Fig. 3.1
Schematic drawing of prostate. Sagittal (a) and coronal (b) schematic drawings of prostate show anterior fibromuscular stroma (A), transitional zone (T), central zone (C), and peripheral zone (P). The upper part of prostatic urethra is surrounded by transitional zone and central zone, and the lower part of prostatic urethra is surrounded by peripheral zone. (c) Schematic drawing from posterior aspect of prostate shows seminal vesicle (S), vas deferens (V), and ampullary portion of vas deferens (A). Vas deferens forms ampullary portion with communication of seminal vesicle and connects ejaculatory duct (E). The ejaculatory duct communicates with prostatic urethra on verumontanum
The central zone is located in the upper anterior portion of the peripheral zone and surrounds the ejaculatory ducts. The central zone occupies about 20–25 % of the prostate. The immunohistologic features in central zone is quite different from the remaining part of the prostate. The reason is thought that the central zone is originated from the Wolffian duct, not urogenital sinus.
The transition zone occupies about 5 % of total prostate. As benign prostate hyperplasia (BPH) develops, the transition zone becomes the predominant components. The enlarged transition zone condenses the surrounding central zone into a thin rim. This rim serves as the pseudocapsule used as the plane for surgical treatment of BPH. BPH nodules very often demonstrate a thin, well-defined rim of low T2 signal intensity. Lack of this capsule has been described as a sign associated with central-gland cancers.
The periurethral glandular tissue represents less than 1 % of the volume of the prostate.
The signal characteristics of BPH on T2-weighted MR imaging are largely related to the composition of the BPH nodules. The BPH nodules with predominantly stromal and collagen components show hypointense signal intensity, and highly glandular BPH nodules show high signal intensity on T2-weighted MR image (Fig. 3.2).


Fig. 3.2
MR imaging of benign prostate hyperplasia (BPH). T2-weighted axial image shows well-marginated low signal intensity lesion replaced transitional zone (arrows)
The seminal vesicles are paired lobulated glands found immediately superior and posterior to the prostate base. They can be well demonstrated on axial and coronal T2-weighted MR images. The seminal vesicles have elongated, multi-septated, and fluid-filled structures mimicking bundle of grapes (Fig. 3.3).


Fig. 3.3
Normal appearance of seminal vesicle on MR. T2-weighted axial (a) and coronal (b) image shows elongated, multiseptated, fluid-filled structures mimicking bundle of grapes
3.3 US
3.3.1 Conventional US
For the ultrasound evaluation of the prostate, transrectal, transabdominal, and transperineal approaches are possible; however, TRUS is the most common method for evaluation of prostate due to high image quality. It provides excellent images of the gland, its boundaries, the adjacent bladder, seminal vesicles, and anterior rectal wall (Figs. 3.4 and 3.5).



Fig. 3.4
Normal transrectal ultrasound (TRUS) findings of prostate. Transrectal axial (a) and sagittal (b) image show normal anatomy of prostate. The peripheral zone (PZ) shows relatively homogenous slightly high echogenicity, whereas the central zone (C) shows relatively heterogeneous and low echogenicity

Fig. 3.5
Normal TRUS findings of seminal vesicle. Axial TRUS image reveals bundle of grapes appearance representing seminal vesicle
The main purposes of TRUS are calculating prostate volume; evaluating prostate, BPH, and prostatitis; and seeking the etiology of male infertility. Besides prostate evaluation, TRUS can be also used to evaluate the seminal vesicle, vas deferens, bladder, distal ureter, and urethra and to find out the etiology of hematospermia.
TRUS-guided procedures such as TRUS-guided biopsy or drainage are also commonly used. In case of lower urinary tract symptom, the evaluation of prostate volume is important for the diagnosis of BPH. The prostate volume is easily obtained by prolate ellipse volume calculation (π/6 × transverse diameter × anteroposterior diameter × longitudinal diameter). Transverse diameter and anteroposterior diameter can be commonly obtained from axial TRUS scan, and longitudinal diameter can be obtained from sagittal scan.
Typically the prostate gland is scanned in the axial plane, from the apex to the base (Fig. 3.4). The seminal vesicles are identified bilaterally, using an oblique sagittal view that also demonstrates the ampulla of the vas deferens (Fig. 3.5) [14]. In normal prostate, the peripheral zone shows high echogenicity and is routinely distinguished from the central gland, which is typically hypoechoic and heterogeneous owing to BPH (Fig. 3.6). Prostate cancer is characteristically identified as a round or oval focus of low echogenicity in the peripheral zone (Fig. 3.7); however, they may be isoechoic or hyperechoic [16, 17].



Fig. 3.6
TRUS findings of benign prostate hyperplasia (BPH). TRUS image shows round low echoic area suggesting benign prostate hyperplasia (BPH) arising from transitional zone (arrows)

Fig. 3.7
Prostate cancer shown as focal hypoechoic lesion on TRUS. (a) TRUS axial scan reveals well-marginated low echoic nodular lesion on left portion of prostate. (b) Color Doppler image shows definitely increased vascularity on the focal lesion
About 37–50 % of cancers are isoechoic or only slightly hypoechoic when compared with the peripheral zone [18]. The positive predictive value of the biopsy of a peripheral hypoechoic lesion is 25–30 % [16]. The differential diagnosis in evaluating the low echoic focal lesion includes inflammation, fibrosis, infarction, or benign prostate hyperplasia nodules [19].
B-mode TRUS has very limited ability to identify tumors in the central gland. It has been shown that some prostate cancers demonstrate increased flow on color Doppler, a feature that is also used to guide biopsies (Fig. 3.7) [9].
According to the study of Onur et al. [20], out of 3,912 consecutive patients who performed TRUS-guided biopsies, prostate cancer was detected in 25.5 % with a hypoechoic lesion and in 25.4 % without a hypoechoic lesion. The percentage of core detection was 9.3 % for hypoechoic and 10.4 % for isoechoic areas. Over the past decade, there has been a trend to obtain larger numbers of biopsy specimens, with most clinicians taking 8- to 12-core biopsies; most current studies are recommending a 12-core biopsy scheme [21].
Other attempts were tried. Lee et al. [22] tried to evaluate the imaging findings of focal hypoechoic lesions seen on TRUS with several parameters of shape, margin irregularity, vascularity, and the location of the lesion. They concluded that the positive predictive value was up to 80 % when the focal lesion located in peripheral portion showed nodular and irregular marginated hypoechoic lesion with increased vascularity.
In spite of these results, many reports showed that the simple hypoechoic lesion showed low sensitivity and specificity of grayscale ultrasound for the detection of prostate cancer. And there is limited value for grayscale-targeted biopsies. However, due to the high-quality images and the inexpensive and simple procedure, it is still the most optimal technique for guiding prostate biopsies [23].
Besides, recently, new emerging technologies in TRUS-guided prostate biopsy such as image fusion, elastography, and contrast-enhanced ultrasound were introduced and showed high potential in the diagnosis of prostate cancer. Ultrasound contrast agent studies can provide the information regarding vascularity of the lesion. New novel technologies for the synthesis of new microbubbles with specific ligand are introduced and visualize the presence of specific marker. The advent of ultrasonic molecular imaging may provide a new diagnostic method for the early diagnosis of prostate cancer.
Elastography is an emerging ultrasound technique that can provide the information regarding tissue elasticity and stiffness. The image fusion, which can show the information both from multiparametric magnetic resonance imaging (mpMRI) and TRUS imaging, will have a potential modality in performing targeted biopsy.
3.3.2 US Elastography
Elastography is an ultrasound imaging technique based on the concept that significant differences exist between elastic properties of benign and malignant tissue [24]. It is an emerging ultrasound technique that can visualize tissue elasticity and stiffness [25]. It is based on the assumptions that if force is applied to the unit area (stress), relative displacement of points (strain) will be proportional to the applied force and is represented by well-described Young’s modulus. Tumors are usually stiffer than normal tissue because of its increased cellular density. Prostatic cancer is normally 5–28 times stiffer than the surrounding soft tissue [26]. This change of local stiffness is the background of digital rectal exam of prostate gland. However, digital rectal exam is subjective to the examiner, and only part of the prostate is palpable.
In elastography, there are two types, using strain and shear wave technology. Strain forces are generated by manual compression by transducers, while shear wave is a technique that uses a sonographic push pulse to generate a shear wave in the tissues (Figs. 3.8 and 3.9) [27].



Fig. 3.8
Elastography using strain forces. Elastography shows relatively focal lesion showing low elasticity (arrows). This lesion is well matched with the focal lesion on grayscale TRUS

Fig. 3.9
Prostate cancer of shear wave elastography. (a) Elastography shows area of low elasticity in left peripheral zone of prostate (arrows), which is not definite on grayscale TRUS. Pathologic report revealed that prostate cancer was in this lesion. (b) Apparent diffusion coefficiency (ADC) map image shows diffuse signal drops in whole prostate, suggesting diffuse prostate cancer
Soft tissues tend to exhibit higher strain (deformation) than stiffer areas when compression is applied. Strain elastography of the prostate is based on the analysis of tissue deformation in a region, generated by compression of the tissue by the transrectal transducer. A speckle comparison, before and after compression, yields to a color map of local tissue deformation or strain called elastogram [28]. Because tissue stiffness is estimated by visualizing the differences in strain between adjacent regions, there is no quantitative elasticity.
The ROI can be placed on abnormal lesion and the other areas of the prostate. Semiquantitative information can be derived by measuring strain ratio between two ROI (usually one considered “normal” and the other “abnormal”). The strain for each pixel is color coded and displayed as overlay on the B-mode image (Fig. 3.8). Because the stiffness color scale is automatically distributed from the lowest to the highest strain found in the image plane, it may induce some variability [29]. Elastography permits depiction of the cancer of isoechogenecity on grayscale ultrasound, otherwise can be missed by conventional TRUS.
Shear wave elastography (SWE) is another type of elastography, which can provide quantitative information on tissue elasticity. The basic principle of SWE relies on two successive steps; a shear wave is remotely induced by the endorectal transducer in the prostate, using the acoustic radiation force of a focused ultrasonic beam, and the shear wave propagation is captured by imaging the prostate. By measuring the shear wave propagation velocity, shear modulus can be derived. The shear wave speed (in meter per second) or the Young’s modulus (in kilopascal) is color mapped on the images in B-mode [29].
Unlike strain elastography, SWE requires no compression on the rectal wall to produce elastograms (Fig. 3.9). SWE is based on the measurement of shear wave velocity propagating through the tissues [30]. This technique has advantages of providing a quantitative map of elastic characteristics in real time, which can be displayed either in kilopascal or in meter per second.
Several studies reported that elastography provides useful additional information to conventional TRUS for prostate cancer detection. There are several applications of elastography. It can be used in characterization of region detected by grayscale imaging, color Doppler US, or MRI (Fig. 3.9). It can be also used for the characterization of lesions which were not detected on conventional US, and it can be used for biopsy targeting (Fig. 3.10) [31].


Fig. 3.10
Figure showing shear wave elastography-guided biopsy. TRUS image shows shear wave elastography-guided biopsy. The guide indicates that the lesion with low elasticity
A meta-analysis study using strain elastography reported sensitivity in the range of 71–82 % and a specificity of 60–95 % with reference standard of radical prostatectomy specimen [28]. Elastography-guided prostate biopsies in patients with cancer were 2.9-fold more likely to detect prostate cancer than systemic biopsy [32].
Another prospective study of elastography by Brock et al. reported that overall prostate cancer detection rate was significantly higher in patients who underwent biopsy with the elastography-guided approach compared to the grayscale ultrasound-guided biopsy (51.1 % vs. 39.4 %) [33]. However, the sensitivity of elastography did not reach levels to omit a systematic biopsy approach.
The comparison of elastography with T2-weighted conventional MRI was reported by Aigner et al. [34]. Overall sensitivities and specificities were similar between elastography and T2-weighted MRI.
The drawback of strain elastography is that quantification of tissue elasticity is not achievable. Semiquantitative stiffness evaluation using a strain index (strain ratio of tissue over normal tissue) is introduced to overcome this limitation and reported to be useful in the evaluation of prostate cancer [35]. However, these studies are all based on region of interest drawing, which have some difficulties in reproducibility and standardization.
With SWE, during evaluation, the transducer should be kept in steady-state position for several seconds until stabilization of the signals. Usually, stiff tissues are color coded in red, while soft tissues appear in blue color [29]. Hypoechoic lesions with stiff elasticity are suspicious for malignancy. The absolute value of elasticity can be obtained for each ROI. In young men without prostate disease, the elasticity values are below 30 kPa. Typical prostate cancer nodules in the peripheral zone are stiffer, showing more than 35 kPa [29].
The advantage of SWE over strain elastography is that SWE does not require compression by the transducer, which means that measurement is operator independent. A few initial reports showed very promising results for SWE, including high sensitivities and specificities over 90 % for prostate cancer [27, 36]. However, the sensitivity and specificity were decreased to be 50–60 % in another recent study by Woo et al. [37]. Nevertheless, SWE parameters of mean stiffness and mean stiffness ratio are significantly different between prostate cancer and benign tissue and correlate with Gleason score.
For the evaluation of diagnostic accuracy or feasibility, more validation studies beyond the initial reports will be required with prospective trials and/or radical prostatectomy specimen basis.
Elastography is rapidly maturing and now produces excellent and diagnostically useful images of prostate. By measuring the stiffness, it may help for the characterization of the focal lesion seen on TRUS and for guiding the prostate biopsy.
3.3.3 Contrast-Enhanced TRUS
Microbubble contrast agents are composed of tiny bubbles of gas contained within a supporting shell. The shell may be composed of albumin, galactose, lipids, or polymers ranging from 10 to 200 nm [38]. The inner space is filled with gases having a high molecular weight and low solubility such as perfluorocarbon or sulfur hexafluoride which has characteristics of prolonging the agents’ existence in the blood pool [39].
Ultrasound contrast agents are mainly used as intravascular contrast media, although they can be instilled into the urinary bladder to evaluate ureteric reflux or into the uterus to look out for tubal patency [40, 41]. The size of microbubbles is nearly equal to that of red blood cells, and they behave as intravascular blood pool agents (Fig. 3.11). They are able to better show the neovascularity and increased vascular density that has been correlated to the presence of prostate cancer, cancer stage, metastasis, and postoperative biochemical failure [42, 43].


Fig. 3.11
Figure showing microbubble. Microscopic image shows bubble like structures with the size less than 10 μm
The echogenicity of an individual microbubble is dependent on the elasticity of the shell, the compressibility of the core, the length of time that the bubble will remain in circulation, and the amount of acoustic energy that the microbubbles can absorb before breaking down [44].
Prostate cancer, like other solid organ malignancy, has been demonstrated to have measurable neovascularity and tumor-associated angiogenesis. The tumor growth and metastasis require angiogenesis, the growth of new blood vessels. These abnormal blood vessels may represent a possible marker for the presence of cancer [42]. The measure of tumor angiogenesis correlates with the microvessel density (MVD) and metastasis in various malignancies [45]. Hence, microbubbles have considerable potential for imaging of tumor angiogenesis as intravascular contrast agents. According to preclinical study in xenograft prostate cancer model using PC-3 prostate tumor cells, maximum intensity was positively correlated with the MVD with statistical significance (Figs. 3.12 and 3.13) [46]. In clinical study, Weidner et al. reported that microvessel counts increased with increasing Gleason score in prostatectomy specimen [45]. Sedelaar et al. showed that ultrasound contrast-enhanced areas had a 1.93 times higher MVD as compared to the nonenhanced areas (Fig. 3.14) [47].




Fig. 3.12
Xenograft animal study showing microbubbles for angiogenesis assessment. Contrast-enhanced US shows well-enhancing solid mass on PC-3 xenograft mouse model (Published with kind permission of Korean Society of Ultrasound in Medicine J Korean Soc Ultrasound Med 2009;28:139–145)

Fig. 3.13
Quantitative evaluation of tumor angiogenesis. Time-intensity curve can be obtained with software. Several quantitative parameters can be calculated based on time-intensity curve. Published with kind permission of Korean Society of Ultrasound in Medicine

Fig. 3.14
Contrast-enhanced TRUS showing prostate cancer. Contrast-enhanced TRUS shows well-enhancing lesion on right peripheral zone of prostate (arrows). The lesion showed prostate adenocarcinoma after TRUS-guided biopsy
For the contrast-enhanced ultrasound, various imaging strategies including grayscale, Doppler, harmonic imaging, maximum intensity projection, and flash replenishment techniques can be used. Harmonic imaging uses the distortion produced by the microbubble contrast agent on the sound wave reflection, in harmonic multiples of the incident frequency [38]. Maximum-intensity projection adds the information between ultrasound frames, allowing tracking of microvessel morphology.
In clinical practice, contrast-enhanced sonography has many advantages in that it can be performed on patients safely, easily, and repeatedly without any radiation. However, it has disadvantages in its lack of objectivity in determining the extent of enhancement because the image qualities are affected by many factors. Also the results are sometimes operator dependent. However, these disadvantages may be overcome by development of dedicated software for analysis.
For the evaluation of TRUS-guided biopsy using ultrasound contrast agents, many clinical studies were performed. The detection rate of prostate cancer was higher when they use contrast-enhanced color Doppler-targeted biopsy comparing 10 systemic biopsies in 690 men (26 % vs. 20 %). And, the Gleason score was also higher in contrast-enhanced targeted biopsy than that of systemic biopsy (mean, 6.8 vs. 5.4) [48]. In a recent report published by Jiang, the peak intensity on contrast-enhanced ultrasound correlated with Gleason score and MVD in 147 prostate cancer patients [49].
According to the meta-analysis regarding the diagnostic performance of contrast-enhanced ultrasound in patients with prostate cancer, the pooled sensitivity and specificity were 0.7 and 0.74 from 2,624 patients [50]. At present, contrast-enhanced ultrasound is a promising tool in the detection of prostate cancer, but it cannot completely replace systematic biopsy under the present circumstances.
Targeted microbubbles have additional ligand molecules that bind to the specific sites. Possible receptor targets for prostate cancer are those that are upregulated during the process of angiogenesis. Most research regarding targeted microbubbles has been focusing on the vascular endothelial growth factor (VEGF) receptors [51]. For contrast-enhanced imaging of the high expression of VEGF receptor 2 (VEGFR2) in tumor neovasculature, VEGFR2 receptor-loaded targeted microbubbles were introduced [52].
Recently, there are other novel technologies for targeting prostate cancer cells using nanoscale ultrasound contrast agents. Among prostate cancer biomarkers, prostate-specific membrane antigen (PSMA) is considered to be the most important protein target in diagnostic-specific immunolocalization imaging and immune-directed therapy [53, 54]. PSMA is a type II transmembrane glycoprotein in the prostate cell membrane. The levels of PSMA expression are different in normal prostate tissue, benign prostatic hyperplasia, and prostate cancer epithelial tissue, respectively. And it is also known that positive PSMA expression rate in hormone-refractory prostate cancer and metastases are significantly higher than that of the normal or benign tissue [53]. Wang et al. [54] reported in vitro and in vivo results of PSMA-targeted nanoscale microbubbles in prostate cancer. They synthesized stable PSMA monoclonal antibody-loaded MBs and investigated their in vitro target-binding capability with the selected prostate cancer cells. Besides, targeted contrast enhancement and specificity were also examined with a xenograft prostate tumor models. The results showed that targeted microbubbles can significantly increase peak intensity and duration of contrast enhancement than that in control microbubbles [54]. These targeted ultrasound contrast agents, which are on the way of development, would be very potential methodologies not only for prostate cancer-specific imaging but also for targeted ultrasound-guided prostate biopsy.
3.3.4 Image Fusion
TRUS plays a crucial role in the screening imaging study and guidance of the biopsy of the prostate glands. However, overall detection rate of TRUS for prostate cancer remains approximately 50 %, and biopsies yield at least one positive biopsy in only 25 % of the patients [55, 56]. Moreover, over detection of the clinically insignificant cancer is another important issue, which leads to overtreatment. Therefore, detection of highest grade or representative cancer tissue in the prostate gland is required to decide treatment plan. Several potential benefits of MRI-targeted biopsy were reported in the literature. The accurate localization of significant cancer before biopsy may correct the limitations of systematic random biopsy [6].
Introduction of high-field MRI in clinical field impacts improved image quality due to increased signal-to-noise ratio (SNR). Still there remain some controversies, but the use of uncomfortable endorectal coil is not obligatory for the prostate MRI because of its increased SNR of 3.0 Tesla MRI [57].
Application of stronger magnet and multiparametric MRI (mpMRI) from morphologic and functional MRI technique enables to detect more cancers. T2-weighted MRI is excellent in the evaluation of anatomy and detection of peripherally located cancer. However, T2-weighted image has limited value in the detection of central-gland cancer. In addition, T2-weighted images are very susceptible to post-biopsy hemorrhage, which shows low signal intensity and interferes tumor detection [58].
Functional techniques including apparent diffusion coefficient (ADC) map from diffusion-weighted imaging, dynamic contrast-enhanced MRI with fast imaging, and magnetic resonance spectroscopy (MRS) are very helpful in the diagnosis of prostate cancer. The detection rates of prostate cancer are increased with these techniques, and even centrally located cancer can be more easily and confidently diagnosed [59]. For the staging of prostate cancer, mpMRI is superior to detect extracapsular extension and seminal vesicle invasion. To monitor treatment effect, mpMRI significantly improves the assessment of patients with suspected recurrence after treatment [60].
ADC map can discriminate cancers with Gleason score over 7 (4 + 3) from cancer with lower Gleason score [59]. Because of this superior detectability of cancer with highest Gleason score with MRI, MRI-guided prostate biopsy is introduced. Although it has great advantage of reducing the number of core biopsy, it has limited value due to increased procedure time and costs [61].
The simplest way to MRI-guided biopsy is visual estimation of MRI during TRUS-guided biopsy. Visual estimation allows the adaptation of MRI-targeted biopsy in clinical practice without significant cost, but it needs a significant learning curve and lacks real-time feedback regarding accuracy [6]. The current studies about TRUS-guided biopsy with MR visual estimation suggest improved accuracy and efficiency compared to systematic biopsy but also demonstrated that visual estimation biopsy varies by operator experiences [62, 63].
MR-US fusion image by co-registration software can be another powerful option for guidance of prostate biopsy (Fig. 3.15). Image fusion is the process of combining image information from two or more images into a single image, and the resulting image provides more information than any input image alone. Image fusion of prostate refers to the superimposition of stored prostate MRI images and real-time TRUS images, when prostate biopsy is performed [64]. The fused image gives the operator the advantages of the tumor-detecting value of MRI with the ease of use of TRUS.


Fig. 3.15
Image showing MRI-TRUS fusion. TRUS-MR fusion image-guided biopsy can cover the most suspicious lesion seen on ADC map. TRUS guide biopsy was performed on the suspicious lesion seen on ADC map in anterior portion of transitional zone, and pathologic report revealed prostate cancer with Gleason score of 8 (arrows)
A number of commercial platforms for image fusion have become available [6]. These applications vary by co-registration technologies including mechanical, electromagnetic, or real time. Hardware platform to align the biopsy is different.
Reduction of time and cost of direct MRI guidance without sacrificing diagnostic accuracy can be achievable. Sonn et al. [61] reported that targeted biopsy with MR-US fusion was three times more likely to identify cancer than a systematic biopsy (27 % vs. 7 %). Out of the men with Gleason score 7 or greater cancer, 38 % had disease detected only on targeted biopsies. Fusion biopsy can provide improved detection of prostate cancer in men with prior negative biopsies and elevated PSA levels [65]. Fusion software co-registration potentially overcomes the limitation of cognitive fusion through reproducible methods of identifying MRI lesions on ultrasound. MR-US fusion biopsy potentially has greater reproducibility because it involves less operator dependence and provides real-time feedback of actual biopsied locations.
There is still some technical issue to be solved in MR-US fusion. Precise registration of MRI and US is the key for the successful image fusion. However, the shape of prostate in MR and TRUS is different because the prostate is displaced and deformed due to ultrasound probe. Nonrigid registration of the prostate gland for this elastic deformation is needed, but still many fusion techniques are based on rigid registration which cannot reflect elastic deformation by transducer. However, this issue can be overcome by development of fusion techniques [66]. Disadvantages also include a high cost for the image fusion software/device, dependence on the software for accuracy, and the associated learning curve and operator training.
Among the patients with no previous biopsy, the role of image fusion is poorly defined, but it has the potential to reduce false-negatives contributing to reduce repeated biopsies and to get the representative information regarding patients’ cancer. The patients with a previous negative biopsy but persistent clinical suspicion have the potential for increased cancer detection and reduced further repeated biopsy. With development of MRI technologies for depicting the prostate cancer, image fusion may have more important role in prostate cancer patients.
3.3.5 TRUS-Guided Biopsy
The diagnosis, management, and prognosis of prostate cancer are largely dependent on histopathological features obtained by TRUS and TRUS-guided biopsy [14]. Traditionally, the decision to perform prostate biopsy has been based on abnormal digital rectal examination (DRE) or increased PSA level. TRUS-guided biopsy uses B-mode and/or color Doppler imaging to localize the prostate, target suspicious areas, and systematically obtain anywhere from 6- to more than 40-core biopsy using 18-gauge needles.
Because of these sampling errors, however, the reported false-negative rate of TRUS-guided biopsy reaches up to 30 % [67]. In addition, Gleason scores established through TRUS-guided biopsy are often discordant with prostatectomy specimens. TRUS-guided biopsy underestimates the score of cancer in up to 38 % of patients, therefore misrepresenting tumor aggressiveness [68]. The volume of disease can be estimated based on the number of positive core biopsies and on the percentage of tumor seen in positive cores. According to Park et al., among several parameters from prostate biopsy, the maximum cancer length was found to be the most reliable predictor of disease staging [69]. In other studies, multiple risk-stratification schemes are available to predict prognosis and plan treatment [70, 71]. These criteria rely heavily on the histopathologic findings of Gleason grade and extent of tumor seen in the TRUS-guided core biopsy specimens.
Although there is no absolute cutoff value for prostate biopsy, general agreement is that PSA higher than 4 ng/ml is suggestive of the presence of prostate cancer for TRUS-guided biopsy [31]. In other reports, a PSA threshold of 2.6 ng/ml doubles cancer detection rates in men younger than 60 years with little loss in specificity [72]. There are several other factors to consider in proceeding to biopsy, potentially including PSA velocity, % free PSA, PSA density (PSAD), age, family history, ethnicity, and comorbidities.
The National Comprehensive Cancer Network (NCCN) in the United States recommends the use of a PSA velocity of 0.35 ng/ml/year or greater as indication for biopsy in men with PSA 2.5 ng/ml or less [73]. The use of % free PSA as an alternative indication of initial biopsy was also suggested. The % free/total PSA ratio can be used to recommend biopsy if the value is less than 10 %, and prostate biopsy is not recommended if the value is greater than 25 % [31]. PSAD and age-referenced PSA have been investigated but are still controversial [73]. Several predictive models have been developed to reduce unnecessary biopsies and still detect most clinically important cancers and are available on the internet.
Pain control is one of the important issues in the aspect of patient care. Periprostatic nerve block (PPNB) with 1 % lidocaine is considered the standard anesthetic technique. And many protocols and researches were reported to reduce the pain during TRUS-guided biopsy [74, 75]. The most common site of injection is bilaterally at the junction of the base of the prostate and seminal vesicles, because neural pathway originates from the inferior hypogastric plexus located at the tip of seminal vesicles and passes between the prostate and rectum [76]. Various sites of infiltration were studied including the apex-only [77, 78] bilateral neurovascular bundles [79, 80], apex and neurovascular regions [81, 82], or three locations of base, mid, and apex [82]. Ismail et al. reported that combined bilateral neurovascular bundles with apical infiltration resulted in more effective reduction in pain associated with TRUS biopsy than bilateral neurovascular infiltration alone, especially in younger patients [83]. Lee et al. reported that intraprostatic anesthesia and periprostatic nerve block are effective and useful techniques tolerated by the patient based on randomized, double-blinded study [84]. In other respect, for the relieving pain related to probe insertion, Cormio et al. reported that lidocaine-prilocaine cream was most effective in probe-related pain for prostate biopsy [81].
The number of cores from TRUS-guided biopsy is also important because it is related with the patient’s discomfort or the accuracy of prostate cancer diagnosis. Recently, TRUS-guided biopsy with 12 cores is considered the standard procedure for cancer detection. Several studies have reported the higher yield of an extended prostate biopsy versus the old standard sextant biopsy, without an increase in the detection of insignificant cancer [75, 85]. The studies regarding the number of core biopsy have demonstrated sextant prostate biopsy sensitivities at 30 %, with increasing sensitivity with increasing numbers of core biopsies, 36–58 % for 12-core biopsies and 53–58 % for 18-core biopsies [86]. On the other hand, it has been shown that the saturation technique as an initial prostate biopsy strategy does not improve cancer detection. Jones et al. had suggested that further efforts at extended biopsy strategies beyond 10–12 cores are not appropriate as initial biopsy strategy [87]. Eichler et al. showed that there is no significant benefit in taking more than 12 cores and methods requiring more than 18 cores have a poor side-effect profile [21]. Sampling accuracy tends to decrease progressively with an increasing prostate volume. In addition to the number of cores and prostate volume, the site of core biopsy is also important. Because the apex and the base of the peripheral gland are the most common cancer sites, it is advised that biopsies should be directed to apex and base of peripheral zone. The parasagittal biopsies have been shown to have the lowest possibility of prostate cancer at the initial biopsy. The American Urological Association (AUA) has recently completed a white paper confirming that a 12-core systematic biopsy is optimal and that there was not compelling evidence that individual site-specific labeling of cores benefits clinical decision making regarding the initial management of prostate cancer [31]. Studies of repeat prostate biopsy after negative extended prostate biopsy indicate that up to 30 % of patients have cancers that were not previously diagnosed [88, 89]. Repeated biopsy seems to be recommended in patients with an initial negative biopsy but persistent suspicion of prostate cancer based on age, comorbidities, DRE findings, PSA measurements, other PSA derivatives (% free PSA, PSAD, PSA velocity), or urinary prostate cancer antigen 3 (PCA3) score [28].
While MRI-targeted biopsy and MR-TRUS fusion biopsy have the potential to overcome the limitations of standard TRUS-guided biopsy, they need further studies regarding the accuracy in prostate cancer detection, cost-effectiveness analysis, and improvements in technical limitations [6].
The complications of prostate biopsy include urinary tract infection, hematuria, bleeding per rectum, hematospermia, and acute urinary tract obstruction. In case of bleeding, clinically significant hematuria was developed only in 1–2 % of patients who underwent prostate biopsy. Desmond et al. reported that out of 670 patients who underwent prostate biopsy, about 0.6 % showed gross hematuria and 0.1 % of patients needed management [90]. Azjen et al. reported that 1 % of the patients showed transient hematuria [91]. In larger scales, Berger et al. showed hematospermia (36.3 %), hematuria (14.5 %), and rectal bleeding less than 48 h (2.3 %); however, most cases need no management. They reported that only 0.7 % of patients showed fever immediately after biopsy, 0.6 % showed severe rectal bleeding, and 0.2 % showed acute urinary retention [92]. Infections after prostate biopsy are urinary tract infection, prostatitis, epididymitis, prostatic abscess, or sepsis. To avoid significant infection, it is important to use prophylactic antibiotics before biopsy and to detect the symptoms and signs of infection earlier.
3.4 CT
Computed tomography (CT) is one of the most commonly used modality in abdomen and pelvis imaging; however, it gives little information regarding prostate and has a very limited role in prostate cancer staging [93]. CT shows little contrast between prostate and adjacent organs including seminal vesicle. However, it may be considered for the staging of men with high-risk prostate cancer. High risk is usually defined as total serum PSA greater than 20.0 ng/ml, locally advanced disease on clinical assessment such as positive findings on DRE, or Gleason score greater than or equal to 8 [14]. CT of prostate cancer is almost used for assessing potential metastasis in lymph nodes, soft tissues, or bones of advanced prostate cancer patient (Fig. 3.16). The most common sites for lymph node metastasis in prostate cancer include anterior or lateral route of pelvic pathway (external iliac lymph nodes), internal iliac route (internal iliac lymph nodes), and sometimes presacral route (presacral lymph nodes). Even in patients with high-risk cancer, the sensitivity of CT scanning for detecting positive nodes is only about 35 % [14].


Fig. 3.16
CT findings of multiple metastasis of prostate cancer. (a) Post-contrast CT scan shows bulky mass involving prostate and posterior bladder wall (arrows). (b) At the higher level, CT scan shows multiple metastatic lymphadenopathies (arrows)
For the diagnosis of bone metastasis, bone scan is significantly more sensitive than CT, and many patients with normal CT findings show positive radionuclide scan findings. Although osseous metastases can be identified on CT, CT is usually used to follow up patients with known metastatic disease rather than to diagnose it.
3.5 MRI
3.5.1 Conventional MRI
Usually, prostate MRI studies combine anatomic images from high-resolution T2-weighted (T2W) images and functional information obtained from diffusion-weighted imaging (DWI), dynamic contrast-enhanced imaging (DCEI), and MRS, in a multiparametric approach.
High-resolution T2-weighted images are used for prostate cancer detection, localization, and staging. Many institutions obtain T2-weighted sagittal, axial, and coronal planes. Fast-spin echo T2-weighted image provides high signal-to-noise ratio (SNR) and high spatial resolution images with significant T2 contrast. High-contrast T2-weighted images allow the depiction of subglandular structures located mainly in the peripheral zone.
In T2-weighted images, the normal peripheral zone of the prostate has hyperintense signal, whereas the central and transition zones have low signal intensity, allowing the zonal anatomy of the prostate to be clearly demonstrated (Fig. 3.17). The prostate capsule is demonstrated as a thin line of low signal intensity surrounding the gland [94]. The nodular, glandular, stromal, and cystic changes of BPH can be clearly visualized [95]. For T2W imaging without functional sequences, the sensitivity and specificity for prostate cancer are approximately 57–83 and 62–82 % [96, 97].


Fig. 3.17
Normal findings of prostate in 45-year-old man. (a) T1-weighted axial image shows homogenous low signal intensity of prostate. (b) T2-weighted axial image shows bright signal intensity of peripheral zone. Also note low signal intensity transitional zone (T) and anterior fibromuscular stroma (F) in central and anterior portion of prostate. (c) Post-contrast MR image shows relatively well-enhancing transitional zone (T) and anterior fibromuscular stroma (F). Also note the peripheral zone shows little enhancement due to prominent glandular portion (P)
Normal peripheral zone consists primarily of glandular lumen lined with secretory epithelium, all of which is embedded within a stromal matrix [98, 99]. In normal structures, macromolecular water content and free water content are low in stroma and high in luminal space on T2-weighted images. These characteristics result from relatively long T2 values and unrestricted water diffusion with high ADCs in the peripheral zone. Peripheral zone prostate cancer typically shows low signal intensity nodule on T2-weighted images, but imaging findings can be changed according to the tumor growth pattern and the aggressiveness of the tumor. In case of tumors with densely packed malignant glands, T2-weighted image shows low-signal-intensity mass usually on the peripheral zone (Fig. 3.18). However, in case of tumors with sparse malignant glands intermixed with normal tissue, the signal intensity of the tumor is not significantly different from the surrounding normal tissue and is not readily detected on MRI [100]. Sometimes it is difficult to differentiate prostate cancer from other benign lesions showing low signal intensity on T2-weighted image. For focal low signal intensity on the T2-weighted images, wedge-shaped and diffused extension without mass effect are the best predictors of benignity, whereas mass effect and irregular margin suggest malignancy [101]. A few benign conditions of the prostate, including chronic prostatitis, scars, and post-biopsy hemorrhage, can result in low-signal-intensity areas on the T2W images and can be confused with cancer [102]. In case of prostatitis, the glandular luminal space is replaced by the inflammatory cells. These inflammatory changes with scar formation due to the thickening of the ductal walls contribute to shorter T2 values, which reveal subsequently hypointense regions on T2-weighted image [103]. Sometimes age-related changes in the prostate show increase in ADC in the peripheral zone [104].


Fig. 3.18
Typical findings of prostate cancer. (a) T2-weighted axial scan shows well-marginated low signal intensity lesion located in left peripheral zone (arrows). (b) T1-weighted image also shows low signal intensity in left peripheral zone (arrows). Also note high signal intensity lesions in peripheral zone suggesting hemorrhage probably due to previous biopsy. (c) TRUS shows relatively irregular marginated low echoic lesion in same area (arrows). (d) Color Doppler image shows increase vascularity. The pathologic report after prostatectomy showed prostatic adenocarcinoma
The detection of tumors located in the transition zone is more difficult. Because the signal intensities of BPH and prostate cancer usually overlap on the T2-weighted images, other imaging features such as homogeneous low T2W signal intensity, ill-defined margins, lack of capsule, lenticular shape, and extension into the fibromuscular stroma may help for the suspicion of prostate tumor [105].
Although post-biopsy hemorrhage may give us confusion, sometimes the hemorrhage can support the detection of a suspected lesion known as “hemorrhage exclusion sign.” It is noted when the tumor composed of compact tumor cells presents as an isointense area on the T1-weighted images, surrounded by hyperintense hemorrhage in normal tissue [106] (Fig. 3.18).
To avoid unnecessary diagnostic difficulties due to biopsy hemorrhage, prostate MRI is usually recommended at least 6–8 weeks after biopsy; however, post-biopsy hemorrhage can also persist for several months [95]. It is presumed that the anticoagulative effects of the citrate which is highly concentrated in normal prostate tissue but is low in cancer tissue results in increased bleeding. Prostate cancer tissue is probably also a better site of degradation for hemorrhage than normal prostate tissue.
3.5.2 Diffusion-Weighted Image (DWI)
One of the most important recent advances in prostate cancer imaging is the use of diffusion-weighed imaging technique for characterizing prostate tissues and localizing the prostate cancer. DWI reflects the Brownian molecular motion of water. DWI has become an important part of oncological imaging since most of malignant tumors show different Brownian motion of water comparing normal tissue [107].
Recently, the technological developments of echo-planar imaging (EPI), high-amplitude gradients, multichannel coils, and the introduction of parallel imaging have extended the applications of DWI from neuroimaging to the abdomen and pelvis [108].
On DWI, the prostate cancers show high signal intensity on b values of 800–1000 s/mm2. The sensitivity of the DWI sequence to molecular motion can be modified by changing the “b value” parameter. The b value depends on the amplitude, duration, and time interval between the paired gradients used to generate the DWI sequence [100].
An optimal b value for prostate imaging has not yet been established. Increased b values over 1000 s/mm2 can increase tumor visibility, especially in the transition zone. However, increased b values also lead to an increase in the scan time and more pronounced image distortion artifacts. In 2012, the European Society of Urogenital Radiology (ESUR) suggests the use of the b values of 0, 100, and 800–1000 s/mm2 [57].
The signal intensity on the DWI images reflects the amount of restriction of water to diffusion but also the T2-weighted characteristics of the tissue, which is the so-called “T2 shine through” effect. Apparent diffusion coefficient (ADC) can be calculated from the diffusion-weighted image data. The ADC map is obtained by using data from DWI trace images with at least two different b values and does not reflect T2W characteristics.
In the ADC maps, areas with diffusion restriction such as cancer show low signal intensity. The ADC levels are significantly lower in prostate cancer tissue than in noncancerous prostate, which enables the localization of prostate cancer [109]. Prostate cancer demonstrates high signal intensity on the DWI at high b values and has low signal intensity on the ADC map (Fig. 3.19). For detecting prostate cancer on diffusion-weighted imaging study, both DWI and the ADC map should be assessed because some tumors are diagnosed only on the ADC maps, and others are visible only on the DWI images [110]. Most studies showed that DWI is a very useful and it can increase sensitivity by 10–25 % in the diagnosis of prostate cancer [111–113]. From the point of view about technical aspect, to optimize the discrimination of prostate cancer from benign tissue using ADC, Kim et al. and Metens et al. suggested b values of 1000 to 1500 s/mm2 to be the most helpful [114, 115].


Fig. 3.19
Apical prostate cancer seen on diffusion-weighted imaging. (a) T2-weighted axial scan shows irregular marginated low signal intensity lesion surrounding prostatic urethra (arrows). (b) Apparent diffusion coefficient (ADC) map image shows signal drops of prostate apex (arrows). This lesion was confirmed as prostate cancer after prostatectomy
Because ADC values are lower in BPH, usually located in transition zone, than in the peripheral zone, the accuracy for detection of prostate cancer in the transition zone is significantly lower and even lower in the prostate base [116]. And DWI must be evaluated together with T2-weighted imaging especially when assessing the central portions of the prostate gland to avoid confusing the BPH and prostate cancer [112].
Several studies showed that DWI can also reflect the information regarding the aggressiveness of prostate cancer. They have shown a correlation between the ADC and the Gleason score [117, 118]. ADC value in cancer has a negative correlation with the Gleason score, and low ADC values were seen primarily in high-grade aggressive prostate cancers [111, 119]. Some researchers reported that DWI may play a potential role in differentiating low-grade tumor with Gleason score of 6 from high-grade tumor with Gleason score of more than 7 (4 + 3) [117, 120, 121].
In conclusion, DWI is a fast and simple technique in the point of not only increasing accuracy in detection but also providing additional quantitative information that correlates with aggressiveness of prostate cancer.
3.5.3 Dynamic Contrast-Enhanced MRI
Dynamic contrast-enhanced (DCE) MRI is a fast T1-weighted imaging technique that dynamically measures a bolus pass of an intravenously administrated MR contrast agent through the prostate and cancer [13]. The goal of DCE imaging is to capture the passage of contrast material into and out of the prostate using T1WI with high temporal resolutions. In DCE acquisition, the temporal resolution is fast enough to capture the rapid increase of signal within the first 30 s of contrast administration.
Contrast-enhanced MRI sequences can be used to assess the vascularity and permeability of tissues. Usually fast T1-weighted gradient echo sequences with a temporal resolution of 4–10 s are used in prostate imaging [57]. Prostate cancers are characterized by early washin (early peak enhancement) and early washout compared to normal prostate tissue [122].
For its nutrient and oxygen supply, a tumor rapidly forms new networks of tumor vessels named of neo-angiogenesis. In tumor tissue, these vessels made from tumor angiogenesis are often leaky or incomplete, which makes it easier for a contrast agent to extravasate into the extravascular extracellular space. In this extracellular space, the gadolinium-based contrast agent increases the signal intensity of T1-weighted images.
DCE-MRI measures the time-intensity curves passing through the prostate by repeatedly acquiring T1-weighted images at high temporal resolution with the order of seconds (Fig. 3.20). These time-intensity curves can be described semiquantitatively or modeled into pharmacokinetic parameters. The parameters of semiquantitative measurement include the start of enhancement, washin gradient, maximum enhancement, time to peak, washout gradient, and area under the gadolinium curve. And the pharmacokinetic parameters after the fitting to a pharmacokinetic model include forward leakage rate, washout rate constant, and leakage space [123].


Fig. 3.20
Dynamic contrast-enhanced (DCE) MRI of prostate cancer. DCE images show early enhancing lesion in apex (arrows) comparing contralateral portion
For example, on the basis of enhancement curves, gadolinium concentrations in tissue and tissue transport constants in the direction of the tumor interstitium (Ktrans) and back in the direction of the blood plasma (Kep) can be calculated using two-compartment Tofts model [124].
For an accurate assessment of the model parameters, an arterial input function is needed that describes the shape of the contrast bolus arriving at the prostate. Tumor tissue of the prostate is characterized by increased pharmacokinetic parameters compared with healthy tissue.
DCE-MRI has been shown to be of use in detection and staging of prostate cancer within a multiparametric protocol [97, 125, 126] and is especially useful in follow-up after systemic treatment [127, 128]. Even though the currently available studies do not provide a clear conclusion regarding the improvement of prostate cancer detection via DCE, some studies were able to show an improvement of the diagnostic accuracy of conventional MRI (T2-weighted and T1-weighted images) when supplemented by DCE imaging [129, 130].
As with other imaging technologies, DCE also has a problem in differentiating hyperplastic nodules in BPH from prostate cancer. Because BPH nodules can enhance and wash out quickly like prostate cancer, Ktrans and Kep can be increased and limit the sensitivity and specificity of DCE in diagnosing prostate cancer [131]. In addition, inflammation can often have greater vascularity and tissue permeability, which confuse the diagnosis of prostate cancer.
3.5.4 MR Spectroscopy
MR spectroscopy (MRS) analyzes the chemical substances in an organ. The normal prostate gland produces citrate-containing secretion, resulting in high citrate content and a low choline level. A high concentration of citrate is unique to the prostate, and it plays an important role in normal physiological function of the gland [30]. Measured in parts per million (ppm), citrate, creatine, and choline-containing compounds appear at 3.2, 3.0, and 2.6 ppm with respect to the water resonance at 4.7 ppm [93]. In prostate cancer, the choline level is significantly elevated, and the citrate content is reduced due to the metaplastic processes of the cell membranes (Fig. 3.21). Increased concentrations of choline-containing metabolites are related to an increased cell turnover and have also been associated with the presence and progression of prostate cancer [133]. The relationship between these two metabolites can be used as a measure of malignancy [134]. At common clinical field strengths, even though it is difficult to differentiate choline peaks from creatine peaks on the spectra, the ratio of choline plus creatine to citrate is used as a metabolic biomarker for prostate cancer [135].


Fig. 3.21
MR spectroscopy (MRS) of prostate cancer. Note that the choline peak is increased in the box area (arrows). The lesion was confirmed as prostate cancer (Gleason score, 7) after prostatectomy (Courtesy of prof. Chan Kyo Kim, Samsung Medical Center)
MRS increases the diagnostic accuracy of the morphological MRI examination from 52 to 75 % [136]. The European Society of Urogenital Radiology (ESUR) suggested Prostate MR Guidelines 2012 using either a qualitative or a quantitative approach for interpreting prostate MRS. The qualitative approach is based on visual analysis of the citrate and choline/creatine peaks. If the choline/creatine peak is higher than the citrate peak in at least three adjacent voxels, prostate cancer is suspected. In the quantitative approach, the areas of the peaks are measured, and choline plus creatine-to-citrate ratios higher than 0.72 in at least two adjacent voxels are considered to indicate malignant tissue, whereas ratios between 0.58 and 0.72 are considered ambiguous [57].
For the good spectral resolution, a homogeneous magnetic field and sufficient suppression of the fat and water signal during the measurement is needed in MRS measurements. In addition to sequence adjustments, MRS usually requires multiple shimming steps and saturation bands around the prostate for optimal information. Evaluation and interpretation of MRS are very complex and often only possible after appropriate physical adjustments. Because of these technical requirements, complexity of interpretation, and significant time investment, MR spectroscopy will remain limited in its daily use.
3.5.5 Multiparametric MRI
Multiparametric MRI incorporates several different imaging modalities including T2WI, DWI, and DCE to assess potential lesions in the prostate (Fig. 3.22). In prostate MR imaging, no single technique is able to adequately detect and characterize prostate cancer. T2-weighted image shows the best tissue contrast for the detection, localization, and staging of prostate cancer, which has shorter T2 comparing normal prostate tissues. However, other process such as prostatitis or BPH can also show T2 shortening. Although the image quality is not so good as T2-weighted image, DWI provides the information regarding Brownian motion of water molecules. Because free water motion is generally restricted within cancer tissue, DWI is an essential component of mpMRI (Fig. 3.22).


Fig. 3.22
Multiparametric MR images of prostate cancer. T2-weighted (a), Kep map (b), Ktrans map (c), and ADC map (d) images show focal abnormal lesion on right peripheral zone of prostate (arrows). This patient underwent radical prostatectomy and confirmed as prostate cancer with Gleason score of 7 (Courtesy of prof. Chan Kyo Kim, Samsung Medical Center)
Modern MRI studies often incorporate a combination of the several MR techniques including T2-weighted imaging, DWI, DCE, and MRS. The combination of anatomic T2W images and the functional techniques has been shown to increase the predictive power of MRI for detection and staging of prostate cancer [93]. ESUR prostate MR guideline suggests the use of T2W images plus two functional techniques [137].
There are several reports about the clinical results of mpMRI. Tanimoto et al. reported a significant increase in the area under the receiver operating curve (AUC) for the detection of prostate cancer as they evaluated a protocol with T2W images only (AUC = 0.711), a protocol combining T2W imaging and DWI (AUC = 0.905), and finally a more complete protocol including T2W imaging, DWI, and DCE (AUC = 0.966) [138]. Turkbey and colleagues also reported a higher sensitivity for the combination of T2W images, MRS, and DCEI than for each sequence alone [139].
The advantages of mpMRI are achieved by combining the information obtained with the various techniques. Software for the evaluation of two or more multiparametric images in one view is developed for the integrated interpretation of anatomic and functional findings. In addition, supportive techniques such as computer-aided diagnosis are needed to achieve fast and reliable diagnosis from large complex data [140–142]. For the more common usage of mpMRI, the education, experience, and dedications of radiologists are essential [143].
3.5.6 MR PI-RADS
In 2012, the ESUR created the magnetic resonance prostate imaging reporting and data system (MR PI-RADS) as part of its MRI guidelines for prostate imaging [57]. They suggested a standardized method for reporting mpMRI of the prostate for the detection of prostate cancer.
Based on criteria according to PI-RADS, every lesion suspicious for tumor within the prostate is assigned a point value between 1 and 5 for mpMRI, usually consisting of T2W, DWI, and DCE. A value 1 means that a lesion is probably benign, while a value 5 indicates a high probability of malignancy. Total point value is calculated for every lesion suspicious for tumor [144]. Thus, a probability statement regarding the presence of a clinically significant prostate cancer should be possible. Many studies reported good and reproducible diagnosis accuracy [145–147]. In 2015, they revised PI-RADS to PI-RADS v2. The difference point of PI-RADS v1 and v2 is that the primary determining sequence is different according to the zone in v2. According to PI-RADS v2, for the peripheral zone, DWI is the primary determining sequence; on the contrary, for the transition zone, T2-weighted image is the primary determining sequence.
3.5.7 MRI-Guided Biopsy
If patients have negative results after TRUS-guided biopsy with clinically suspicion of prostate cancer, MRI can be used to detect suspicious areas to be targeted during TRUS-guided biopsies. Three different methods are suggested in MR-guided biopsy. The first one is to use fusion software for MRI and TRUS images during TRUS-guided biopsy procedure, which is the most common method using MRI. The second one is direct targeting in the magnet using MRI compatible devices (Fig. 3.23). The last one is cognitive targeting in which the operator reviews the MRI images before TRUS-guided prostate biopsy and tries to target the suspected area during the TRUS-guided biopsy [10].


Fig. 3.23
MR-guided biopsy using MR-compatible device in the magnet. MR compatible endorectal device is inserted into the rectum, and the location of the needle can be confirmed in MR magnet (arrows) (Courtesy of Prof. Chan Kyo Kim, Samsung Medical Center)
Many literatures about image-guided biopsy of prostate using MRI images revealed that most studies reported superior performance than random systematic biopsies [100]. Haffner et al. reported a higher diagnostic accuracy for the detection of significant cancer than random systematic biopsies when they used cognitively targeted biopsies (0.98 vs. 0.88, respectively) [148].
In random systematic biopsies, the tumors located at low apical and anterior prostate are difficult to detect. Anteriorly located prostate tumors account for about 21 % of all prostate cancers and are more often missed on systematic biopsies [149]. A study using mpMRI-targeted biopsy revealed that the accuracy of prostate biopsy on anterior tumors was about 98 %, comparing 46 % in random systematic biopsies [150]. Clinically, under the situation of elevated PSA and a negative biopsy, the option to perform the repeat biopsy using direct or indirect MRI-guiding can be suggested.
3.5.8 MRI for Active Surveillance
Active surveillance is defined as the initial expectant management, with close follow-up and selective delayed intervention for the subset of patients reclassified over time as at higher risk for progression, based on clinical, pathological, or molecular parameters [151]. The criteria of active surveillance include Gleason ≤6, PSA <10 ng/ml, and fewer than three positive biopsies. All core biopsies should not contain more than 50 % cancer involvement. mpMRI can play a significant role in identifying suitable patients [152].
Recently, as alternatives to the prostatectomy, a number of minimally invasive, focal, organ-preserving methods have been introduced. These methods include focal tumor ablation using cryoablation, high intensity-focused ultrasound (HIFU), or laser-induced ablation [153]. The main advantages of these treatments are to avoid postoperative complications such as incontinence and impotence. Advanced imaging technologies including mpMRI made it possible to determine the exact location of relevant tumor foci and to guide focal therapies [153].
3.5.9 MR Imaging of Treated Prostate Cancer
The treatment options of prostate cancer include radical prostatectomy, radiation therapy, androgen-deprivation therapy, or focal therapy such as cryotherapy or HIFU. Radical prostatectomy has been performed for more than a century and the most common treatment choice in patients with organ-confined disease [154]. MRI after radical prostatectomy reveals low signal intensity at anastomotic site. These lesions suggest postoperative scarring. Sometimes the remained seminal vesicles are observed in about 20 % of patients after prostatectomy [155]. The presence of soft tissue showing iso-signal intensity on T1-weighted image and slightly hyperintense signal intensity on T2-weighted image at prostatectomy site strongly suggests the tumor recurrence. The most common site of prostate cancer recurrence is around vesicourethral anastomosis around urinary bladder and membranous urethra [127]. In most cases, it is possible to differentiate the tumor recurrence at anastomotic site and postoperative fibrosis. On DCE-MRI, recurrent tumor tends to early enhancement and early washout pattern, while postoperative changes tend to show no enhancement or mild enhancement in the venous phase (Fig. 3.24).


Fig. 3.24
Prostate cancer recurrence after prostatectomy. (a) T2-weighted axial scan shows slightly high signal intensity lesion at anastomotic site (arrows). (b) Post-contrast T1-weighted image shows well-enhancing mass-like lesion at anastomotic site (arrows). (c) ADC map shows decreased signal intensity lesion at anastomotic site. TRUS-guided biopsy revealed that this lesion was prostate cancer with Gleason score of 9
Radiation therapy is another option alternative to prostatectomy. It is known that about 25 % of patients with prostate cancer undergo radiation therapy [156]. Three-dimensional conformal RT is introduced [157]. The computer modifies the radiation beams to focus the region of the prostate. Intensity-modulated radiation therapy is one of the most sophisticated types of radiation therapy. Brachytherapy, in which radioactive seeds or needles are implanted directly into the prostate gland and sometimes into the surrounding tissues, is one of radiation therapy. After radiation therapy, MR study reveals that the entire prostate and seminal vesicles show marked decreased size and low signal intensity on T1- and T2-weighted images. The whole prostate, including central zone, transitional zone, and peripheral zone, is not distinct and shows low signal intensities. The recurrent tumor after radiation therapy typically shows a nodular lesion of lower signal intensity than adjacent normal prostate [158]. Restricted diffusion on DWI or rapid enhancement on DCE-MRI suggests the presence of tumor recurrence [158].
Androgen deprivation therapy has been used in patients with metastasis, increasing PSA levels after local treatment or advanced disease. Androgen deprivation therapy can be performed surgically or pharmacologically [159]. The MRI findings of after-androgen-deprivation therapy are similar to those after radiation therapy. The size and the signal intensity of prostate are decreased (Fig. 3.25). However, the radiation therapy-induced changes in adjacent organs are not definite [158].


Fig. 3.25
Prostate MR imaging after hormone therapy. This 85-year-old man underwent hormone therapy for 1 year since he was diagnosed as prostate cancer. (a) T2-weighted axial scan shows atrophic changes of prostate. The signal intensity of prostate is low regardless of the zone. (b) The seminal vesicle also shows atrophic changes and obliteration of seminal vesicle lumen (arrows)
Especially, MRI plays an important role not only in localized prostate cancer but also in evaluating the recurrence after prostatectomy, radiation therapy, or androgen-deprivation therapy.
3.5.10 Hyperpolarized MRI
Hyperpolarized 13C MRI is a new technique that enhances signal tens of thousandfold [160]. For 13C imaging, the polarization mixture typically contains the 13C-enriched substrate, free radical, gadolinium, and glassing material. Chen et al. reported their initial experience about hyperpolarized 13C metabolic imaging of mice with transgenic adenocarcinoma of the prostate (TRAMP) using hyperpolarized 13C-pyruvate. They found that highly elevated lactate signal in later-stage prostate tumors. In case of advanced prostate cancer, the level of lactate signal is increased with tumor progression and correlated with histologic grade [160].
3.5.11 The Future of Prostate MR
There are a number of ongoing technical developments that may have an impact on the evaluation of prostate. The development of ultrahigh-field MR systems such as 7 T showed the possibilities for potentially higher spatial and spectral resolution [137], diffusion tensor imaging (DTI) [161], and multi-b-factor approaches to access multicomponent diffusion information. Hyperpolarized 13C MRI showed the potentials for direct observation and measurement of dynamics within the biochemical pathways in normal and abnormal prostate metabolism [162]. MR elastography that combines the principles of ultrasound elasticity and MRI to make measures of the “elasticity or stiffness” of prostate tissues has been demonstrated [163, 164]. Even though these techniques have technical challenges, they show potentials for exact localization of the prostate cancer by advanced techniques and for getting the representative prostate tissue by advanced image-guided biopsy techniques.
3.6 PET-CT
PET/CT is a widely used molecular imaging modality. 18F-FDG, glucose metabolism targeting radiotracer, is the main radiotracer for oncologic study. Enhanced glucose metabolism, Warburg effect, is one of the hallmarks in cancer [165], though it is not a cancer-specific phenomenon. The clinical utility of oncology PET using FDG has been proven in staging and restaging of malignant tumors such as the head and neck, lung, breast, and colorectal cancers, malignant lymphoma, and melanoma [166]. FDG-PET is capable of visualizing malignant tumors and associated lymph nodes and distal metastatic sites in a single test. However, 18F-FDG-PET has a limited role in primary diagnosis and staging of the prostate cancer due to not only overlap accumulation of normal and abnormal prostate tissue but also the intrinsic metabolic alteration of prostate cancer itself [167]. There is limited data on the use of FDG-PET/CT in initial staging of prostate cancer because of the general low avidity of FDG for the primary prostate cancer [168]. But, FDG-PET is not generally recommended in the diagnosis or staging of clinically organ-confined disease due to overlap of uptake among normal, benign, and tumor tissues and the high excreted radiotracer in the adjacent urinary bladder [169]. However, FDG-PET may be useful in patients with suspected poorly differentiated primary tumors (Gleason sum score above 7) and higher serum PSA level [170] (Figs. 3.1 and 3.26). In the early analysis of the National Oncologic PET Registry (NOPR) data in the Unites States for initial staging of prostate cancer, FDG-PET/CT had an impact on clinical management in 32 % (95 % CI: 30.0–34.1 %) [171].


Fig. 3.26
FDG-PET has a limited role in detecting malignant tumors of the prostate. However, it may be helpful in patients with suspected poorly differentiated prostate cancer and higher serum PSA levels. A 64-year-old man presented with nocturia/hematuria and elevated serum PSA level of 19.7 ng/mL. FDG-PET/CT (a) shows focal hypermetabolism (3.7 of SUVmax, arrow) corresponding the lesion of low signal intensity (arrow) on T2-weighted MRI (b). Prostate adenocarcinoma was confirmed on pathology with Gleason score of 7 (4 + 3)
In recent years, lipogenesis targeting radiotracer, such as 11C-choline or 11C-acetate upregulated in malignant cells, has shown potential usefulness in brain, prostate, and esophageal cancers because of enhanced synthesis of membrane phospholipids [172]. Both 11C-choline and 18F-fluorocholine (FCH) have been studied for prostate cancer. 11C-choline has the advantage of low excreted urine activity. 18F-fluorocholine has the advantage of longer half-life that facilitates regional tracer distribution without the need of on-site cyclotron and radiochemistry facilities [173]. 18F-FCH PET/CT reveals promising but limited evidence for primary prostate cancer staging and has been known to be useful for detection of locoregional and distant metastasis of recurred prostate cancer [172, 174] (Fig. 3.27). A systematic review and meta-analysis by Umbehr et al. of 11C-choline and 18F-fluorocholine PET for the initial staging of prostate cancer reported a pooled sensitivity and specificity of 84 % and 79 % on a per-patient basis (10 studies, n = 637 patients, respectively) [175, 176]. The uptake of choline tracers in prostate cancer is higher than that of prostate hyperplasia, but there is still overlap between the prostate cancer and benign disease [177].


Fig. 3.27
A 84-year-old patient performed 18F-FCH PET/CT in suspicion of prostate cancer recurrence. Serum prostate-specific antigen (PSA) level increased from 2.3 to 57.3 ng/ml. On 18F-FCH PET/CT, paraaortic lymph nodes metastasis (a, b, arrow) and T8 spine metastasis (c, d, arrow) are detected
Other kinds of molecular imaging targets have been proposed in order for more specific to prostate cancer. Anti-1-amino-3-18F-fluorocyclobutane-1-carboxylic acid (anti-18F-FACBC) is a synthetic non-metabolized amino acid analog that accumulates in prostate cancer via overexpression of the ASC (alanine, serine, and cysteine) transport system and other amino acid transport systems [178]. 18F-fluoro-5α-dihydrotestosterone (18F-FDHT) is a labeled analog targeting the androgen receptor, which plays an important role in the development, growth, and maintenance of the prostate gland [179]. PSMA is a type II cell surface transmembrane glycoprotein (also known as folate hydrolase I or glutamate carboxypeptidase II) upregulated in prostate cancer, providing a promising molecular target for diagnostic imaging and directed therapy labeled with radionuclide such as 177Lu or 90Y [180].
Another approach is to use the multimodal, multiparametric imaging for the prostate cancer. Simultaneous PET/MRI has been developed lately and expected to be a better diagnostic imaging modality by combining functional, molecular, and morphological information. Moreover, it is expected to be valuable than PET/CT in the aspect of one-stop imaging with low radiation exposure and good soft tissue contrast resolution [181]. Combined PET/MR imaging could facilitate the use of MRI-assisted metabolic parameters or PET-guided multiparametric imaging analysis for the precise identification of targeted lesions and appropriate method for diagnosis and therapy planning of the primary prostate cancer [182] (Fig. 3.28). Simultaneous acquisition of multiparametric MR and PET images with an appropriate radiotracer may be particularly valuable for identifying high-yield candidate biopsy sites that could reduce false-negative initial and repeated biopsies [183, 184].


Fig. 3.28
A 77-year-old patient performed 18F-FCH PET/MRI for initial staging of prostate cancer. Axial T2-weighted MRI (a) shows low signal intensity (arrow) in the prostate cancer, with diffusion restriction (arrow) on the ADC map (b). 18F-FCH PET (c) and PET/MRI (d) demonstrated concordant results (arrow) with a possible application of MRI-assisted metabolic-volumetric PET quantification
In summary, FDG-PET has been evaluated with promising and disappointing results in the field of urologic cancer [166, 168]. PET as molecular imaging and PET/MRI with dual tracers and multiparametric approaches are challenging fields to be applicable to all clinical phases of prostate cancer with the concept of precision and personalized medicine [173, 182]. Additional potential applications may include not only the assessment but also the deciphering of new insights into the biologic changes induced by various novel therapies [176].
3.7 Pathology of Prostate Cancer
3.7.1 Acinar Adenocarcinoma
3.7.1.1 Macroscopy
Grossly prostatic adenocarcinoma is a hard, yellow, or whitish nodule (Fig. 3.29). But frequently, prostatic adenocarcinoma is not distinguishable grossly, and tumor border is also not evident. Prostatic acinar adenocarcinoma mainly arises in the peripheral zone (posterior and posterolateral side) and is frequently multifocal [185–187].


Fig. 3.29
On the cut surface of prostatectomy specimen, a vague yellow to whitish nodule (arrow) is found in the left lobe. Grossly prostatic adenocarcinomas are frequently ill-defined
3.7.1.2 Microscopy
Microscopic appearance of acinar adenocarcinomas varies according to their differentiation. Well-differentiated tumors show aggregation of well-formed small glands with infiltration between benign glands (Fig. 3.30a). Poorly differentiated tumors reveal poorly formed glands, glandular fusion, cribriform glands, single cells, cords, or solid tumor nests (Fig. 3.30b).


Fig. 3.30
(a) Infiltrations of small well-formed carcinoma glands (arrows) between normal glands are found in well-differentiated adenocarcinoma. (b) Poorly differentiated adenocarcinoma shows cribriform glands
Two differences between malignant and benign prostatic glands help the pathological diagnosis of prostatic adenocarcinoma. First, malignant glands have prominent nucleoli. Second, basal cells are not present in malignant glands (Fig. 3.31a). Loss of basal cells is readily identifiable by immunohistochemical staining for basal cells. High-molecular-weight cytokeratin and p63 are widely used for basal cell marker (Fig. 3.31b) [188, 189].


Fig. 3.31
(a) Malignant glands of prostate show prominent nucleoli and loss of basal cells. (b) Immunohistochemical staining for basal cell markers (high molecular weight cytokeratin and p63 cocktail) shows loss of basal cells in carcinoma glands (arrows). Adjacent normal glands have basal cells positively stained with basal cell markers
3.7.1.3 Gleason Grading
The Gleason grading system is widely used grading system of prostatic adenocarcinoma. It was firstly developed by Gleason in 1966 [190, 191]. This grading system described five basic architectural patterns of carcinoma glands, and each pattern is scored from 1 to 5. In resection specimen, the Gleason grade is defined as the sum of most common and second common patterns, and this sum is reported as Gleason score [192].
This grading system has been modified several times. Recently, International Society of Urological Pathology (ISUP) 2005 consensus conference proposed some modification in Gleason grading system. This modification includes criteria of Gleason pattern, grading of variants of prostatic adenocarcinoma, and reporting of secondary and tertiary pattern.
According to the 2005 ISUP modified Gleason system [192], Gleason patterns are defined as below:
Pattern 1 is defined as a well-circumscribed nodule of closely packed, well-formed, medium-sized, round to oval glands. The glands are usually larger than pattern 3, and there is no infiltration into adjacent benign glands. This pattern is very rare. Pattern 2 is defined as fairly circumscribed nodule with minimal infiltration (Fig. 3.32a). The tumor glands shows more size variation and are more loosely arranged than pattern 1. Pattern 3 is defined as well-formed individually discrete glands with infiltration between benign glands (Fig. 3.32b). More size variation is present than pattern 1 or 2. Small cribriform glands with smooth margin are also included in this pattern. Pattern 3 is the most common pattern. Pattern 4 includes fused microacinar glands (Fig. 3.32c), ill-defined glands with inconspicuous lumina (Fig. 3.32d), and large cribriform glands (Fig. 3.32e). Pattern 5 is defined as tumors with no glandular differentiation. It consists of solid sheets (Fig. 3.32f), cords, or aggregation of single cells (Fig. 3.32g). Cribriform pattern with central comedonecrosis is also included in pattern 5 (Fig. 3.32h).


Fig. 3.32
(a) Gleason pattern 2 shows relatively well-circumscribed mass with minimal infiltration and size variation of tumor glands. (b) Gleason pattern 3 shows infiltration of well-formed tumor glands (arrows) between benign glands (arrow heads). Gleason pattern 4 includes (c) fused glands, (d) ill-defined glands with inconspicuous lumina, and (e) cribriform glands. Gleason pattern 5 includes (f) solid growth pattern, (g) tumor cell cords or single cells, and (h) cribriform glands with comedonecrosis
3.7.1.4 Variants of Acinar Adenocarcinoma
Some morphologic variants are present such as atrophic variant (Fig. 3.33a, b), pseudohyperplastic variant (Fig. 3.33c, d), and foamy gland variant (Fig. 3.33e).


Fig. 3.33
Variants of prostatic adenocarcinoma. (a, b) Atrophic variant shows malignant tumor glands with scanty cytoplasm (arrow) (a), and these glands reveal the loss of basal cells in immunohistochemistry for basal cell markers, indicating their malignant feature (b). (c, d) Pseudohyperplastic variant shows large glands with papillary infoldings mimicking benign hyperplastic glands (c), but loss of basal cells in immunohistochemical staining for basal cell markers confirms the diagnosis of adenocarcinoma (d). (e) Foamy gland carcinoma reveals characteristic abundant foamy cytoplasm with relatively small nuclei
3.7.1.5 High-Grade Prostatic Intraepithelial Neoplasia
High-grade prostatic intraepithelial neoplasia consists of atypical cells confined to preexisting prostatic duct or acini (Fig. 3.34a, b). The abnormal cells resemble adenocarcinoma cells frequently showing prominent nucleoli. This lesion has intact basal cell layer, and is frequently found around prostatic adenocarcinoma. High-grade prostatic intraepithelial neoplasia is regarded as a precursor for prostatic adenocarcinoma.


Fig. 3.34
Microscopic findings of high-grade prostatic intraepithelial neoplasia (HGPIN). (a) HGPIN in this photograph shows large glands with papillary infoldings (arrow). The lining cells occasionally show prominent nucleoli. Adenocarcinoma glands are found adjacent HGPIN. (b) Immunohistochemical staining for basal cells shows preserved basal cell layer in HGPIN
3.7.2 Other Carcinomas
3.7.2.1 Ductal Adenocarcinoma
Histologic feature of ductal adenocarcinoma is somewhat different from acinar adenocarcinoma. This tumor shows large papillary or cribriform architecture (Fig. 3.35). The tumor cells are tall columnar cells with pseudostratification. Basal cells are not present. This tumor is classified as Gleason pattern 4.


Fig. 3.35
Ductal adenocarcinoma consists of tall columnar cells with pseudostratification. This case shows cribriform pattern
3.7.2.2 Urothelial Carcinoma
Urothelial carcinoma of prostate is developed in prostatic urethra or periurethral glands. Morphologic features are identical to bladder urothelial carcinoma.
3.7.2.3 Small Cell Carcinoma
Small cell carcinoma can be developed in prostate. Histologic features of small cell carcinoma of prostate are similar to small cell carcinoma of other sites. Microscopically tumor cells have scanty cytoplasm with round nuclei and “salt and pepper” nuclear chromatin pattern (Fig. 3.36). Necrosis is quite common. Tumor cells express the neuroendocrine markers.


Fig. 3.36
Small cell carcinoma of prostate shows identical morphology to small cell carcinoma of other sites
3.8 Staging
The information about the staging is essential because it influences the treatment plans and guessing the prognosis and disease spread. The clinical parameters such as pretreatment PSA level, rate of rise or doubling time, Gleason score, clinical T staging, and volume of disease detected on biopsy are also important in evaluating stages. Imaging modalities commonly used for the prostate include MR, CT, radioisotope bone scan, and positron emission tomography (PET). Local staging about periprostatic invasion is usually performed by digital rectal examination (DRE) and MRI (Fig. 3.37). Regional staging in pelvic area is performed by either CT or MRI. And CT or bone scan are used for the evaluation of distant metastasis, metastatic lymphadenopathy, or bone metastasis (Fig. 3.38). In addition, clinical or laboratory data including PSA level or Gleason score can also yield useful information for evaluating tumor stage.



Fig. 3.37
Prostate cancer with periprostatic invasion. T2-weighted axial image shows irregular contour bulging lesion in left peripheral zone, suggesting periprostatic invasion (arrows)

Fig. 3.38
Prostate cancer with SV invasion. T2-weighted axial scan (a) and coronal scan (b) reveals destruction of seminal vesicle wall and mass-like low signal intensity lesion in left seminal vesicle (arrows). (c) ADC image also reveals signal drop, suggesting diffusion restriction
For the staging of prostate cancer, the evaluation of seminal vesicle seen on MR is very important (Fig. 3.39). For the evaluation of seminal vesicle invasion, MR is the most commonly used modality. Jung et al. suggested six categories according the shape of seminal vesicle. Among six groups, the groups with apparent mass with destructive architecture or focal asymmetric low signal intensity lesion within the seminal vesicle without definite mass showed high positive predictive values [193].


Fig. 3.39
Advanced prostate cancer with multiple metastatic lymphadenopathies. (a) T2-weighted axial scan shows the whole prostate is replaced by low signal intensity lesion (arrows). Also note that the low signal intensity lesion invades the bilateral seminal vesicles, suggesting seminal vesicle invasion (arrowheads). (b) T2-weighted axial scan at bladder level shows irregular-shaped large mass, suggesting conglomerated metastatic lymphadenopathy (arrows). (c) T2-weighted coronal image show irregular-shaped lymphadenopathy (arrows). Also note ill-defined prostate mass, suggesting prostate cancer (arrowheads)
The American Joint Committee on Cancer (AJCC) staging is a widely used staging system in prostate cancer [194]. Stage T1 is clinical staging defined as “clinically inapparent tumor neither palpable nor visible by imaging.” Pathologic stage T2 means tumors confined to prostate. pT3 includes extraprostatic extension (pT3a) (Fig. 3.40a) and seminal vesicle invasion (pT3b) (Fig. 3.40b). pT4 is tumors with invasion of an adjust organ such as the rectum, levator muscle, or pelvic wall.


Fig. 3.40
(a) Extraprostatic extension of prostatic adenocarcinoma (pT3a) is defined as infiltration into soft tissues beyond fat plane (arrow). (b) Prostatic adenocarcinoma with cribriform pattern invades into the seminal vesicle (pT3b)
3.9 Treatment of Prostate Cancer
3.9.1 Active Surveillance
3.9.1.1 Introduction
Widespread prostate cancer screening, which uses DRE and PSA, has led to increase the detection of low-volume, low-grade disease, namely, clinically insignificant prostate cancer (CIPC) [195]. Immediate definitive treatment for CIPC may give rise to unnecessary complications related to treatment. In the light of these points of view, active surveillance (AS) can redeem the problems of overdiagnosis and overtreatment resulting from early diagnosis of prostate cancer [196]. Therefore, AS has been universally considered to be a therapeutic modality in low-risk-localized prostate cancer. Given that active intervention is performed if there is clear evidence of progression of the cancer during observation period, AS is a distinct therapeutic modality from previous expectant management or watchful waiting [197]. In other words, the objective of AS is to conduct a definitive therapy with curative intent in patients who show clear signs of cancer progression and to reduce treatment-related complications in patients with a low possibility of progression during observation period.
3.9.1.2 Definition of CIPC
CIPC generally refers to “low-risk” prostate cancer. Many physicians and patients take into account AS for selected low-risk prostate cancers. In fact, one of the major controversies in AS may be a definition of the CIPC [198]. The criteria stratifying the risk of prostate cancer have been suggested by several investigators (Table 3.1). However, caution should be taken when assessing the CIPC using these criteria. In several studies utilizing the postoperative pathologic findings, it was reported that approximately 8 % of prostate cancers which were classified as CIPC on the basis of Epstein criteria were not localized within the prostate [199, 200]. The consensus, which early detected CIPC as a result of serum PSA testing has no significant effect on clinical outcomes of the patients with life expectancy of less than 20 years, has been reached among NCCN guideline panels [201].
Table 3.1
Definition of insignificant prostate cancer
Epstein criteria for CIPC | D’Amico low-risk prostate cancer [203] | NCCN risk stratification [201] |
---|---|---|
1. Clinical stage T1c 2. Gleason score ≤6 3. A third or less core biopsies positive 4. 50 % or less involvement of any 1 core 5. PSA density <0.15 ng/ml/g | 1. Gleason score <6 2. PSA <10 ng/ml 3. Clinical stage T1 | 1. Very low risk (a) Clinical T1c (b) Gleason score ≤6 (c) PSA <10 ng/ml (d) Fewer than 3 prostate core biopsy positive, ≤50 % cancer in any core (e) PSA density <0.15 ng/ml/g 2. Low risk (a) Clinical T1-T2a (b) Gleason score ≤6 (c) PSA <10 ng/ml |
3.9.1.3 Selection of Patients and Triggers for Intervention in AS
There have not been standardized criteria for reclassification of patients requiring definitive treatment during AS period, and different criteria among institutions have been applied. The examples of various AS protocols are summarized in Table 3.2. Generally, the definition for disease progression during follow-up period is determined on the basis of serum PSA value (i.e., PSA doubling time (PSADT) of 3 years or less) and histologic (i.e., Gleason sum of 4 + 3 or higher on repeat biopsy of the prostate) and clinical evidence of disease progression (i.e., more than a doubling of the product of the maximum perpendicular diameters of the primary lesion as measured by DRE, local progression of prostate cancer requiring transurethral resection of the prostate, development of ureteral obstruction, or radiologic or clinical evidence of distant metastasis) [204–208]. NCCN has recommended an initiation of curative treatment in case of patients with Gleason grade 4 or 5, increased tumor number, or volume on repeat biopsy, PSADT <3years [201].
Table 3.2
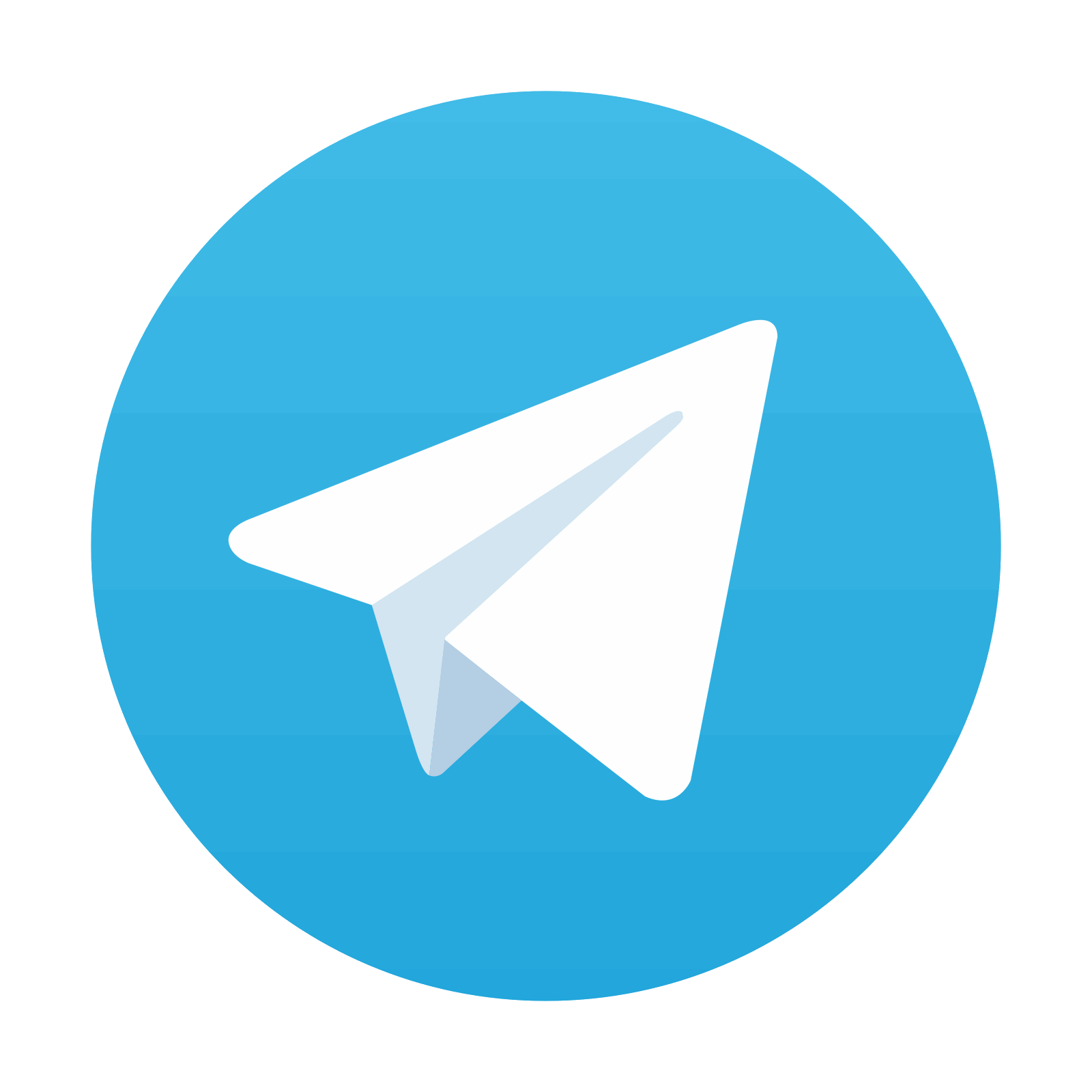
Various active surveillance protocols
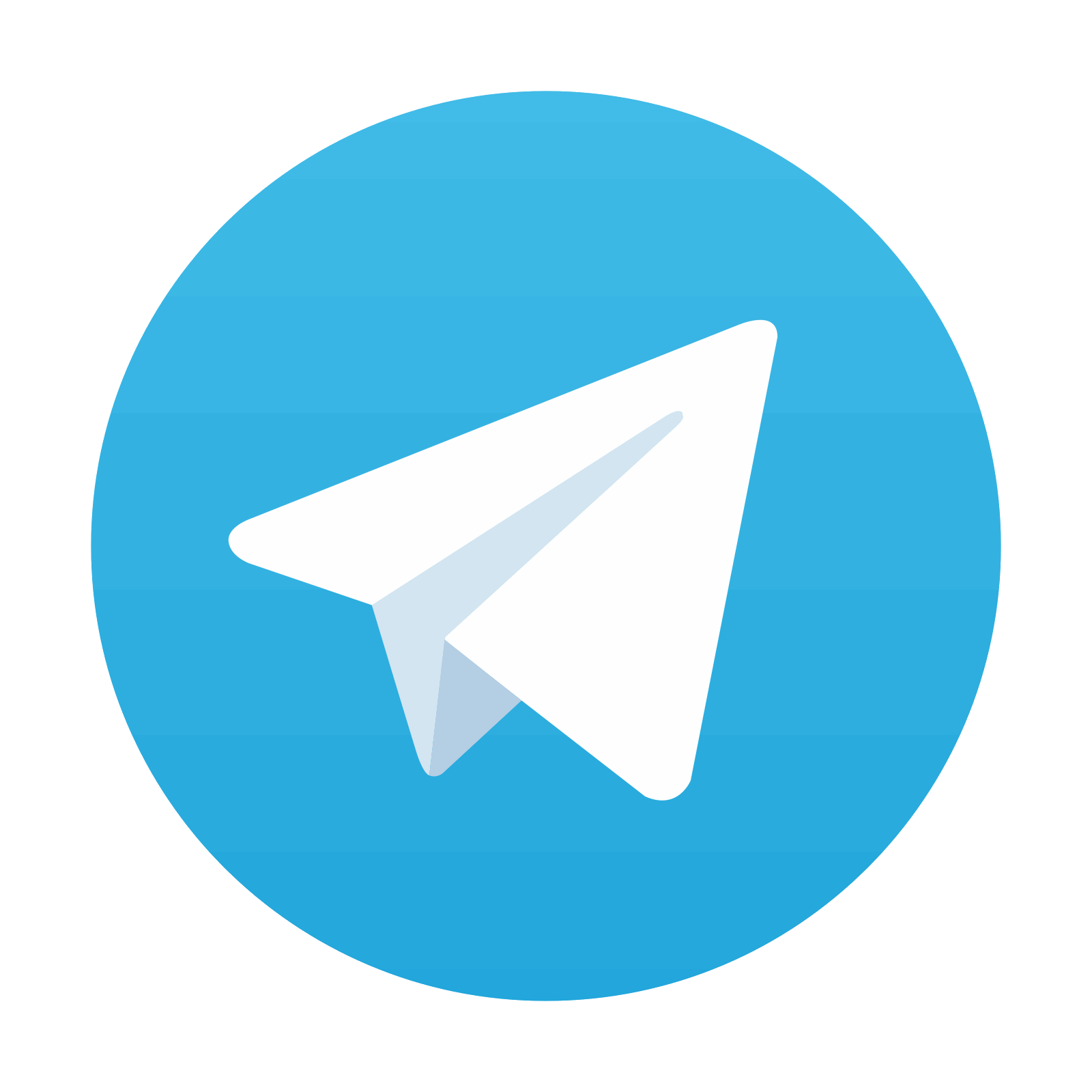
Stay updated, free articles. Join our Telegram channel

Full access? Get Clinical Tree
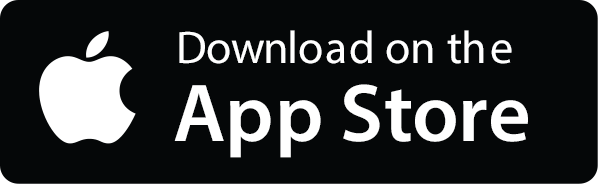
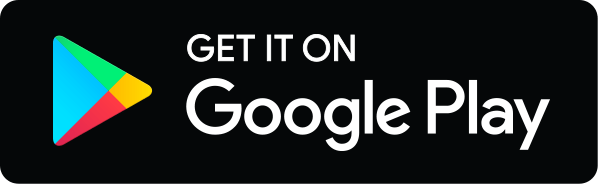
