Gut microbiota composition can discriminate between allergic and healthy children, and the distinction may precede clinical manifestations of disease. The mother provides the first inoculum of bacteria, which influences the risk of becoming allergic later in life. Bifidobacterium species are major determinants of disease risk. Specific probiotics may modulate early microbial colonization, which represents the first intervention target in allergic disease, together with their ability to reverse the increased intestinal permeability characteristic of children with atopic eczema and food allergy. Probiotics also enhance gut-specific IgA responses, which are frequently defective in children with food allergy. In addition, probiotics have the potential to alleviate allergic inflammation locally and systemically.
Key points
- •
Allergic diseases constitute the most common chronic diseases of childhood.
- •
Early nutrition exerts short- and long-term effects on health of the host by programming its immunologic, metabolic, and microbiologic development.
- •
Recent scientific advances demonstrate that deviations in gut microbiota composition precede development of the allergic phenotype, inviting preventive and therapeutic applications.
- •
To reverse the increasing burden of allergic diseases, the joint action of specific nutrients and probiotics on the intestinal milieu can be exploited.
- •
The rationale of probiotic intervention comprises restoration of the properties of the unbalanced indigenous microbiota, control of the inflammatory response, and tolerance induction.
- •
The clinical benefit depends on host characteristics and properties of probiotic strains, strain combinations, and their interactions with nutritional components in food.
The hygiene hypothesis and gut microbiota: the starting point
The epidemiologic evidence indicating that early exposure to microbes is associated with a reduced risk of developing atopic sensitization and atopic disease is abundant, and the development of allergic diseases has been attributed to reduced microbial contact. People living in affluent hygienic environments lack the pressure of microbial stimulation, in particular the diversity of microbial contact that has evolved between the host and the gut microbiota, the total genetic pool of the microbiome. The same progression is seen in human nutrition. A shift in food preservation from drying and natural fermentation to industrial pasteurization and sterilization has reduced the microbial exposure associated with food intake. Moreover, a switch from a low-fat, plant-polysaccharide–rich diet to a high-fat, high-sugar diet, collectively described as the Western diet, immediately alters the microbiota composition in the gastrointestinal tract.
The shift of emphasis in the hygiene hypothesis has thus evolved from acute infection protecting from atopic disease to a balanced gut microbiota, the earliest and most massive source of microbial exposure, protecting from the chronic inflammatory conditions, collectively characterized as Western lifestyle diseases. Indeed, environmental changes in the industrialized world reducing microbial contact at an early age have occurred concomitant with the growing epidemic of atopic eczema, allergic rhinoconjunctivitis, and asthma, but also with diseases phenotypically as different as inflammatory bowel disease and type I diabetes, and obesity with its comorbidities. All in all, the development of these conditions points to a failure to generate and maintain a balanced host-microbe interaction, resulting in a tolerogenic milieu in the mucosae, the interface between the internal and external environments of the host. Our conception of the microbes has thus changed; traditional thinking links microbes directly to pathogens and so consequently to acute disease, whereas the current view brings to the fore the microbiome as an important organ of host defense operating at the intersection between host genotype and diet to modulate the host’s adaptation to the modern environment.
The rationale of probiotic intervention here is to provide safe, timely, and sufficient microbial contact to avert the establishment of a deviant gut microbiota and immune responsiveness, and thereby reduce the risk of immunoinflammatory disease. An aberrant gut microbiota composition may result in delayed maturation of gut barrier function, or the perturbation may follow on environmental triggering (ie, gut microbiota changes may precede the development of chronic disease or be merely a consequence of a local inflammatory response). There are data on record to support both conceptions.
It seems that the compositional development of the gut microbiota differs between infants evincing and not evincing atopic manifestations. Healthy infants harbor a natural predominance of bifidobacteria with specific strains, whereas those later developing atopic disease have more Clostridium species and fewer Bifidobacterium species in stools compared with nonatopics. Allergic infants seem to be colonized mainly with Bifidobacterium adolescentis , and healthy infants mainly with Bifidobacterium bifidum and Bifidobacterium longum . The intestinal Bifidobacterium species from allergic infants have been shown to induce proinflammatory cytokine production, in contrast to those from healthy infants, suggesting a need for a more precise characterization at species and strain levels of the healthy gut microbiota. Sequence-based information has recently accumulated, but most of the current methods fail in species or strain-level identification of microbiota components. The precise identification process needs to be completed before the microbial communities can successfully be modulated toward health.
The hygiene hypothesis and gut microbiota: the starting point
The epidemiologic evidence indicating that early exposure to microbes is associated with a reduced risk of developing atopic sensitization and atopic disease is abundant, and the development of allergic diseases has been attributed to reduced microbial contact. People living in affluent hygienic environments lack the pressure of microbial stimulation, in particular the diversity of microbial contact that has evolved between the host and the gut microbiota, the total genetic pool of the microbiome. The same progression is seen in human nutrition. A shift in food preservation from drying and natural fermentation to industrial pasteurization and sterilization has reduced the microbial exposure associated with food intake. Moreover, a switch from a low-fat, plant-polysaccharide–rich diet to a high-fat, high-sugar diet, collectively described as the Western diet, immediately alters the microbiota composition in the gastrointestinal tract.
The shift of emphasis in the hygiene hypothesis has thus evolved from acute infection protecting from atopic disease to a balanced gut microbiota, the earliest and most massive source of microbial exposure, protecting from the chronic inflammatory conditions, collectively characterized as Western lifestyle diseases. Indeed, environmental changes in the industrialized world reducing microbial contact at an early age have occurred concomitant with the growing epidemic of atopic eczema, allergic rhinoconjunctivitis, and asthma, but also with diseases phenotypically as different as inflammatory bowel disease and type I diabetes, and obesity with its comorbidities. All in all, the development of these conditions points to a failure to generate and maintain a balanced host-microbe interaction, resulting in a tolerogenic milieu in the mucosae, the interface between the internal and external environments of the host. Our conception of the microbes has thus changed; traditional thinking links microbes directly to pathogens and so consequently to acute disease, whereas the current view brings to the fore the microbiome as an important organ of host defense operating at the intersection between host genotype and diet to modulate the host’s adaptation to the modern environment.
The rationale of probiotic intervention here is to provide safe, timely, and sufficient microbial contact to avert the establishment of a deviant gut microbiota and immune responsiveness, and thereby reduce the risk of immunoinflammatory disease. An aberrant gut microbiota composition may result in delayed maturation of gut barrier function, or the perturbation may follow on environmental triggering (ie, gut microbiota changes may precede the development of chronic disease or be merely a consequence of a local inflammatory response). There are data on record to support both conceptions.
It seems that the compositional development of the gut microbiota differs between infants evincing and not evincing atopic manifestations. Healthy infants harbor a natural predominance of bifidobacteria with specific strains, whereas those later developing atopic disease have more Clostridium species and fewer Bifidobacterium species in stools compared with nonatopics. Allergic infants seem to be colonized mainly with Bifidobacterium adolescentis , and healthy infants mainly with Bifidobacterium bifidum and Bifidobacterium longum . The intestinal Bifidobacterium species from allergic infants have been shown to induce proinflammatory cytokine production, in contrast to those from healthy infants, suggesting a need for a more precise characterization at species and strain levels of the healthy gut microbiota. Sequence-based information has recently accumulated, but most of the current methods fail in species or strain-level identification of microbiota components. The precise identification process needs to be completed before the microbial communities can successfully be modulated toward health.
Allergic disorders: a moving target for therapeutic and preventive measures?
Allergic disorders represent a profound pediatric health problem, the most common chronic disease state among children. Notwithstanding the extensive scientific interest centered on this problem, research so far has been unable to conclusively ascertain the determinants underlying the epidemic of allergy. However, the most recent advances in research into chronic inflammatory disease have uncovered similarities in the mechanisms of diseases of the T helper (Th) 1-type, such as inflammatory bowel disease, and atopic disease of the Th2-type, and obesity, and thereby opened up new angles on the role of environmental exposures in the development and perpetuation of allergic disease. Specifically, studies on microbial contact early in life, including probiotic research, have linked aberrant gut microbiota and inflammatory responses during critical periods of maturation to perturbed immunologic homeostasis.
Immune priming by microbial exposure may be essential particularly for the gut-associated immune system to fulfill its two opposing functions: mounting a brisk response to pathogens and maintaining hyporesponsiveness to innocuous antigens. The healthy gut microbiota shapes the host responder type and its resilience to environmental offense (reviewed in ). Recent experimental and clinical reports also highlight the way the immune regulation exerted by the gut microbiota extends to systemic immunity and to peripheral tissues, such as the lung. Current research interest is focused on these active immunologic processes promoting tolerance and their potential for exploitation in clinical intervention studies. However, deeper insight into the multiple mechanisms of allergic disease is still needed for the validation of specific strains carrying antiallergic potential.
Epigenetic variables tie the hereditary susceptibility to the allergic phenotype in general, but not to definite allergic diseases. The heterogeneous manifestations of what are called “allergic disorders” (ie, the diverse phenotypes) remain clinically and immunologically poorly defined, and consequently no universal remedy can be expected. Hence, evaluation of the probiotic potential as an alternative to attain prophylactic or therapeutic effect in “allergic disorders” calls for an accurate characterization of the target: definition of the study population, in terms of age, immune responder type, diet, including the presence or absence of food allergies, and the composition and activity of the existing gut microbiota, exemplifying common determinants interacting with probiotics.
Because of confusion in the definition of allergic disorders, alternative approaches have been endorsed. These include scientific validation of and criteria for markers to support health claims for foods, including probiotics, the current ILSI Europe working group on “Monitoring immune modulation by nutrition in the general population,” which focuses on adapting methodology to assess risk reduction in the healthy European consumers. In a similar manner, the European Science Foundation has gathered a forward look assessment to cover future research targets in this area.
To define the strategic direction for clinical studies, one hypothesis has been pinpointed as a working target for probiotics in the development and treatment of allergic conditions : impaired barrier functions of the skin epithelium and gut mucosa, including the local microbiota in these functions ( Fig. 1 ). Specific probiotics counteract the hypersensitivity process by modifying the immunomodulatory activity of dietary antigens, and potentiate the host’s endogenous defense mechanisms by stabilizing the gut mucosal barrier function.
It seems justified to expect that clinical research focusing on the critical window and the mechanisms involved, together with the environmental elements that interact with the most effective probiotic strains, will devise novel preventive therapies.
Establishment of the gut microbiota: new insight into probiotic action
Environmental exposures during the critical stage of maturation may directly shape the risk of disease. Certain sources of exposure go beyond all others: the establishment of the gut microbiota, the mode of delivery, and breastfeeding. Recent experimental and clinical studies have demonstrated that the mother’s nutritional status and immune responses during pregnancy, and the mode of delivery and breastfeeding/breast milk composition, all exert an important impact, the gut microbiota interacting with all other elements in the nutritional, immunologic, and microbiologic programming of child health ( Fig. 2 ).
Compositional Development of the Gut Microbiota
Although colonization process in the infant gut has been intensively studied, the early events guiding microbiome development remain unresolved. Establishment of the gut microbiota can be characterized by three steps. The first step of colonization at and after birth involves mainly facultative anaerobic bacteria. Among the colonizers are bacteria derived from the mother’s gut and skin, including Escherichia coli , clostridia, staphylococci, and some Bacteroides species.
The second step comprises a rapid succession by anaerobic genera, such as Bifidobacterium , Eubacterium , Clostridium , and increases in Bacteroides species, especially in breastfed infants. Different species of bifidobacteria can range from 60% up to 90% of the total fecal microbiota in breastfed infants and frequently the composition comprises Bifidobacterium breve , B infantis , and B longum species, whereas the most common lactobacilli in breastfed and formula-fed infant feces are usually related to the Lactobacillus acidophilus group.
The third major step coincides with weaning, which heralds a major shift leading to a microbiome consisting of microbial consortia characteristic of the adult microbiota. During this step, rapid changes also take place in energy-harvesting Bacteroides species, these increasing a thousandfold. The adult type of microbiota has been estimated to be achieved by 2 to 3 years of age. Shifts in abundance in major taxonomic groups occur during this time period, this presumably reflecting the diet or health status of the host.
Microbial Contact in Prenatal, Perinatal, and Postnatal Periods
Current dogma defines fetal life as microbiologically sterile except in cases of infection. Investigations on microbial growth during the fetal period make no reference to the normal microbiota before delivery and the newborn gut has also been described as sterile. The intrauterine environment is likely to be protected from and protective against pathogenics, but it is coming to be accepted that a very selective microbial exposure ensues already before birth, because placenta and amniotic fluid contain specific bacteria. Particularly, placenta samples after extremely premature deliveries have been documented to contain diverse bacteria. Conversely, pathogenic bacteria in the placenta and amniotic membranes may lead to premature birth, or to neonatal and maternal infection. However, there are data suggesting that small quantities of microbes are also present in the fetoplacental area in term pregnancies, but their sources and roles remain undefined. One mechanism and route for the exposures currently being investigated is through the maternal mucosal system. The recently reported presence of bacterial DNA in the neonate meconium likewise tends to corroborate the prenatal origin of microbes.
Taken together, one could consider the microbiota transfer from the mother to the newborn during the perinatal period as a continuum and as reflecting the close environment. This inoculum may induce adaptation to the anticipated postnatal environment, concomitantly impacting on later disease susceptibility.
Breastfeeding
After birth, microbial contact continues through breastfeeding. Human milk is generally considered the optimal nutrition for neonates because it contains the required nutrients and protective compounds, including specific oligosaccharides and fatty acids influencing early microbial colonization, but also specific microbiota and molecules operating in host-microbe interaction. Indeed, there is abundant evidence that breast milk also transmits bacteria to the infant gut : the mother provides the infant with bifidobacteria, lactic acid bacteria, and other microbiota components in significant quantities during breastfeeding. Breastfeeding also exposes the infant to the mother’s skin bacteria. Perez and colleagues have shown that some bacterial signatures in breast milk are common to the infant’s feces and mother’s blood samples, which implies a link. Hence, the milk microbiota contains a distinct bacterial community from skin, gut, vagina, or mouth.
The microbiota transfer can constitute a protective microbiota or mediate the mother’s aberrant microbiota composition to the infant, depending on maternal health and nutritional state. Collado and coworkers have shown that the composition and development of the infant gut microbiota are influenced by the body mass index (BMI), weight, and weight gain of the mother during pregnancy. In a similar manner, mothers with allergic disease have deranged counts of bifidobacteria in breast milk and the maternal fecal and breast-milk bifidobacterial counts guide the compositional development of the Bifidobacterium microbiota in the infant.
Mode of Delivery
The mode of delivery has been reported to impact on microbiota transfer from the mother to the infant and the effects are present immediately after birth, but also beyond infancy. The mode of delivery has been demonstrated to influence the acquisition and structure of the initial microbiota in multiple body habitats in newborns, especially in respect of the levels of Clostridium difficile , which may subsequently influence the risk of atopic manifestations. Moreover, it has recently been demonstrated that the mode of delivery has an impact on breast milk microbial composition, which differs strikingly between mothers delivering vaginally and those undergoing caesarean section, but interestingly also between the type of caesarean delivery (elective vs nonelective caesarean sections). These observations suggest that the lack of physiologic stress or hormonal signals during labor may influence the process of microbial transmission to the mother’s milk.
Probiotics: definitions and specifications
According to the World Health Organization definition probiotics are “Live microorganisms which when administered in adequate amounts confer a health benefit on the host.” The definition also requires the use of a nomenclature of bacteria, which conforms to the current, scientifically recognized terminology. Bacteria should be characterized and deposited in international culture collections with deposit numbers to ensure consistency in research. These are the requirements most scientific journals also set forth to facilitate the understanding and interpretation of results concerning specific probiotics. However, other factors hitherto overlooked impacting on probiotic properties include the manufacturing process and the food matrix used. Both have been reported to modify probiotic properties significantly. Such interaction should also be assessed before human intervention studies.
Strict adherence to the code of strain specifications and identification of manufacturer and probiotic quality and viability measures would also aid in strain-specific meta-analysis and comprehensive reviews on probiotic efficacy. For instance, most nutrition journals require authors to deposit the microbial strains they use in any study submitted in publicly accessible culture collections (eg, the American Type Culture Collection) and to refer to the collection and strain numbers in the text. When the authenticity of subcultures of culture collection specimens that are distributed by individuals cannot be ensured, authors should indicate the relevant laboratory strain designations and donor sources, and the original culture collection identification numbers.
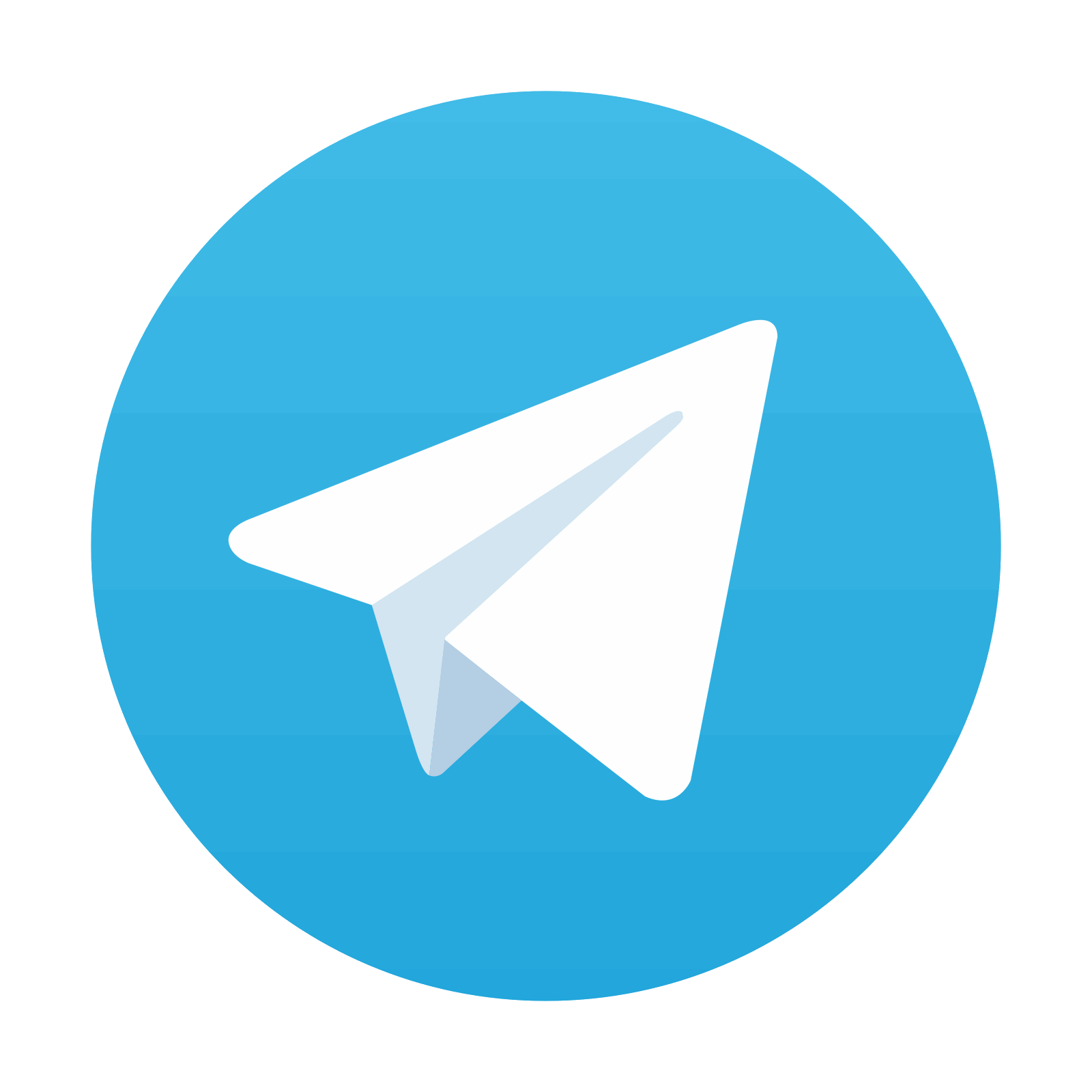
Stay updated, free articles. Join our Telegram channel

Full access? Get Clinical Tree
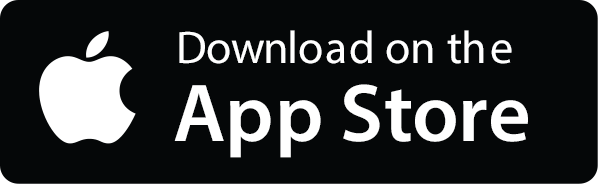
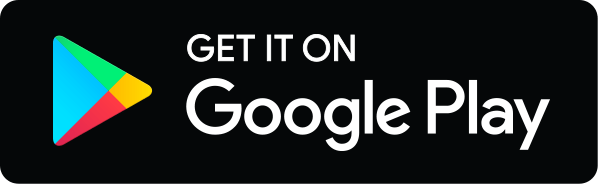