Fig. 7.1
Polypropylene mesh. Normal or standard macroporous monofilament PP
Type II: Artificial microporous, with pores smaller than 10 μm in at least one of its three dimensions. The best example is the mesh Gore-Tex®, made with PTFE. See Fig. 7.2.
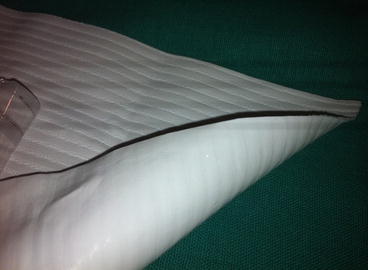
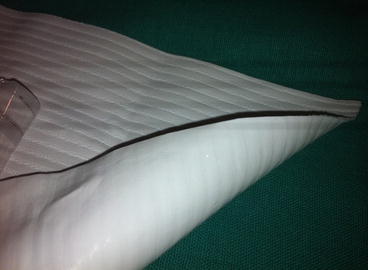
Fig. 7.2
Mycromesh® (L. Gore & Associates, Newark, DE, USA) PTFE-e. Biomaterial incorporates a microporous node and fiber structure with regularly spaced macropores. This structure ensures early fixation to host tissue with minimal foreign body response and extensive vascularization
Type III: Prosthetic components, macroporous or microporous multifilament (mixed mesh). Examples are braided polyethylene mesh (Mersilene™, Ethicon Endosurgery, Blue Ash, OH, USA), PP braided (Surgipro™ (Covidien, Dublin, Ireland)), or PTFE perforated (MicroMesh®, L. Gore & Associates, Newark, DE, USA). See Fig. 7.3.
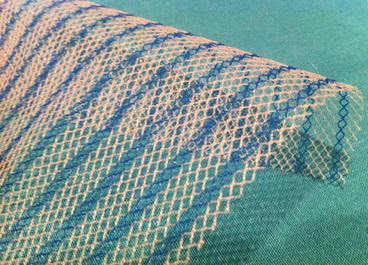
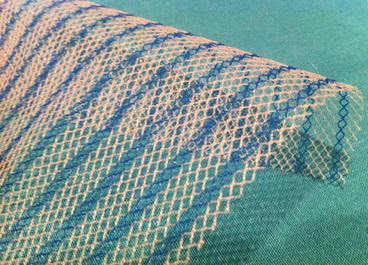
Fig. 7.3
Light Mesh. Ultrapro™ (Ethicon Endosurgery, Blue Ash, OH, USA) Partially Absorbable Lightweight Mesh is a unique and superior macroporous partially absorbable mesh
Type IV: Prostheses with pores smaller than 1 μm, such as silastic, and pericardium or dura substitutes. These meshes are not suitable for hernia repair, although biomaterials in combination with type I can make a surface suitable for intraperitoneal implantation.
Constant evolution has made the Amid classification obsolete. Reticular PP prostheses tend to have a smaller amount of the biomaterial in order to enhance integration into the host tissue and patient comfort, without compromising on the strength of the parietal repair. These prostheses also are associated with absorbable material, forming hybrid prostheses, leaving an even smaller amount of foreign material in the host organism [7].
PTFE also has been subjected to changes in its structure, after initially evolving toward a “multiperforated” patch without developing better biomechanical strength. In subsequent generations of this material, innovations have appeared: incorporation of a roughened surface which promotes integration via its outer face (DualMesh ®, L. Gore & Associates, Newark, DE, USA), argentic impregnation to prevent bacterial adhesion in the early stages after implantation.
Fully absorbable biomaterials have been incorporated into the context of prosthetic laminar: tissue extracted from its natural environment and serving as support for cell colonization. These are called biological prostheses; they achieve both repair and regeneration of host tissue, ultimately forming a “neopared” for the repair of the hernia defect. These natural prostheses require stringent controls given their animal origin, as they are not risk-free for possible transmission of disease as has happened in lyophilized dura prosthesis. The most commonly used are derived from the submucosa of porcine small intestines and collagen of human or porcine origin.
Finally, the composite type prosthesis or “composite” has opened a wide range of possibilities in the repair of large hernia defects in which the biomaterial must be left in contact with the visceral peritoneum. These prostheses have two components: one is the main or first biomaterial component, to which another is added in order to meet the specific function that can be performed first, so that the first material assumes the role of tissue integration, while the second is in contact with the peritoneal surface and modulates the behavior of this interface. The first component is usually a reticular prosthesis PP or polyester type; the latter may be absorbable or nonabsorbable, constituting a real barrier between the core material and the peritoneum and, in turn, promoting the generation of a mesothelial layer which prevents adhesions [7]. See Fig. 7.4.
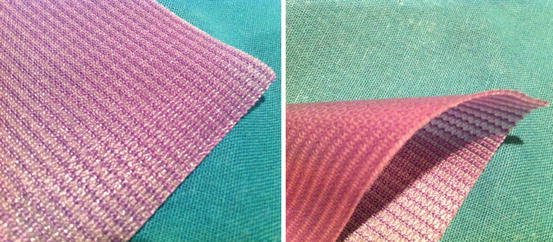
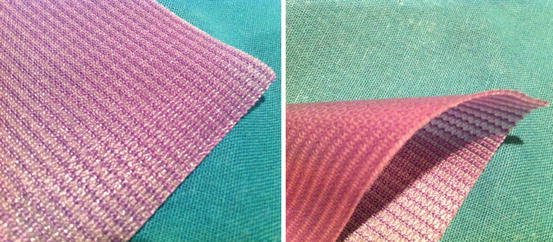
Fig. 7.4
Sepramesh™ (Davol, Warwick, RI, USA). Combines the strength of polypropylene mesh with a bioresorbable hydrogel coating for ventral hernia repair
Thus, the new classification is as follows:
Reticular prosthetics
Not absorbable: polypropylene (high or low density), polyester
Partly absorbable: polypropylene/polyglactin 910
Polypropylene/poliglecaprone
Absorbable: polylactic, polyglactin 910
Laminar prosthetics
Not absorbable: expanded polytetrafluoroethylene (ePTFE)
Silicone, polyurethane
Absorbable: porcine intestinal submucosa (SIS)
Composite prosthesis
Components nonabsorbable polypropylene/ePTFE
Polypropylene/polyurethane
Components absorbable polyester/polyethylene
Polypropylene/polyethylene
Polypropylene/hyaluronan
Polypropylene/polydioxanone/cellulose
As previously noted, research in the field of the host response to the implantation of the prosthesis has led to the modification of the PP reticular prosthesis in the sense of creating meshes with fewer biomaterials to expand the size base of pores and lower spatial rearrangement of the filaments, which will facilitate tissue growth between collagen fibers. This has led to the concept of “pore PP prosthesis, broad, and low density,” also called “lightweight,” with a similar behavior in terms of the tissue integration of classic PP meshes; this causes less foreign body reaction and maintains receptor tissue elasticity after implantation [7].
In an extensive and recent review published by Coda et al. [8], a total of 166 products registered as prosthetic biomaterial for abdominal wall repair were identified, of which 80 were made of PP, suggesting the following classification of biomaterials:
1.
Prostheses that are composed of a single biomaterial (PP, PET, PTFE, or PU PGA) with the same texture on both sides, mono- or multifilament, with or without drugs included
2.
Composite prostheses: made of two or more layers, one of which is simple, while the other or others are resorbable (A) or non-resorbable (B):
(A)
The non-resorbable layer or layers with or without drugs can be of PTFE, PU, PET, PP silicone, or condensed.
(B)
The layer or layers may be resorbable collagen, collagen + PEG+ glycerol + ORC PDO, CMC + HA, PVP + PEG, or O3FA.
3.
Prostheses composed of two woven materials:
(A)
Both materials nonabsorbable:
With coated filaments (e.g., titanium PP)
With interwoven strands (e.g., PP + PVDF)
(B)
Only one filament absorbable:
PP monofilament + PGCA
PP monofilament + PLA
PP multifilament + PG910
PET monofilament + PLA
PP monofilament + PGACL
This complex classification has been complemented with additional function of molecular weight of the prosthesis, a characteristic, as previously has been mentioned, that is gaining increasing interest. In the aforementioned review, 70 of the 80 registered PP prostheses had no information about molecular weight expressed in g/m2, and 40 of these also reported on thickness. This has given rise to proposing a classification that focuses primarily on PP reticular prostheses, which is justified because it is the most universal biomaterial used in the repair of abdominal wall hernias [8]:
1.
Ultralight prosthesis: <35 g/m2
2.
Artificial light: > 35 <70 g/m2
3.
Prosthetics standard: > 70 <140 g/m2
4.
Heavy prosthetics: > 140 g/m2
These new classifications of biomaterials are justified by the need to systematize the characteristics of the flood of products that the industry offers. The goal should be to agree on a “common language” that is being used by both surgeons and by the manufacturers of meshes, to collect the important features of the prostheses for application (molecular weight, thickness, tensile strength, wing rupture, elasticity, pore size, etc.) and to establish rules regarding the information that companies are required to provide about their products.
Relevance of the Molecular Weight, Pore Diameter, and Other Prosthetic Features
The purpose of surgical meshes in hernia repair is to reinforce and replace tissue for long-term stabilization of the abdominal wall. The introduction of meshes for inguinal hernia repair has reduced recurrence rates significantly.
The type of prosthetic mesh used for inguinal hernia repair is controversial. The traditional densely woven PP mesh induces a profound inflammatory reaction, leading to a firm scar plate with reduced elasticity of the abdominal wall. Theoretically, a lighter and softer mesh might be more beneficial by decreasing nerve entrapment and related pain by creating less fibrosis; it also appears compliant against surrounding tissues. The extent of the foreign body reaction with its provoked scar tissue formation depends on the amount and structure of the incorporated material [9].
The portfolio of meshes should be cost-effective, have no adhesion potential, exhibit excellent tissue integration and minimal shrinkage, have good memory, and be easy to use. Ideal meshes should not promote infection, fistula, or seroma formation and should not limit or negatively affect a patient’s normal activity.
Looking for the ideal mesh, the surgeon must now be familiar with their characteristics:
1.
Pore size and molecular weight
These two characteristics, closely related, can affect formation of adhesions following intra-abdominal placement, tissue integration, active surface area, elasticity, and memory.
The monofilament mesh with a pore size of 2.5 mm seems ideal because a bigger pore size improves the integration into the tissue and also preserves a high degree of elasticity and stability in the implant matrix.
Prosthetics with small pores (<1 mm) are “heavyweight,” whereas those with large pores are “low” weight. However, there are some meshes with low weight but small pores, and some heavyweight polymers have large pores.
Because porosity is more difficult to measure than weight, the term most often used by manufacturers is “weight,” so the molecular weight-related classification of prosthetics becomes important.
The use of lightweight meshes seems to reduce acute postoperative pain and discomfort compared with the use of traditional heavyweight meshes [10].
2.
