown to participate in both acute and chronic inflammatory responses, being primarily expressed by endothelial cells. VCAM-1 binds α 4 β 1 (VLA-4) and α 4 β 7 integrin and participates in leukocyte firm adhesion and migration. VCAM-1 has also recently been reported to bind the α 9 β 1 integrin of neutrophils and facilitate neutrophil migration across endothelial cells.
Platelet endothelial cell adhesion molecule (PECAM-1) was originally identified through the serological characterization of surface proteins of vascular endothelium and contains six Ig-like domains, a transmembrane domain, and a cytoplasmic tail that can be differentially phosphorylated and palmitoylated. Modification of the cytoplasmic tail facilitates outside-in signaling, which can influence endothelial cell function (e.g., angiogenesis or cell survival) and leukocyte recruitment. PECAM-1 preferentially localizes at intercellular junctions of endothelial cells, suggesting a role in leukocyte emigration. Consistent with this hypothesis, blocking antibodies against endothelial PECAM-1 prevents leukocyte emigration across endothelial monolayers. PECAM-1 expression was also shown on several other cell types, including platelets, monocytes, neutrophils, natural killer cells, and certain T-cell subsets, which may facilitate adhesion and transmigration through homotypic binding of endothelial PECAM-1.
MAdCAM-1 was identified through monoclonal antibody screening studies that showed discrete staining of HEVs of mucosal lymph nodes (Peyer’s patches). Additional studies of MAdCAM-1 expression identified constitutive expression in tissues involved in mucosal immunity as well as inducible expression by proinflammatory mediators (e.g., TNF-α). As suggested by its tissue distribution, MAdCAM-1 mediates lymphocyte homing by directing adhesion and emigration across HEVs of mucosal tissue. MAdCAM-1 contains three extracellular Ig-like domains with a mucin-like region between domains 2 and 3. Primary ligands for MAdCAM-1 include L-selectin and α 4 β 7 integrin, which bind to Ig domain 1. Importantly, several reports have documented an important role for MAdCAM-1 in mediating chronic inflammatory states involving gastrointestinal (GI) tissues such as ulcerative colitis, Crohn’s disease, and experimental colitis models. Ongoing trials are assessing the efficacy of its blockade in UC, while Phase 2 studies in CD did not show efficacy.
The most recently reported immunoglobulin adhesion molecule is JAM. Three different JAMs (JAM-A, B, and C) have been described. JAM expression is observed on endothelial and epithelial cells, leukocytes, and platelets and consists of two extracellular Ig-like domains and a cytoplasmic tail that differentially associates with multiple signaling and cytoskeletal-associated proteins. The original identification of JAM-A revealed junctional localization in endothelial cells that participated in monocyte transmigration. Studies demonstrated that JAM proteins localize to tight junctions in both epithelial and endothelial cells and that members of the JAM subfamily bind either homotypic (i.e., other JAMs) heterotypic ligands. The latter include integrins LFA-1 (α L β 2 ), Mac-1 (α M β 2 ), VLA-4 (α 4 β 1 ), and α V β 3, emphasizing the importance of these molecules in regulating leukocyte recruitment. Studies have shown that JAM-integrin interactions control leukocyte infiltration during inflammation. However, JAM may also regulate intestinal permeability and inflammatory responses through homotypic interactions. Recent work by Laukoetter et al. revealed complex roles of JAM-A in controlling intestinal inflammation. Interestingly, genetic deficiency of JAM-A did not alter GI epithelial architecture but did increase leukocyte infiltration and lymphoid aggregates concomitant with increased mucosal permeability. Surprisingly, these animals showed attenuated DSS induced colitis and increased epithelial cell proliferation. Together, these results imply that adhesion molecules often influence additional GI functions besides leukocyte recruitment and inflammation.
65.3.3
Integrins
The integrin family of proteins consists of several adhesion receptors, which are obligatory type I αβ-heterodimers. In particular, integrin molecules are formed through various combinations of 17 α and 8 β subunit genes ( Fig. 65.4 ). The α and β subunits are encoded by separate genes that do not necessarily show coordinated transcriptional regulation. As such, several α protein chains interact noncovalently with multiple β protein chains yielding a diverse array of heterodimer pairing. Integrins bind to ligands in the extracellular matrix and counter-receptors on other cells, thus, mediating cell-cell or cell-matrix adhesion. Several integrin ligands have been described and largely include members of the immunoglobulin superfamily and extracellular matrix proteins. Integrins have important roles in regulating intracellular signal transduction and cytoskeletal organization of all cell types, participating in almost every step of cell biology.

Integrins undergo large conformational changes in their extracellular domains in response to intracellular signaling events, a process that is often referred to as inside-out signaling. This process is initiated by adaptor molecules, which affect the positions of the cytoplasmic tails of α- and β-subunits in such a way that exposes the ligand-binding domain to adhesive ligands. The best-known adaptor molecules that initiate integrin activation are talin 1 and kindlins 1–3. In addition to their role in adhesion, integrins are also involved in signal transduction. Upon integrin activation, which follows binding of extracellular ligands a process that is called outside-in signaling develops. This involves ligand-dependent integrin clustering, which leads to proximity of the signaling domains of integrin-proximal proteins and setting off intracellular cascades. The latter include activation of various kinases in platelets and leukocytes and activation of NADPH oxidase in leukocytes.
The β 2 integrins consist of a common beta subunit (CD18) noncovalently attached to different alpha subunits (CD11a-d). The β 2 integrin family, also referred as CD18 integrins, consists of four different adhesion proteins LFA-1 (CD11a/CD18, targeted by efalizumab/Raptiva), Mac-1 (CD11b/CD18), p150/95 (CD11c/CD18), and CD11d/CD18. Importantly, expression of the β 2 integrins is limited to leukocytes and platelets, which suggests their importance for immune responses. Members of the immunoglobulin superfamily, including ICAM-1, 2, and 3, and proteins of the JAM family, are major ligands for β 2 integrins. Integrin-mediated cell adhesion is controlled through multiple mechanisms, including alterations in surface density and changes in receptor affinity. The important role for β 2 integrin/ligand interactions in immune processes is illustrated by the attenuated inflammatory responses in several experimental models of disease in mice bearing mutations of CD18. Data from numerous studies indicate that β 2 integrins are critical mediators of leukocyte adhesion and emigration, costimulation and activation, leukocyte-mediated tissue damage, and serve pathophysiological roles during autoimmune disease. However, it is important to bear in mind that complete inhibition of all β 2 integrins (via genetic deletion or immunoneutralization of CD18) is considerably different than selective inhibition of a single member of the family (i.e., inhibition of CD11a, CD11b, CD11c, or CD11d). Along that line, genetic deletion of CD11a confers protection against inflammation and immunological disorders similarly to CD18, whereas the loss of CD11b may exacerbate inflammatory responses and tissue injury.
The α 4 integrins, α 4 β 1 (VLA-4) and α 4 β 7 , are primarily expressed by lymphocytes, yet may also be found in granulocytes and monocytes. They are unique in that they can participate in all steps of leukocyte recruitment, such as tethering, rolling, and firm adhesion. VCAM-1 ligand binding occurs with both molecules while MAdCAM is the primary ligand for α 4 β7. Through these interactions, α 4 β 7 facilitates lymphocyte homing to the mesenteric lymph nodes and Peyer’s patches while VLA-4 plays a role in facilitating immune cell recruitment in the blood circulation. VLA-4 may also play an important role in autoimmune responses since antibody blockade of the molecule protects in several autoimmune disease models.
65.3.4
Cadherins
Adhesion molecules of the cadherin family are typically identified as surface proteins located at the adherens junction or desmosome with specific calcium requirements for homotypic interaction. Cadherin proteins contain three general structural regions: a cytoplasmic domain, a transmembrane domain, and extracellular cadherin repeat domains that are structurally similar to Ig domains. The amino terminal end of the extracellular region contains the HAV domain that is primarily involved in homotypic binding. Cadherin molecules provide structural support to adherens junctions and desmosomes through binding catenins that link to the actin cytoskeleton. Moreover, cadherins also influence signal pathways and gene expression through differential association with various catenins (e.g., β- or γ-catenin). Numerous cadherins have now been identified, which are divided into classical and nonclassical groups. A primary function of nearly all cadherins is the regulation of homotypic adhesion of several cell types such as epithelial, endothelial, neuronal, and others. Homotypic adhesion typically involves one predominant cadherin molecule; however, different cadherin molecules may also be expressed by the same cell. For example, lateral epithelial adherens junction is maintained by E-cadherin homotypic interactions, yet both N- and P-cadherin may also be expressed. Recent reports have also shown that cadherins may bind other adhesion molecules in a heterotypic manner. T-lymphocyte adhesion to epithelial cells has been reported to occur through interaction between T-cell α E β 7 integrin and epithelial E-cadherin. The pathological importance of this heterotypic interaction may be minimal, since genetic loss of β 7 integrin does not prevent spontaneous colitis in IL-2-deficient mice and only delays the development of experimental colitis in the CD4 + CD45RB high transfer model.
Endothelial cell-specific vascular endothelial cadherin (VE-cadherin/cadherin-5) plays important roles in controlling endothelial cell growth, solute barrier properties, as well as leukocyte transmigration. Numerous reports have shown that various cytokines, chemokines, and biochemical mediators (e.g., reactive oxygen species, bradykinin, histamine, etc.) stimulate VE-cadherin phosphorylation, dissociation from catenins, and redistribution away from lateral cell-cell junctions resulting in increased endothelial and microvascular permeability. Recent findings suggest that leukocyte β 2 integrin binding to counter-ligands such as ICAM-1 may activate signaling pathways that lead to transient and discrete disorganization of VE-cadherin homotypic junctional binding between endothelium to allow for leukocyte transmigration.
65.3.5
Platelet Glycoproteins
Platelet adhesion to endothelium or subendothelial matrix may be of importance in immune-mediated diseases that involve the microvasculature of various tissues, including the GI tract. Platelets contain several unique adhesion molecules that facilitate interaction with endothelium and other platelets. The GPIb-IX-V complex of glycoproteins mediates platelet adhesion through binding of the counter-ligands P-selectin, Von Willebrand factor (vWF), and Mac-1. GPIb-IX-V-dependent adhesion is important for platelet adherence under high-shear stress to matrix vWF (e.g., denudation of arterial endothelium), bacterial endotoxin-mediated adhesion to the microvasculature, and platelet recruitment to mesenteric venules activated with histamine. Likewise, platelet GPIIa-IIIb (also classified as integrin α IIb β 3 ) also mediates platelet adhesion through binding to other GPIIa-IIIb molecules, ICAM-1 via fibrinogen bridging, Mac-1, and α v β 3 integrin. GPIIa-IIIb is important for platelet adhesion to venular endothelial cells experiencing low-shear stress and binds to fibrinogen linked to ICAM-1 in venules of postischemic intestine and liver. Both GPIb-IX-V and GPIIa-IIIb glycoproteins participate in intracellular processes with GPIb-IX-V demonstrating outside-in and inside-out signaling, while GPIIa-IIIb undergoes inside-out activation. The glycoprotein vWF is released from platelet α-granules as well as endothelial cell Weibel-Palade bodies that facilitate platelet-platelet binding and adhesion to the vessel wall. These adhesive interactions can be further aided by platelet P-selectin and PSGL-1 (P-selectin glycoprotein ligand-1) interaction among platelets themselves or with endothelium.
65.3.6
Occludin and Claudins
Endothelial and epithelial tight junctions represent a final barrier that leukocytes must cross to access interstitial spaces. Tight junctions demonstrate both a “gate” function (to regulate cell passage) and a “barrier” role (to maintain endothelial and epithelial permeability). Dysregulation of either function may affect leukocyte recruitment and inflammatory responses. Occludin was the first integral tight junction protein identified through membrane fraction and immunolabeling techniques. Occludin is a 65 kDa integral membrane protein that contains four transmembrane domains with two hydrophobic extracellular loops with cytosolic amino and carboxy terminal ends. The second extracellular loop mediates homotypic interaction with itself as well as JAM and claudin proteins. The carboxy terminus of occludin binds with ZO proteins (ZO-1 and ZO-2), which link it to actin cytoskeleton. Importantly, junctional organization of occludin restricts leukocyte transmigration across both endothelial and epithelial cells, and is actively reorganized away from lateral cell-cell junctions by several agents (e.g., leukocytes, oxidants, cytokines, chemokines), thereby increasing solute permeability. Genetic deficiency of occludin does not alter morphological properties of tight junctions or differences in epithelial barrier properties but results in chronic inflammation and hyperplasia of GI epithelium. Interestingly, studies have shown that anti-CD3 stimulation of cultured T cells and intraepithelial lymphocytes upregulate occludin expression in vitro and in vivo. These findings demonstrate that occludin expression plays a role in regulating leukocyte recruitment and GI mucosal immunity, which remains poorly understood.
The claudin proteins are the largest protein family found within tight junctions and primarily regulate permeability selectivity of endothelial and epithelial cells. The claudin protein family consists of 24 different members with molecular weights ranging between 20 and 27 kDa. The structure of claudin proteins is similar to occludin in that they are tetra-span integral membrane proteins with two extracellular loops; yet they do not share sequence homology with occludin. The carboxy terminal tail of claudin proteins also bind ZO proteins that link them to actin cytoskeleton. Claudin proteins bind each other in a homo- and heterotypic manner, as well as in cis or trans interaction formats. These binding properties form the basis for the characteristic tight junction protein strands, as seen by freeze-fracture electron microscopy. They also provide a wide array of interactive binding conformations that confer unique barrier functions of epithelial and endothelial cells from different tissues. Claudin 1 and 5 play important roles in regulating endothelial cell barrier function and have been suggested to be involved in modulating leukocyte recruitment. However, much less is known regarding the importance of claudin proteins in regulating leukocyte recruitment across endothelial and epithelial cells.
65.3.7
Chemokines and Receptors
In addition to the adhesion molecules that were presented above, trafficking of leukocytes to peripheral tissues is critically regulated by a large family of 50 chemokines and their 20 receptors. Chemokines are classified into four distinct groups according to the pattern of the first two of four cysteine residues: CC family (the first two cysteine residues adjacent to each other), CXC family (a single amino acid residue between the first two cysteines), CX3C family (three amino acid residues between the first two cysteines), and the XC family (absence of two cysteine residues). Several chemokines are constitutively expressed, being mostly involved in the regulation of homeostatic leukocyte trafficking. Others, however, are mainly upregulated during inflammatory states and mediate recruitment of leukocytes to areas of inflammation, hence their classification as inflammatory chemokines. Most chemokines are secreted proteins that are produced by activated endothelial or mesenchymal cells and bind to matrix glycosaminoglycans. As the latter are anchored to cellular or matrix proteins, chemokines become immobilized in the tissues through their attachment to glycosaminoglycans.
Chemokines bind to heptahelical receptors present on the surface of leukocytes. Chemokine receptors are seven-transmembrane-spanning proteins coupled to heterotrimeric G protein (so-called G-protein-coupled receptors). When chemokines attach to their corresponding receptors an intracellular signal is transduced, which leads to rapid increase in integrin binding avidity. This, in turn, facilitates firm adhesion of leukocytes to endothelial cells and, eventually, transmigration into the tissue.
The orchestrated movement of leukocytes to peripheral tissues is mediated by various combinations of ligand/receptor pairs, which are dependent upon the particular cell type, the specific tissue, and the inflammatory status (healthy vs inflamed). Neutrophils and monocytes are cells of innate immunity that infiltrate acutely inflamed tissues. Their movement into inflamed areas are mediated by chemokines of the CXC family and the CC subfamily of monocyte chemoattractant proteins. Proinflammatory cytokines provide strong stimulatory signals for the upregulation of CXC chemokines and subsequent recruitment of innate cells. Trafficking of lymphocytes is dependent upon the expression of various chemokine receptors, which vary along their maturation process from naïve to primed cells. Entry of naïve T cells into secondary lymphoid organs is mediated by interactions between lymphocytic CCR7 and its ligands, CCL19 and CCL21. Dendritic cells are also subjected in a coordinated maturation process, during which expression of constitutively expressed chemokine receptors (CCR1, CCR5, and CCR6) is switched off, whereas expression of CCR7 is turned on, thus allowing entry into the afferent lymphatic vessels and the lymph node (LN). After priming, T-cells downregulate the expression of CCR7 and upregulate other receptors, which may differ between the various subclasses of effector lymphocytes. In particular, CCR5 and CXCR3 predominate on Th1 cells, whereas CCR4 and CCR8 are preferentially found on Th2 cells. The pivotal class-defining cytokines, such as interferon (IFN)-γ and IL-4, generate self-amplifying loops of chemokine expression. Memory T cells are further differentiated by the expression of CCR7. CCR7 + memory T cells are termed central memory cells (TCMs) and are capable of migrating into lymphoid tissues, but, also to inflamed peripheral areas, as they simultaneously express inflammatory chemokine receptors. On the other hand, CCR7-memory cells, which are termed effector memory (TEM), do not have LN homing potential due to the lack of CCR7. Thus, CCR7 is pivotal for lymphocytic trafficking inasmuch it allows for both entry of naive cells into the lymph nodes, but also for the recirculation of activated effector and memory T cells back to the areas with inflammation. The specific combination of chemokines and their receptors are also critical for applying tissue-specific address codes to circulating lymphocytes. In that perspective, several homing molecules implicated in intestinal immunity have been described. Among those, the chemokine CCL25 has been shown to be exclusively expressed in small intestinal tissue and through its interaction with receptor CCR9 regulates trafficking toward the small intestine (see below).
65.4
Regulation of Leukocyte-Endothelial Cell Interactions in Nonlymphoid Tissues
65.4.1
Adhesion Molecules
The dual radiolabeled monoclonal antibody technique has provided novel insights into the magnitude and kinetics of endothelial CAM expression in GI tract and other vascular beds. Members of the immunoglobulin supergene family exhibit high levels of constitutive intestinal expression for ICAM-1, VCAM-1, and MAdCAM-1, with substantial and sustained increases after cytokine stimulation. On the other hand, PECAM-1 and ICAM-2 also show high basal levels of expression; nevertheless, their intestinal expression remains unchanged after stimulation with LPS or TNF-α.
The aforementioned responses for the Ig supergene family proteins contrast with those seen for selectins, which exhibit a much lower level of basal expression with more profound (yet transient) increases after endothelial cell activation. In particular, significant constitutive intestinal expression of P-selectin was demonstrated in wild-type mice. The high constitutive levels of P-selectin and other endothelial CAMs (see Table 65.2 ) in the gut are consistent with the view that this organ is normally in a state of controlled inflammation. Transcription-dependent upregulation of both P- and E-selectins can be induced by cytokines and LPS, with P-selectin expression reaching a maximum between 4 and 8 h and E-selectin between 3 and 5 h. These increments of selectin expression may exceed 10-fold. Histamine also significantly increases the expression of P- but not E-selectin in the GI tract and most vascular beds. Unlike transcription-dependent response, histamine induces a two- to fourfold increase in P-selectin expression on endothelial cells within 5 min, which lasts for as long as 1 h. This rapid upregulation of P-selectin by histamine most likely represents mobilization of a preformed pool Weibel-Palade bodies. This mobilization of preformed P-selectin to the endothelial cell surface can be inhibited by an H 1 – (but not H 2 )-receptor antagonist, indicating that it is mediated by histamine engagement of H 1 receptors.
Organ | Endothelial CAM Expression (Molecules × 10 6 per cm 2 ) | |||
---|---|---|---|---|
P-Selectin | E-Selectin | ICAM-1 | VCAM-1 | |
Small intestine | 254.9 | 87.8 | 171.0 | 32.7 |
Lung | 26.6 | 1.10 | 268.1 | 23.7 |
Heart | 5.7 | 46.5 | 92.5 | 81.8 |
Muscle | 10.2 | 0.0 | 89.0 | 35.0 |
Brain | 4.8 | 1.5 | 9.0 | 42.9 |
Direct visualization of the microvasculature in GI tissues that are either acutely or chronically inflamed has revealed that leukocytes selectively bind to endothelial cells that line postcapillary venules. Leukocyte sequestration in capillaries can occur during GI inflammation; nevertheless, this phenomenon most probably represents steric hindrance of less deformable circulating leukocytes within long narrow capillaries rather than cell-cell interactions via adhesion molecules. An additional mechanism may involve entrapment of leukocytes within capillaries of inflamed tissues due to endothelial cell swelling within capillaries and compression of the capillary lumen by elevated interstitial fluid pressure due to local edema. In any case, it is endothelial cells that line the walls of postcapillary venules that appear to sustain most of the leukocyte trafficking toward inflamed tissues. For example, in rat mesenteric microcirculation, 39% of all leukocytes passing through venules are rolling, in contrast to only 0.6% in the upstream arterioles. The relatively higher shear rates in arterioles and higher endothelial CAM expression in venules are the two most plausible explanations for the differences in leukocyte-endothelial cell adhesion between these two vascular segments. Shear forces generated by the movement of blood within the microvasculature are generally higher in arterioles than in downstream venules. For example, a 30 μm diameter venule in rat mesentery is likely to exhibit a resting shear rate that is half that of an arteriole of comparable diameter. Since wall shear stress, or shear rate, represents antiadhesion forces that oppose the proadhesive forces generated by CAMs, vessels with a low spontaneous shear rate (low blood flow) would tend to exhibit more leukocyte adhesion than vessels with high-shear rates. Based on this hypothesis, one might predict that reductions in arteriolar shear rate to levels obtained at venules should promote leukocyte adhesion in arterioles. However, this is not the case, because when mesenteric arterioles and venules of the same size are exposed to the same range of shear rates (100–1250/s), venules still exhibit far more leukocyte rolling and adherence than arterioles. Studies using retrograde perfusion of the microcirculation have also provided some insight into the role of shear rate in arteriolar-venous differences in leukocyte-endothelial cell adhesion. In the mesentery, retrograde perfusion is associated with a reduced flux of rolling leukocytes in venules and increased leukocyte rolling in arterioles. However, more leukocytes still rolled in venules during normograde perfusion than rolled in arterioles during retrograde flow. These observations indicate that hemodynamic differences between arterioles and venules cannot explain the predilection for leukocyte rolling and adherence in venules, and that a higher density of counter-receptors (ligands) for leukocyte adhesion molecules on venular endothelium is a more likely explanation.
65.4.2
Products of Endothelial Cell Activation
Bacterial toxins as well as a variety of cytokines produced by macrophages and mast cells that normally reside in the GI tract can engage with specific receptors on endothelial cells to elicit an increased expression of CAMs. In particular, LPS, cytokines, and oxidative stress result in the activation of the transcription factors NF-κB and AP-1 in endothelial cells. This is followed by induction of the expression of genes encoding for CAMs. Indeed, binding sites for NF-κB have been identified in the promoter regions of the genes for E-selectin, VCAM-1, MAdCAM-1, and ICAM-1, while AP-1 has a binding site on the promoter region of the gene for ICAM-1.
65.4.3
Auxiliary Cells
Although endothelial cells remain a major candidate for providing the inflammatory mediators that elicit and amplify the leukocyte-endothelial cell adhesion, there is growing evidence that other cell populations contribute as well. Mast cells, macrophages, and platelets are good examples of auxiliary cells that are in proximity to venular endothelial cells and capable of producing a variety of mediators (e.g., PAF, ROS, cytokines), which promote leukocyte adhesion. In particular, in different experimental models of GI inflammation, increased numbers of activated and/or degranulated mast cells have been reported to exist close to postcapillary venules, whereas, further support was provided by studies with agents that degranulate (compound 48/80) or stabilize (ketotifen) mast cells or antagonize mediators that are relatively unique to mast cells (antihistamines). Relatively little information is available concerning the role of macrophages in the regulation of leukocyte-endothelial cell adhesion in the intact GI tract, largely as a result of the limited experimental strategies that are available to selectively manipulate the numbers and activity of these resident phagocytic cells in GI tissues.
A growing number of reports have revealed that the development of a proinflammatory state in the microvasculature is frequently accompanied by a prothrombogenic phenotype; this likely reflects molecular and chemical changes in endothelial cells that create a hyperadhesive surface for both platelets and leukocytes. This link between hemostasis and inflammation appears to have important pathophysiological consequences, with platelets increasing the intensity of the inflammatory response and exacerbating microvascular dysfunction and tissue injury. GI Inflammation that takes place in several experimental models is clearly associated with formation of platelet-leukocyte aggregates, adhesion of platelets directly onto endothelial cells, and attachment of platelets to already adherent leukocytes. There is mounting evidence that adherent leukocytes represent a major substrate for the binding of platelets to the walls of inflamed microvessels ( Fig. 65.5 ). Since platelets have also been shown to play a major role in the recruitment of leukocytes, a codependency of platelet and leukocyte adhesion appear to exist in inflamed venules. Platelet-leukocyte adhesion can be mediated by different ligand-receptor interactions, including P-selectin (platelet)-PSGL-1 (leukocyte) and GPIbα (platelet)-CD11b/CD18 (leukocyte) interactions. Neutrophils may also entrap platelets via the release of neutrophil extracellular traps (NETs), which are largely comprised of DNA. Upon activation, neutrophils can release granular proteins and chromatin that combine to form the structural backbone of NETs. While NETs are thought to play a role in the entrapment and killing of pathogens, there is also evidence suggesting that, in vitro, platelets under flow bind avidly to NETs, thereby promoting thrombosis. Once platelets attach to leukocytes, the capacity of the latter cells to produce superoxide is dramatically increased, suggesting that diffusible molecules liberated by the attached platelets and/or signaling mechanisms that are activated as a result of this heterotypic cell adhesion greatly enhance the activation state of the leukocyte. It is noteworthy that roughly half of the leukocytes that adhere in inflamed intestinal venules are platelet bearing and the other half are platelet free, suggesting that the subpopulation of adherent leukocytes that sustain platelet adhesion may achieve a higher state of activation that favors platelet adhesion. Since neutrophil-derived superoxide has also been implicated in the modulation of platelet adhesion in intestinal venules, an enhanced production of this ROS by the platelet-bearing leukocytes may account for the preferential binding to a subset of adherent leukocytes. A role for ROS is further supported by the observation that the formation of NETs by activated neutrophils also depends on ROS formation by NADPH oxidase.

65.5
Intestinal-Specific Characteristics of Inflammatory Cell Trafficking
65.5.1
Cellular, Molecular, and Structural Organization of Intestinal Immunity
The intestinal mucosa is a unique environment whereby an enormous number of food and bacterial antigens are lined opposite to the largest and most complex component of the immune system and only separated by a single columnar epithelial layer. Avoidance of unnecessary and self-destructive overreactivity is achieved via the development of several elaborate mechanisms by mucosal immune cells, which ensure responsiveness against pathogenic bacteria, parasites, and viruses on the one hand, and harmless dietary proteins and commensal bacteria on the other. Furthermore, the mucosal immune system is able to choose the appropriate effector responses necessary to deal with each pathogen. To deliver such sumptuous functions, the intestinal tract has developed a complex network of distinct but highly interconnected structures, cells and molecules, which work in high synchronization to form the intestinal mucosal barrier. Central to this barrier is the gut-associated lymphoid tissue or GALT, a highly developed immunological organ that is involved in antigen transport, processing and presentation to T cells. The latter takes place in the mesenteric lymph nodes (MLNs), the largest lymph nodes in the body, which serve to collect and concentrate antigens draining from the intestinal mucosa and mount immune responses against them.
To accomplish its multiple tasks, GALT is comprised of inductive and effector sites. Inductive sites are discrete forms of organized lymphoid tissue, which include macroscopically visible Peyer patches (PPs) and microscopic lymphoid aggregates (isolated lymphoid follicles, including cecal patches, colonic patches, and cryptopatches), scattered throughout the lamina propria of the small and large intestine. The PPs are lymphoid aggregates immediately underlying the intestinal epithelium and are found throughout the entire length of the small intestine. These “lymph-node like” structures consist of collections of large numbers of T cells, separated by well-defined B-cell follicles. At the luminal side, Peyer patches are covered by a specialized layer of columnar epithelial cells, the follicle associated epithelium (FAE) ( Fig. 65.6 ). The space immediately underlying the FAE is referred to as the subepithelial dome (SED). FAE can be distinguished from the epithelial cells that cover intestinal villi by its less pronounced brush border, reduced levels of digestive enzymes, and large number of infiltrating T- and B-lymphocytes and antigen-presenting cells (APCs). The most characteristic feature of the FAE is the presence of microfold (M) cells. These cells are specialized enterocytes that do not contain neither surface microvilli nor mucus and play critical roles in binding invasive microorganisms and particulate antigens, as well as transporting luminal antigens to the SED.

There are several ways for antigen sampling, uptake, and transport of intraluminal antigens to the inductive sites of GALT and MLNs. One well-characterized pathway is via uptake by M cells and transfer to the underlying SED region, whereby antigens are endocytosed by professional APCs called dendritic cells (DCs) ( Fig. 65.6 ). DCs then migrate to the B- and T-cell regions of the PPs and prime/activate B cells to produce the antimicrobial IgA. Whether priming of naïve (antigen-inexperienced) T cells may occur within the PPs remains a matter of debate, with most evidence being somewhat vague and ill-defined. At present, most data support the notion that generation of effector T cells takes place in the MLNs, primarily, when antigen-loaded DCs arrive there from the PPs via afferent lymphatics and present captured and processed antigens to naïve T cells.
Luminal antigens may also gain access to the intestinal lamina propria following endocytosis by DCs that reside within the intraepithelial cell compartment of the small bowel. Such DCs are CX3CR1 + and possibly CD11b + TLR5 + and migrate to the basolateral space between epithelial cells where they extend their pseudopodia apically/luminally between epithelial cells to “sample” the luminal environment. Because antigen-loaded CX3CR1 + DCs are unable to migrate to the MLNs, they appear to promote the generation of Th17 locally within the lamina propria of the gut. Finally, enteric antigens may gain access to the intestinal tissue by simple paracellular and/or transcelluar diffusion processes. These antigens may then be endocytosed by interstitial DCs, which transfer then to the MLNs. Finally, free antigens may diffuse from the lamina propria into the systemic circulation and get captured/endocytosed by DCs localized within other secondary lymphoid tissue such as the spleen and/or PLNs.
The effector arm of immunity against intraluminal bacteria is exerted by specific populations of T-lymphocytes, primarily CD4 + cells. The major subsets of CD4 + effector cells include the T helper cell-1 (Th1), Th2, and Th17 populations. These cells are identified by unique patterns of cytokine production and of chemokine receptor expression. Classical Th1 cells are produced in response to antigens derived from intracellular bacteria (and viruses) and secrete interferon-gamma (IFN-γ), interleukin-2 (IL-2), and lymphotoxin-α (LT-α). They can be distinguished from other effector cells by their surface expression of CCR1, CCR5, and CXCR3. Th2 cells are generated in response to parasitic helminthes, produce IL-4, IL-5, and IL-13 and express CCR3 and CCR4. Th17 effector cells develop primarily in response to certain extracellular bacteria and fungi, produce IL-17A, IL17F, IL-22, and express CCR4 and CCR6. It should be noted, however, that this Th1, Th2, and Th17 model of effector immunity may be a simplification as unique populations of differentiated T cells are constantly described. In addition, the whole concept of terminal differentiation of T cells has been challenged by the identification of considerable plasticity of effector CD4 + lymphocytes, which were shown to convert to other phenotypes under the appropriate immunological pressures.
The potential for the immune system to produce local and systemic tissue injury suggests that the immune system has developed ways to efficiently regulate immune responses to both pathogenic and harmless antigens. This regulatory activity is accomplished by a variety of different leukocyte populations. The best characterized of these populations are naturally occurring regulatory T cells (nTregs) expressing the phenotype CD4 + CD25 + Foxp3 + . These nTregs are known to suppress a wide range of immune responses via several different mechanisms including production of regulatory cytokines, competition for essential cytokines, and contact-dependent mechanisms. These Tregs are capable of inhibiting a variety of experimental autoimmune diseases including chronic colitis. In addition to CD4 + nTregs, studies have identified certain CD8 + T cells that are part of the intraepithelial lymphocyte (IEL) compartment in the gut and are capable of attenuating chronic colitis in vivo. Furthermore, specific subsets of B cells have been shown to function as regulatory cells in mice. It is becoming increasingly appreciated that IL-10 and TGF-β are required for the regulatory function of nTregs and B-cells in vivo and that both of these regulatory cytokines play important roles in continuously regulating immune responses to enteric bacteria such that gut is maintained in a state of immunological “tolerance” to commensal microorganisms.
65.5.2
Homing of Naive T Cell to GALT and MLNs
Naïve T cells constantly circulate in the blood and search for their cognate antigens. They encounter gut-derived antigens in the GALT and MLNs where such antigens have been concentrated and presented on the surface of DCs. Migration of naïve T cells from the blood to these sites follows the general adhesion and signaling steps of cell-trafficking, namely, selectin-mediated rolling, chemokine-triggered activation, and integrin-dependent arrest of T cells. These consecutive steps occur exclusively by way of HEVs, which are composed of specialized postcapillary venular endothelial cells in these lymphoid tissues. HEVs in the GALT are distinguished from venules of nonintestinal tissues because they affluently express MAdCAM-1, which serves as the receptor for integrin α4β7. HEVs associated with PPs contain MAdCAM-1, whereas those within MLNs express both MAdCAM-1 and peripheral lymph node addressin (PNAd). It is believed that T-cell-associated L-selectin (CD62L) binds to both MAdCAM-1 and PNAd to tether the lymphocytes to the HEVs to initiate T-cell rolling ( Fig. 65.7 A). Nevertheless, α4β7 may also be used by naïve T cells to tether themselves to MAdCAM-1, thereby providing additional tethering capacity for these cells in both PPs and MLNs. Another gut-selective molecule expressed in HEVs of the GALT is chemokine CCL21, which induces adhesion and chemotaxis through CCR7, a receptor that is expressed by naïve T cells but also by B cells. Further signals are provided by the Epstein Barr virus induced gene-1 ligand chemokine (ELC; CCL19) that is presented on the luminal surface of the HEVs. CCL19 leads to activation of LFA-1 and α4β7 on naïve T cells, thus facilitating their binding to ICAM-1 and MAdCAM-1, respectively. These interactions promote firm adhesion and lymphocyte arrest, which ultimately lead to T cell extravasation into the MLNs and PPs.

65.5.3
Homing of Effector Lymphocytes to GALT
Once naïve CD4 + T cells enter the MLNs (and/or PPs), they move into close proximity to the DCs and recognize their cognate antigens (Ag) of intestinal origin. Those are presented by DCs as an MHC-Ag complex to which T cells ultimately bind via their T-cell receptor. This process of antigen-specific priming of naïve T cell induces their differentiation to effector lymphocytes, which may acquire one of several immunophenotypes, mostly Th1 or Th17, and also promotes the clonal expansion of these effector cells within the lymphoid tissue. During this process, T cells shed their L-selectin and enhance their surface expression of specific “gut-homing” adhesion molecules such as α4β7 and CCR9 in addition to LFA-1, VLA-4 (α4β1), and CD44.
Following their priming/activation within the GALT/MLNs, antigen-experienced effector T-cells reenter the systemic circulation via the efferent nodal lymphatics. After this, lymphocytes may home to various locations within the gut with the use of relevant adhesion molecules. The latter may differ according to the specific intestinal region (small vs large bowel), the particular tissue compartment (intraepithelial vs lamina propria) but also the cell population (CD4 + vs CD8 +). For example, homing to the intraepithelial compartment may differ between the various subsets. Conventional or type a intraepithelial lymphocytes (IELs) come from circulating T cells, which become activated in the lymph nodes, where they acquire gut selectivity via upregulation of a4b7 and CCR9 expression. On the other hand, unconventional or type b IELs originate from CD8ab thymocytes. These cells do not go through imprinting in lymphoid tissues as they already demonstrate positivity for a4b7 and CCR9 when they leave the thymus.
Lymphocyte recruitment to the lamina propria has been much better delineated for the small intestine, than for the colon. According to the basic trafficking dogma, T cells that have been primed in the MLNs, preferentially home to the small intestinal lamina propria. This gut-selective imprinting requires signals from antigen-loaded intestinal DCs that produce retinoic acid. Furthermore, stromal cells also play a role. Such imprinting leads to the acquisition by lymphocytes of a “gut-signature” via the upregulation of the expression of integrin a4b7 and the chemokine receptor CCR9. α 4 β 7 binds to postcapillary venular MAdCAM-1, whereas CCR9 to CCL25. As MAdCAM-1 and CCL25 (TECK) are constitutively expressed on intestinal endothelial cells α 4 β 7 and CCR9 expressing lymphocytes are recruited to the small intestine. Nevertheless, further input is provided by interactions between lymphocyte-associated P-selectin glycoprotein ligand-1 (PSGL-1), LFA-1, α 4 β 1 and CD44 and venular P/E-selectin, ICAM-1 (and ICAM-2), VCAM-1 and hyaluronate, respectively ( Fig. 65.7 B). It should also be noted that during inflammatory conditions, there is upregulated expression of trafficking mediators that are not found in the small intestine under homeostatic conditions. A typical example is the increased expression of the nominally gut-exclusive VCAM1. This may lead to recruitment of recruitment of a4b1 lymphocytes to the inflamed gut.
Homing of lymphocytes to the colon is less well understood. In sharp contrast to the small intestine, the CCL25/CCR9 axis is not involved to T-cell recruitment in the colon as neither the expression of CCL25 is abundant in the colonic epithelium nor colon T cells express CCR9. Recently, GPR15 an orphan G-protein-linked receptor with homology to chemokine receptors has been proposed as an important mediator of T-cell recruitment to the large intestine. Studies in animal models of colitis have shown not only increased expression of GPR15 in colonic lymphocytes but also provided evidence for its functional significance for the localization of effector T cells to the large bowel and its pathogenetic significance for the development of colitis. On the other hand, GPR15 may also be implicated in Treg trafficking to the colon and, thus, confer antiinflammatory effects also. Nevertheless, this may be species-specific effect as human Tregs have not shown immunoreactivity against GPR15.
Another receptor/ligand pair of gut homing molecules is the CCR6/CCL20. CCL20 expression is constitutive at the epithelium that covers the PP follicles and the colon patches, but is also upregulated by inflammatory signals in intestinal epithelial cells. CCR6 is expressed by and involved in the distribution of Th-17 cells along the GI tract. CCR6/CCL20 interactions are distinguished by these between CCR9/CCL15 or α 4 β 7 /MAdCAM-1 in that they participate in T-cell trafficking to both small and large intestines and only under inflammatory conditions. Indeed, colonic tissues from clinical or experimental IBD express higher levels of CCL20 than uninflamed control tissue. Furthermore, blockade of CCL20 function leads to decreased T-cell adhesion to inflamed endothelia in mice with dextran sodium sulfate (DSS)-induced colitis. Finally, CXCR4 and its ligand CXCL12 may also contribute to lymphocyte localization to the gut.
Upon entrance to the gut interstitium, T-cells reencounter their cognate antigen presented on a more diverse population of antigen-presenting cells (APCs) such as macrophages and B cells as well as DCs ( Fig. 65.8 ). This secondary, antigen-specific interaction results in a more rapid and vigorous response of the effector T cells, thereby enhancing dramatically the production of IFN-γ, IL-17, TNF-α, lymphotoxin-α, and IL-2. IL-2 promotes the clonal expansion of T-cells and enhances the function of helper T cells and B cells, whereas IFN-γ interacts with and activates APCs and macrophages to produce additional IL-12. IFN-γ, TNF-α, and IL-17 will also activate endothelial cells thereby enhancing endothelial cell adhesion molecule expression on the postcapillary venular endothelium ( Fig. 65.8 ). In addition, IFN-γ potently activates macrophages and other myeloid cells produce large amounts of proinflammatory cytokines such as TNF-α, IL-1β, IL-6, IL-8, IL-12, IL-18, and IL-23 as well as reactive oxygen (superoxide, hydrogen peroxide) and nitrogen (NO) metabolites. The net result of this concerted interaction following T-cell activation is enhanced killing of ingested pathogenic microbes as well as increased recruitment of additional leukocytes (e.g., PMNs, monocytes, macrophages) into the gut tissue where they engulf and destroy the invading pathogens.

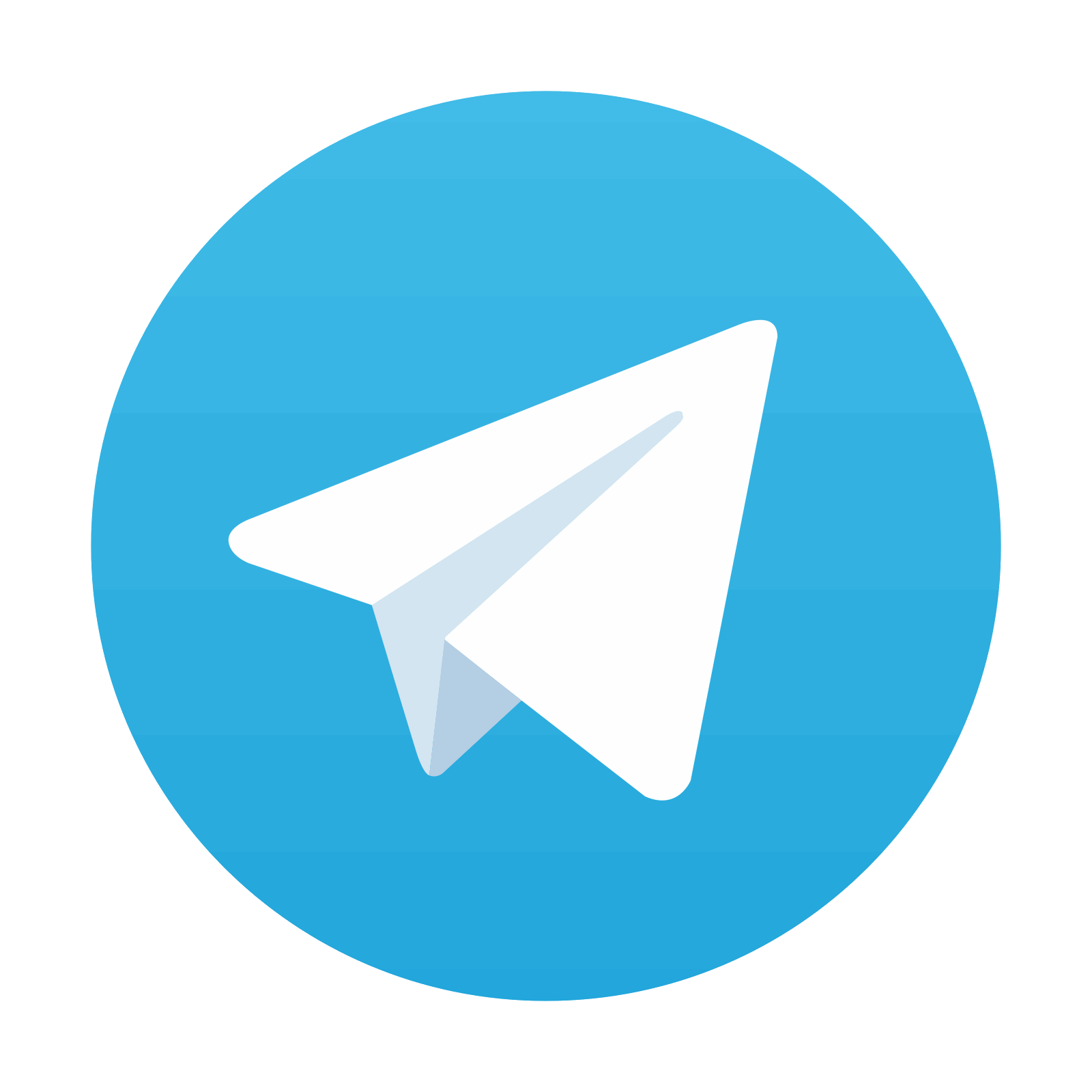
Stay updated, free articles. Join our Telegram channel

Full access? Get Clinical Tree
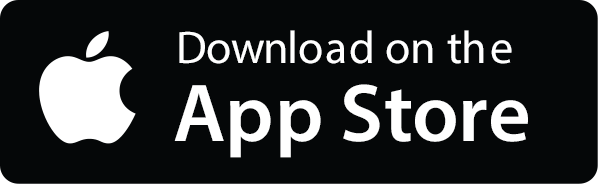
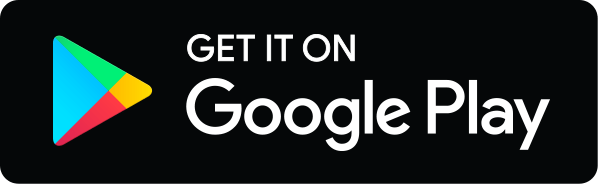
