Obesity-related disorders derive from a combination of genetic susceptibility and environmental factors. Recent evidence supports the role of gut microbiota in the pathogenesis of obesity, type 2 diabetes mellitus, and insulin resistance by increasing energy harvest from diet and by inducing chronic, low-grade inflammation. Several studies describe characteristic differences between composition and activity of gut microbiota of lean individuals and those with obesity. Despite this evidence, some pathophysiological mechanisms remain to be clarified. This article discusses mechanisms connecting gut microbiota to obesity and fat storage and the potential therapeutic role of probiotics and prebiotics.
Key Points
- •
Increased consumption of foods with high energy is involved in obesity development, which is a well-known risk factor for type 2 diabetes mellitus (T2DM) and cardiovascular disease.
- •
Several studies have demonstrated that gut microbiota can modulate host energy homeostasis and adiposity through different mechanisms: energy harvest from diet, fat storage and expenditure, incretins secretion, and systemic inflammation.
- •
Although experimental data suggest gut microbiota manipulation with probiotics and prebiotics can beneficially affect host adiposity and glucose metabolism, their effects are transient and diminish gradually after cessation.
- •
This review analyzes the potential gut microbiota-driven pathways that could represent novel target for treatment of obesity.
Introduction
Obesity-related disorders are related to energy homeostasis and inflammation; gut microbiota are involved in several host metabolic functions and may play an important role in this context through several mechanisms: increased energy harvest from the diet, regulation of host metabolism, and modulation of inflammation.
Human gut flora comprises at least 10 14 bacteria belonging to 3 bacterial phyla: the gram-positive Firmicutes and Actinobacteria and the gram-negative Bacteroidetes. Firmicutes is the largest bacterial phylum and comprises more than 200 genera, including Lactobacillus, Mycoplasma, Bacillus , and Clostridium species. Although each subject has a specific gut microbiota, a core human gut microbiome is shared among family members despite different environments ; nevertheless, the microbiome dynamically changes in response to some factors, including dietary nutrients, illness, and antibiotic use.
This review discusses the interaction of gut microbiota with host metabolism and the impact of manipulating microbiota composition on the pathogenesis and the treatment of obesity.
Introduction
Obesity-related disorders are related to energy homeostasis and inflammation; gut microbiota are involved in several host metabolic functions and may play an important role in this context through several mechanisms: increased energy harvest from the diet, regulation of host metabolism, and modulation of inflammation.
Human gut flora comprises at least 10 14 bacteria belonging to 3 bacterial phyla: the gram-positive Firmicutes and Actinobacteria and the gram-negative Bacteroidetes. Firmicutes is the largest bacterial phylum and comprises more than 200 genera, including Lactobacillus, Mycoplasma, Bacillus , and Clostridium species. Although each subject has a specific gut microbiota, a core human gut microbiome is shared among family members despite different environments ; nevertheless, the microbiome dynamically changes in response to some factors, including dietary nutrients, illness, and antibiotic use.
This review discusses the interaction of gut microbiota with host metabolism and the impact of manipulating microbiota composition on the pathogenesis and the treatment of obesity.
Association between gut microbiota and obesity: pathophysiological mechanisms
Several data suggest that gut microflora play a role in the regulation of host energy homeostasis ( Table 1 ).
Mechanisms | Mediators | Metabolic Effects |
---|---|---|
Reduced intestinal transit rate | Production of SCFA , that increase Gpr41-/Gpr43-mediated PYY secretion | Increased energy harvest from the diet |
Polysaccharide degradation to monosaccharides | Microbial transport proteins and enzymes | Increased CHO absobtion and portal flow |
Increased glucose absorption | Increased intestinal Glut1 expression | |
Increased monosaccharides portal low | Increased capillaries density in intestinal villi | |
Increased de novo lipogenesis | ChREBP and SREBP-1 mediated expression of lipogenic enzyme | Increased hepatic/adipose Tg contents |
Increased adipociyte uptake of circulating FFA | Increased adipose LPL activity through reduction of intestinal Fiaf secretion | |
Reduced FFA oxidation | Reduced Fiaf-induced (PGC)-1α and AMPK-induced expression of mitochondrial FFA oxidative enzymes | Reduced hepatic/muscle FFA oxidation |
Regulation of GLP-2 secretion | Modulation of intestinal L-cell activity | Modulation of intestinal barrier function |
LPS production | LPS-TLR4-mediated induction of hepatic/adipose/macrophagical pro-inflammatory cytokines SOCS-1, SOCS-3, IL-6, TNF-α, MCP-1 | Modulation of systemic/ hepatic/adipose inflammation |
Modulation of gut barrier integrity | Stimulation of L-cell differentiation and GLP-2 secretion | |
Regulation of hepatic/adipose fatty acid composition | Increased linoleic acid conversion to c9, t11 CLA , increased hepatic and adipose contents of DHA and EPA | Modulation of tissue composition of fatty acid |
Animal models suggest obesity is associated with alteration of gut microbiota: germ-free mice have less total body fat than conventionally raised mice. The colonization of germ-free mice with a normal microbiota (composed mainly of Bacteroides and Clostridium genera) results in an increase in total body fat, hepatic triglycerides, fasting plasma glucose, and insulin resistance, despite a reduced food intake. Similarly, conventionalization of germ-free mice with flora from obese donors induces a greater increase in total body fat than colonization with microbiota from lean mice.
Moreover, germ-free mice are protected against the Western diet–induced insulin resistance and gained less body weight and fat mass than conventionalized mice.
Genetically obese leptin-deficient ob/ob mice harbour a significantly higher percentage of Firmicutes and a 50% lower percentage of Bacteroidetes compared with their wild-type littermates fed the same polysaccharide-rich diet. Consistently, in the high-fat/high-sugar Western diet mice, a model of dietary obesity, the development of obesity was associated with enrichment in Firmicutes at the expense of the Bacteriodetes compared with mice receiving a low-fat/high polysaccharide diet. Metagenomic analysis of the obese microbiome showed a depletion of genes involved in motility and an enrichment in genes enabling the capacity of extract energy from the diet, including glycoside hydrolases, phosphotransferases, β-fructosidase and in other transport proteins and fermentation enzymes further processing breakdown products.
Although Bifidobacterium is not a predominating phylum in the gut, it seems to play an important role in host metabolism. In mice, a high-fat diet led to a reduction in Bifidobacterium, associated with increased fat mass, insulin resistance, and inflammatory activity.
Gut microbiota is also connected to metabolic disorders through the modulation of the innate immune system. Mice genetically deficient in Toll-like receptor (TLR) 5, a component of innate immune system in the gut, developed hallmark features of metabolic syndrome, including hyperlipidemia, hypertension, insulin resistance, and increased adiposity, associated with changes in the composition of the gut microbiota. Transplantation of microbiota from TLR5-deficient mice to wild-type germ-free mice conferred many features of metabolic syndrome to the recipients.
Increased Energy Harvest from the Diet
Nutrient absorption and gut motility can be modulated by short chain fatty acids (SCFAs), the major end products of bacterial fermentation. SCFAs (propionate, acetate, and butyrate) represent more than 60% of energy content of carbohydrates from the diet and are ligands for Gpr41 and Gpr43, 2 G protein–coupled receptors that induce intestinal secretion of peptide YY (PYY) and leptin.
Gpr41 functional deletion was related with a reduction in PYY expression, a faster intestinal transit rate, and a reduction of energy uptake from the diet. Consistently, Grp43-deficient mice showed lower total body fat and improved insulin sensitivity; moreover, GPR43 inhibition was associated with higher energy expenditure accompanied by higher core body temperature and increased food intake.
Collectively, these findings disclose the pivotal role for Gpr41 and Gpr43 in mediating microbiota regulation of energy harvest from the diet.
Regulation of Host Energy Storage
In conventionalized mice, microbiota promotes absorption of monosaccharides from the gut lumen. Increased carbohydrate availability promotes de novo lipogenesis in the liver and the adipose tissue by stimulating carbohydrate response element binding protein–mediated and sterol response element binding protein 1–mediated transcription of genes encoding 2 rate-limiting lipogenetic enzymes: acetyl-CoA carboxylase 1 and fatty acid synthase. This mechanism leads to an accumulation of triglycerides in the liver and in adipose tissue.
Fasting-induced adipose factor (Fiaf), also called angiopoietin-like protein 4, is an inhibitor of adipose lipoprotein lipase produced by enterocytes, hepatocytes, skeletal myocytes, and adipocytes in response to fasting, peroxisome proliferator-activated receptor-γ activation, and inflammatory prostaglandins, PGD 2 and PGJ 2 . Fiaf also modulates fatty acid oxidation in skeletal muscle and in adipocytes, increasing the nuclear transcription factor peroxisomal proliferator-activated receptor coactivator 1α, a coactivator of genes encoding key enzymes involved in mitochondrial fatty acid oxidation.
Gut microbiota affect storage of circulating triglycerides into adipocytes by regulating intestinal secretion of Fiaf: conventionalization of germ-free mice suppressed intestinal expression of Fiaf in differentiated villous epithelial cells in the ileum; consistently, germ-free Fiaf-KO mice fed a high-fat/high-carbohydrate diet were not protected against diet-induced obesity. Specific microbiota has different effects on expression of Fiaf: mice fed a high-fat diet supplemented with Lactobacillus paracasei showed increased levels of Fiaf and displayed significantly less body fat and reduced triglyceride levels. In coculture experiments, Lactobacillus also induced Fiaf gene expression. These data suggest that modulation of Fiaf through manipulating gut flora could be an important therapeutic target.
Microbiota may regulate the fatty acid metabolism also by affecting adenosine AMP–AMP (AMPK) activation. AMPK stimulates fatty acid oxidative pathways in the liver and the skeletal muscle through activation of mitochondrial enzymes, such as acetyl-CoA carboxylase and carnitine palmitoyltransferase I, and reduces hepatic glycogen-synthase activity and glycogen stores, improving hepatic and muscle insulin sensitivity.
Gut flora may have an inhibitory effect on AMPK-regulated fatty acid oxidation, because germ-free mice present a persistent activation of hepatic and muscle AMPK, whereas AMPK activity and related metabolic pathways were suppressed in conventionalized mice.
Regulation of Chronic Low-grade Endotoxinemia and Host Inflammatory Response
Chronic activation of the immune system is linked to the development of obesity and T2DM; TLR4-activated inflammatory pathway has been specifically connected with the low-grade chronic inflammation, which characterizes obesity-related disorders.
Gram-negative microbiota may affect host metabolism through lipopolysaccharide (LPS), which binds the complex of CD14 and TLR4 at the surface of innate immune cells, activating inflammatory pathways implicated in the pathogenesis of obesity, insulin-resistance, and T2DM.
Beside LPS, free fatty acid and products from dying cell can bind TLR4 and stimulate inflammatory response in cell expressing TLR4 (gut immune cells, adipocytes, endothelial cells, tissue macrophages, hepatocytes, and hepatic Kupffer and stellate cells). The hepatic Kupffer cells may have an independent role in this contest: in mice, high-fat diet promotes the activation of Kupffer cells, resulting in insulin resistance and glucose intolerance, whereas selective depletion of these cells restores hepatic insulin sensitivity and improves whole-body and hepatic fat accumulation, without affecting adipose tissue macrophages.
Metabolic endotoxiemia is also associated with nonalcoholic steatohepatitis, through hepatic inflammasome activation: a recent study reported, in a mouse model of nonalcoholic steatohepatitis, saturated fatty acids upregulation of the inflammasome that led to sensitization to LPS-induced inflammasome activation. LPS administration modifies the gut microbiota composition (reduction of Bifidobacteria and Eubacteria spp) and determines metabolic effects, such as systemic insulin resistance, increased plasma and hepatic triglyceride content, and reduction of high-density lipoprotein levels ; mice fed a high-fat diet shown the same change in microbiota, associated with a low-grade elevation in circulating LPS levels (metabolic endotoxemia). Consistently, LPS receptor deletion or changes of gut microbiota composition induced by antibiotic administration prevented the metabolic alteration of a high-fat diet.
Modification in gut microbiota composition results in change of metabolic endotoxiemia level: prebiotic fermentable oligofructose (OFS) administration increased the intestinal proportion of Lactobacilli and Bifidobacteria in ob/ob mice, restored normal intestinal permeability through stimulation of epithelial tight-junction proteins, and reduced systemic endotoxiemia, in association with enhanced intestinal glucagon-like peptide (GLP)-2 levels.
Gut microbiota modulates the gut-derived peptide secretion, promoting L-cell differentiation in the proximal colon of rats and increasing GLP-1 secretion in response to a meal in healthy humans ; deletion of GLP-1 abolished the beneficial effects of prebiotics on weight gain, glucose metabolism, and inflammatory pathway activation. Furthermore, gut microbiota may modulate gut barrier integrity and endotoxinemia through GLP-2, a 33-amino acid peptide with known intestinotrophic properties, which is cosecreted with GLP-1 by enteroendocrine L cells.
Ob/ob mice treated with prebiotic plus carbohydrates diet presented an increased circulating GLP-1 and GLP-2, which were associated with an altered gut flora composition (increased proportion of Lactobacilli and Bifidobacteria ), restored tight junction integrity and intestinal barrier function, and lowered endotoxinemia. Administration of a GLP-2 antagonist prevented these effects, which were mimicked by the administration of a GLP-2 agonist, suggesting that GLP-2 could mediate the effects of prebiotics.
Microbiota, such as Bifidobacterium and Lactobacillus , may exert an anti-inflammatory effect through the synthesis of bioactive isomers of conjugated linoleic acid, which shows antidiabetic, antiatherosclerotic, hypocholesterolemic, hypotriglyceridemic, and immunomodulatory activity.
In different mammalian models, dietary supplementation of linoleic acid plus Bifidobacterium breve altered the profile of polyunsaturated fatty acid composition, resulting in higher intestinal, hepatic, and adipose tissue content of c9,t11 conjugated linoleic acid; the animals also present a higher adipose tissue concentrations of eicosapentaenoic acid and docosahexaenoic acid, 2 omega-3 polynsatured fatty acids with anti-inflammatory and lipid-lowering properties. These changes were associated with a reduced expression of proinflammatory cytokines, such as tumor necrosis factor α, interleukin-6, interleukin-1β, and interleukin-8, accompanied with a higher anti-inflammatory interleukin-10 secretion.
Finally, SCFAs elevation also could result in a reduction of the inflammation and an improvement of insulin sensitivity. Butyrate shows anti-inflammatory properties that could improve epithelial permeability. Acetate raised plasma PYY and GLP-1 and suppressed proinflammatory cytokines.
Collectively, these data suggest that endotoxinemia is involved in the pathogenesis of obesity-related diseases, is affected by dietary nutrient composition, and may be modulated by manipulation of gut microbiota composition.
The Role of Vitamin D
Vitamin D deficiency has been associated with allergic diseases development and increased body mass index.
Vitamin D plays a role in immunomodulation and a decreased vitamin D uptake has been correlated with a change in fecal microbiota composition in one study, although this association needs to be confirmed in larger cohorts.
Mice lacking the vitamin D receptor present chronic, low-grade inflammation in the gastrointestinal tract and the absence of the vitamin D receptor results in enhanced inflammation in response to normally nonpathogenic bacterial flora. Moreover, intestinal vitamin D receptor has also been shown to negatively regulate bacterial-induced intestinal nuclear factor κB activation and to attenuate response to infection, suggesting that the vitamin D may affect the impact of intestinal flora on inflammatory disorders.
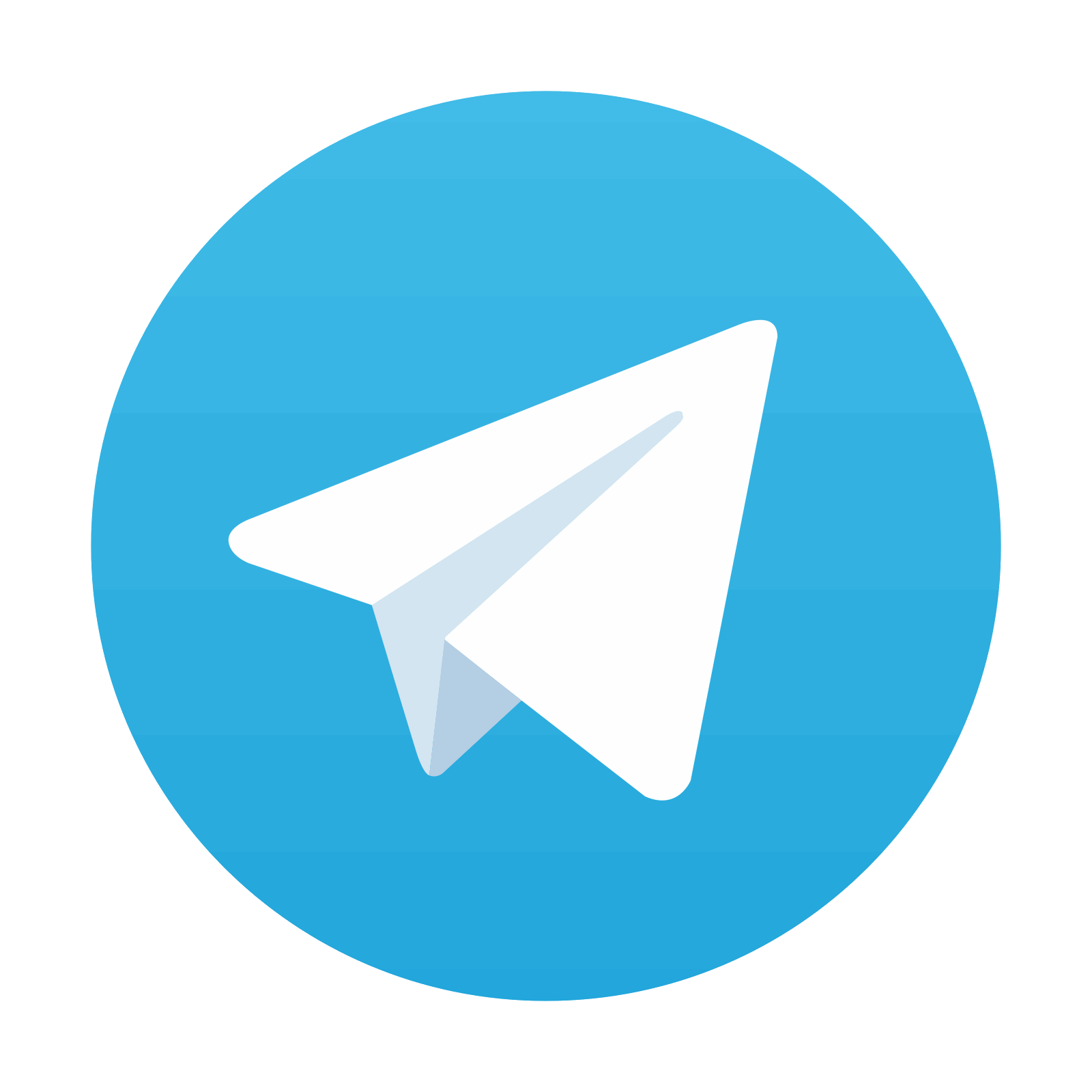
Stay updated, free articles. Join our Telegram channel

Full access? Get Clinical Tree
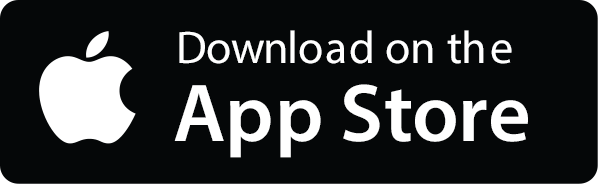
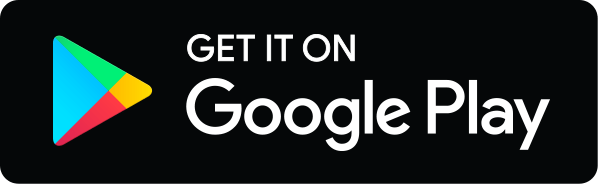