Epidemiology
Affecting more than 1.5 million Americans annually, coronary artery disease is the most common form of heart disease, and its complications are the leading cause of death in both men and women.
Pathophysiology
Coronary artery disease is generally caused by atherosclerotic luminal narrowing, resulting in insufficient coronary blood flow to the myocardium. The process consists of subintimal atheroma deposition, leading to arterial luminal stenosis or occlusion and wall thickening.
Clinical features
Typically, significant coronary atherosclerosis becomes manifest as angina, but it can also present with anginal-equivalent symptoms, including dyspnea, dizziness, syncope, and pulmonary edema. Malignant coronary disease leading to ischemic cardiomyopathy is associated with congestive symptoms. Identified factors that contribute to severe atherosclerotic coronary artery disease include obesity, hypercholesterolemia, obesity, diabetes, tobacco use, and sedentary lifestyle.
Diagnostics
Twelve-lead electrocardiography is initially used to diagnose myocardial ischemia. Further investigation consists of exercise stress testing, evaluation of myocardial enzymes (i.e., CPK-MB, troponin), echocardiography, and coronary angiography.
Treatment
Initial medical management of acute myocardial ischemia consists of beta blockade, nitrates, anti-platelet therapy, and supplemental oxygen. After precise delineation of coronary arteriopathy, definitive treatment consists of coronary artery bypass grafting (CABG) or percutaneous angioplasty and stenting.
Outcomes/prognosis
CABG carries an overall mortality of about 3 percent; elective primary coronary bypass carries a mortality rate of approximately 1.7 percent. Complications associated with CABG include renal failure, neurologic injury, heart failure, hemorrhage, respiratory failure, and renal dysfunction. Overall, CABG achieves excellent outcomes with respect to anginal relief and resumption of normal activities. In general, completeness and durability of revascularization is superior with surgical revascularization versus percutaneous interventions.
Coronary artery disease represents the most common cause of insufficient oxygen delivery to the myocardium, referred to as myocardial ischemia. Understanding the pathophysiology of arterial disease with subintimal atheroma and plaque formation has been a breakthrough of modern medicine. Even with our advanced understanding of heart disease, it remains the most common cause of death for Americans. Cardiovascular disease claims more lives (men and women) than cancer, trauma, and pulmonary disease combined.1
The first coronary artery bypass grafting (CABG) operation, using saphenous vein conduit, was performed by Dr. David Sabiston at Johns Hopkins Hospital in 1962 and has evolved into the “gold standard” therapy for patients with multivessel coronary artery disease. Despite advances in percutaneous interventional techniques, CABG remains among the most frequently performed operations in the United States, associated with approximately US$50 billion in annual health care expenditures.
The right and left coronary arteries originate from the ascending aorta behind their respective aortic valve leaflets, usually in the upper third of the sinuses of Valsalva.2 By convention, the artery that supplies the posterior descending artery (PDA) determines the coronary dominance. The coronary circulation is classified as “right dominant” if the PDA is supplied by the right coronary artery and as “left dominant,” when supplied by the left circumflex artery. Occasionally, the PDA is supplied by both the left and the right coronary circulation and is therefore classified as “co-dominant.” The coronary circulation is right dominant in 85 to 90 percent of normal individuals. This delineation is important when evaluating patients for CABG.
The right coronary artery is usually a singular vessel coursing down the right atrioventricular groove, supplying the anterior right atrial and sinoatrial nodal arteries. It continues over the acute margin of the heart, dividing into the right posterior descending and right posterolateral segmental arteries, perfusing the atrioventricular node and posterior wall of the left ventricle.
The left main coronary artery courses for a distance of 10 to 20 mm from the left sinus of Valsalva between the pulmonary trunk and left atrial appendage. It bifurcates into the left anterior descending (LAD) and circumflex coronary arteries near the left atrioventricular groove. The LAD traverses the anterior interventricular groove toward the apex of the heart, giving off diagonal, septal perforating, and right ventricular branches before terminating at the apex. Occasionally, in 10 to 15 percent of normal individuals, the LAD may collateralize with the PDA or replace it to supply the posterior interventricular groove. The diagonal branches supply the anterolateral wall of the left ventricle. The first diagonal branch may occasionally originate directly from the left main coronary artery and is then referred to as the ramus intermedius coronary artery. The septal perforators supply the anterior two-thirds of the ventricular septum, extending perpendicularly from the LAD. The right ventricular branches supply the anterior surface of the right ventricle.
The circumflex coronary artery courses along the left atrioventricular groove, giving off obtuse marginal branches to supply the lateral wall of the left ventricle, the left atrium, and the posteromedial papillary muscle. Occasionally, the sinus node artery is supplied from the proximal circumflex artery. In most patients, the circumflex coronary artery terminates near the obtuse margin of the left ventricle; in the remaining patients, (i.e., left dominance), it may continue and give rise to the posterior descending coronary artery.
Coronary blood flow is regulated by the oxygen demands of the heart.3 Oxygen stores in the heart are minimal with extraction from the coronary blood flow at near maximal levels (70 percent) under a resting physiologic state. Due to this maximal oxygen extraction, when oxygen demands increase, as in exercise, a majority of oxygen delivery must come from increasing blood flow. Changes in myocardial oxygen requirements bring about a multitude of mechanisms to increase oxygen delivery. The major resistance vessels where these mechanisms are located constitute the arterioles and rich network of capillaries that form interregional collaterals. Myocardial blood flow is under complex regulation by a compilation of metabolic, neurogenic, and hormonal mechanisms.
Adenosine is the primary metabolite of myocyte activity regulating coronary blood flow via A2 receptor-induced smooth muscle relaxation. ATP sensitive K+ channels, hypoxia, and acidosis are other potent metabolic regulators of coronary blood flow. Neurogenic regulation occurs directly and indirectly via sympathetic and vagal innervations. Direct innervations of larger conductance vessels and indirect innervations of smaller resistance arterioles with release of norepinephrine cause vasoconstriction (via α1 receptors) and vasodilatation (via β2 receptors). Vagal release of acetylcholine primarily causes vasodilatation of conductance vessels. Hormonal control of coronary blood flow occurs mainly by local endothelial derived substances such as nitric oxide (vasodilatation) and endothelin-1 (vasoconstriction). However, nonlocal paracrine control of coronary blood flow can be influenced by platelet derived mediators such as thromboxane A2 (vasoconstriction), adenosine diphosphate (vasodilatation) and serotonin (both).
Given the near obligatory aerobic physiology of the heart, metabolic, neurologic, and hormonal mechanisms quickly react to increase coronary blood supply and adequate oxygen delivery. With this autoregulatory system, the heart is particularly vulnerable to occlusive arterial disease.
Coronary atherosclerosis is a slow, complex disease process that typically starts in childhood and often progresses with age. Its precise cause still remains incompletely understood. The “Response to Injury” hypothesis was put forth by Ross and Glomset who combined proposals made initially by Virchow and Rokitansky with more modern knowledge of molecular and cellular biology.4 Infiltration of small lipoprotein particles accumulate in the vessel intima, starting in childhood. Binding of these lipoproteins to proteogly-can make these areas more prone to endothelial injury.5 Mechanisms of endothelial injury are thought to include: turbulent flow, infectious and immunologic factors, and noxious chemicals. Once leukocytes extravasate into the intima, they become lipid-laden macrophages and multiply. With the release of various chemotactic growth factors and vasoconstrictors such as thromboxane A2, migration and proliferation of smooth muscle cells results in the accumulation of collagen, elastic fibers, proteoglycan, calcium, complex carbohydrates, and other connective tissues in the intimal and subintimal areas.6 This deposition progresses to become an atherosclerotic plaque. Within an atherosclerotic plaque, angiogenesis of newly formed small vessels can suddenly bleed, causing plaque hemorrhage and rupture, which can worsen the degree of stenosis and precipitate a myocardial infarction.7 It is believed that most acute myocardial infarctions result from acute thrombotic occlusion.
Coronary atherosclerosis usually involves the proximal portions of larger epicardial coronary arteries, especially at or just beyond branching sites. Therefore, stenoses of the LAD, circumflex, and right coronary arteries often involve the first of the secondary branchings (i.e., first diagonal, obtuse marginal, and posterior descending branches). When the disease is more severe, origins and main trunks may be involved. The left main coronary artery is significantly diseased in 10 to 20 percent of patients. Diffuse distal disease severe enough to render a coronary artery unsuitable for bypass grafting is relatively uncommon.
With coronary artery disease, myocardial ischemia and necrosis occur when coronary blood flow, impaired by atherosclerotic stenosis, is insufficient to meet oxygen demand. Because the heart has virtually no reserve stores of oxygen and relies entirely on aerobic metabolism, its high rate of energy expenditure results in a sudden, striking decline of oxygen tension and left ventricular functional impairment within seconds of coronary occlusion. Coronary occlusion can cause myocardial ischemia in as little as 60s, and depressed function, or myocardial stunning, in less than 20 min.8 The subendocardium is most vulnerable to myocardial ischemia because its collateral blood flow is lowest and oxygen consumption highest.9 Thus, myocardial necrosis progresses toward the epicardium with continued ischemia and is accelerated when there is little collateral flow, marked arterial hypotension (e.g., cardiogenic shock), and elevated oxygen demand caused by inotropic stimulation or tachycardias. Thus blood pressure control and the prevention of tachyarrhythmias are vital during the early postinfarction interval to limit the spread of myocardial damage. The recognition of this time-dependent progression of infarct and the conditions necessary for functional recovery of salvaged myocardium constitute the basis for rapid reperfusion interventions, including thrombolysis and angioplasty. Multiple randomized trials have demonstrated that fibrinolytic therapy within 1 h hour of symptoms remarkably decreases the 30-day mortality of acute myocardial infarction.10
Hypercholesterolemia and other conventional risk factors may precipitate or accelerate the process of coronary atherosclerosis. The identified controllable risk factors are:
Hypercholesterolemia (especially low-density lipoprotein >100 mg/dL)
Smoking or exposure to tobacco smoke
High blood pressure
Diabetes mellitus
Obesity
Physical inactivity
Although global risk assessment models like Framingham are useful, up to 20 percent of patients with coronary artery disease have no conventional risk factors and up to 50 percent only have one risk factor. In light of this, additional risk factors for coronary atherosclerosis are being investigated and tested, including C-reactive protein, lipoprotein A, fibrinogen, and homocysteine.
Angina pectoris is the development of chest discomfort or pain with exertion that ceases within 5 to 15 min of rest, or administration of sublingual nitroglycerin. It is typically described as a substernal heavy, dull, or crushing pain with frequent radiation to the left shoulder, arm, and/or neck. Other sensations are more vague, including pressure, numbness, and burning. It is not an inevitable symptom, nor does its absence indicate freedom from coronary artery disease; however, it does signify the presence of reversible myocardial ischemia without cellular necrosis. Decreased myocardial oxygen supply or increased myocardial oxygen demand leads to symptoms. Since angina results from a reduction in coronary flow reserve, the greater the reduction, the more severe the angina. Several classification systems exist, however the Canadian Cardiovascular Society Functional classification has become the most accepted.11 The severity of angina is categorized into the following four categories:
Class 1: Angina occurring with strenuous activity. There is no limitation to ordinary activity.
Class 2: Angina occurring with fast-paced walking or walking up an incline or stairs. There is only slight limitation to ordinary activity.
Class 3: Angina occurring when walking less than two blocks on level ground or climbing one flight of stairs. There is significant limitation to ordinary activity.
Class 4: Angina occurring with mild activity. May occur at rest but lasts less than 15 min (beyond which the angina is unstable). There is inability to carry out mild activity.
The clinical presentation of patients with angina varies considerably. Part of this is explained by fixed threshold versus variable threshold angina. Fixed threshold angina is due to increased myocardial oxygen demands. The level of exertion to precipitate oxygen debt resulting in angina is relatively “fixed” (two flights of stairs). Variable threshold angina includes dynamic vasoconstriction as an important part of decreased oxygen supply, not just atherosclerotic narrowing of epicardial coronary vessels. These patients are noted to have “good days” and “bad days” as their level of exertion that precipitates angina is varied.
Furthermore, as chest pain in general tends to be nonspecific, the differential diagnosis is broad and includes gastrointestinal reflux, biliary colic, peptic ulcer disease, aortic dissection, esophageal disorders, lower respiratory tract infection, pneumonitis, and a variety of musculoskeletal discomforts. Once other differential diagnoses are ruled out, evaluation with electrocardiography (ECG), exercise or stress testing, and myocardial enzyme levels are necessary to confirm the diagnosis of myocardial ischemia. In general, patients with class 1 and 2 stable angina can be managed medically with coronary angiography as an option following provocative testing for ischemia. Coronary angiography is recommended for patients with class 3 and 4 angina.
Anginal equivalents are described as symptoms of myocardial ischemia other than angina. These symptoms include dyspnea, fatigue, syncope, dizziness, and pulmonary edema. These symptoms are common in the elderly and often due to left ventricular systolic or diastolic dysfunction whenever the myocardium becomes ischemic under stress. Furthermore, a small number of patients exhibit “silent” or asymptomatic ischemia that can be discovered only by continuous ECG monitoring. Nocturnal angina should raise the suspicion of sleep apnea.
Acute coronary syndromes are a spectrum of symptomatic and prognostic worsening of coronary artery disease. Included in this spectrum are unstable angina, non-ST-segment elevation myocardial infarction, and Prinzmetal angina. They are predominantly due to plaque rupture and variable thrombosis/occlusion of the coronary arterial system. Other proposed mechanisms of acute coronary syndrome include coronary spasm as seen in Prinzmetal angina and secondary causes of decreased myocardial oxygen demand such as anemia. This clinical situation remains reversible but tends to recur either as further episodes of unstable angina or a fulminant acute myocardial infarction.
Unstable angina is defined as angina pectoris pain (or equivalent) that includes at least one of the following three characteristics: (1) lasting greater than 20 min if not interrupted by medical intervention, (2) being severe and recent (within 1 month of onset), and (3) presents with crescendo patterns (being more painful, longer duration, or increasing frequency). Furthermore, unstable angina can be categorized according to the clinical presentation of each ischemic event: primary unstable angina, secondary angina (i.e., anemia), and postinfarct angina (less than two weeks after myocardial infarction).
Prinzmetal angina is a variant that causes pain and ST-segment elevation at rest. The proposed mechanism has been increased coronary vasomotor tone or spasm. No precise mechanism has been elucidated, but mutations in genes responsible for the production of nitric oxide and endothelium-derived relaxing factors may play a role.12 The crucial element to diagnose Prinzmetal variant angina is the detection of episodic ST-segment elevation with pain. ST-segment deviations may be present in any leads. Treatment for Prinzmetal variant angina includes smoking cessation, short acting and long acting nitrates, and calcium agonists. Patients with ventricular fibrillation associated with Prinzmetal variant angina may require automatic implantable cardiodefibrillator placement.13
Patients presenting with acute coronary syndrome are evaluated with clinical history, ECG examination, and cardiac biomarker (troponin) level measurement. These patients are treated depending on their risk stratification. Those with low risk are treated with an antithrombotic agent such as aspirin, clopidogrel, or an antithrombin, along with β-blockers and nitrates. Patients with positive troponin levels or ST-segment changes (high risk) are treated with the addition of GP IIb/IIIa inhibition as well as coronary angiography with percutaneous coronary intervention (PCI) or CABG if necessary. A calcium agonist is added for patients with Prinzmetal variant angina. Several trials demonstrate that early intervention in high-risk unstable angina patients results in decreasing mortality, myocardial infarction, and rehospitalization rates.14
Prolonged myocardial ischemia without reperfusion ultimately leads to irreversible cellular necrosis and myocardial infarction. Most acute myocardial infarctions result from subtotal or total coronary artery occlusion by a thrombus associated with acute rupture of an atherosclerotic plaque. Although the acutely occluded coronary artery often was not previously severely stenotic, the mere presence of significant coronary artery disease clearly increases the risk for acute myocardial infarction. For example, patients with severe proximal LAD coronary lesions are particularly prone to suffer acute and often fatal myocardial infarctions. Increased numbers of coronary arteries with stenotic disease also lead to higher probabilities of acute myocardial infarction.
Following an infarction, the location of the coronary arterial occlusion, presence of other diseased vessels, and extent of collateral perfusion will determine the extent of myocardial injury in the early period. Additional detrimental effects of postinfarct arrhythmias, hypotension, left ventricular distention, and increased wall stress can further reduce the viability of borderline regions and increase the ultimate infarct size.
According to the American Heart Association, the updated universal definition of myocardial infarction is15: Detection of rise and/or fall of cardiac biomarkers (preferably troponin) with at least one value above the 99th percentile of the upper reference limit (URL) together with evidence of myocardial ischemia with at least one of the following:
Symptoms of ischemia;
ECG changes indicative of new ischemia [new ST–T changes or new left bundle branch block (LBBB)];
Development of pathologic Q waves in the ECG;
Imaging evidence of new loss of viable myocardium or new regional wall motion abnormality.
Typically, patients present with a sudden onset of severe chest pain or their anginal equivalent that is not relieved by rest or sublingual nitroglycerin. Often, there is associated shortness of breath, nausea, diaphoresis, and general malaise. Subendocardial or nontransmural infarctions usually demonstrate new ST-segmental depressions and T-wave inversions on the 12-lead ECG. As the infarction progresses, ST segments may become elevated and later evolve into Q waves that indicate a transmural infarct.
Since less than 25 percent of patients admitted to the hospital with ischemic-type chest discomfort are subsequently diagnosed as having had an acute myocardial infarction and about half of all patients with acute myocardial infarction do not exhibit diagnostic ST-segment changes, myocardial enzyme markers play an essential role in establishing the diagnosis. The classic myocardial creatine kinase isoenzyme CK-MB, which appears within hours after injury and peaks at 8 to 24 h after infarction, lacks sufficient sensitivity and specificity but remains an efficient means of early detection. Levels of troponin, with its cardiac specific subunits I and C, are more sensitive and specific for myocardial infarction. Furthermore, because troponin release is stoichiometrically correlated with the amount of myocardial necrosis, these enzymes can also be used to estimate infarct size and prognosis. Since serum troponin levels may present up to 14 days after infarction, detection of recurrent acute infarction requires concomitant CK-MB measurements.
Some patients with progressive coronary artery disease suffer from chronic, recurrent myocardial ischemic episodes rather than a single devastating infarction. Consequently, these patients develop symptoms of chronic ischemic cardiomyopathy and congestive heart failure (shortness of breath and peripheral edema). Myocardial ischemia impairs left ventricular function and increases the workload on normal myocardium. Increasing areas of dyskinesis or akinesis from repeated insults results in greater reduction of both systolic and diastolic function. Often, these patients exhibit symptoms of heart failure associated with recurrent myocardial ischemia. Furthermore, right ventricular function can also be expected to be impaired in patients with chronic ischemic heart disease. This can be due to occlusive disease of the right coronary artery, pulmonary arterial hypertension, dyskinesis of the ventricular septum, and left ventricular dilatation.
There is a great deal of physiologic and functional variability among patients with depressed ventricular function from chronic ischemic heart disease. Therefore preoperative prediction of the efficacy of surgical intervention can be difficult. Some patients have moderately increased left ventricular end-diastolic pressures and reduced exercise capacity but minimal cardiomegaly. Although these patients often have marked ischemic dysfunction, revascularization is indicated to rescue or protect viable myocardium and improve functional capacities. In some cases, poor left ventricular function is due to myocardial “hibernation” or “stunning” and therefore may benefit from revascularization. In general, patients with recurrent heart failure and reversible ischemic episodes are likely to improve after CABG.
In some patients, chronic myocardial ischemia leads to ischemic cardiomyopathy. This is signified clinically by moderate-to-severe cardiomegaly, reduced cardiac output, significantly elevated right-sided venous pressures, hepatomegaly, ascites, and peripheral edema. The advanced left ventricular dysfunction is the result of extensive myocardial scarring due to a sustained reduction in coronary perfusion. These patients tend to have diffuse small vessel coronary disease and are therefore not expected to benefit from revascularization.
With the advent of coronary stent technology, indications for coronary artery bypass surgery are evolving. Indications for coronary artery bypass surgery are predicated on improving the patient’s quality of life (i.e., relief of symptoms, increased exercise tolerance, prolonged survival). Based on an extensive review of the literature, a joint task force from the American College of Cardiology and American Heart Association revised the original 1991 guidelines for coronary artery bypass surgery in 2004 (Table 27-1).16 Nominally, based on several studies showing improved myocardial function following operative reperfusion, emergency surgical revascularization is generally preferred over medical/percutaneous intervention after acute myocardial infarction. There is general agreement that CABG provides a survival advantage as compared with medical therapy in patients with (1) left main stenosis, (2) three-vessel disease, (3) two-vessel disease with proximal LAD stenosis, (4) impaired left ventricular function, and (5) severe ischemia and multivessel disease. Percutaneous interventions are now available, which provide improved symptoms over medical therapy alone; however, survival advantages have not been seen.17,18
|
|
|
|
|
|
|
|
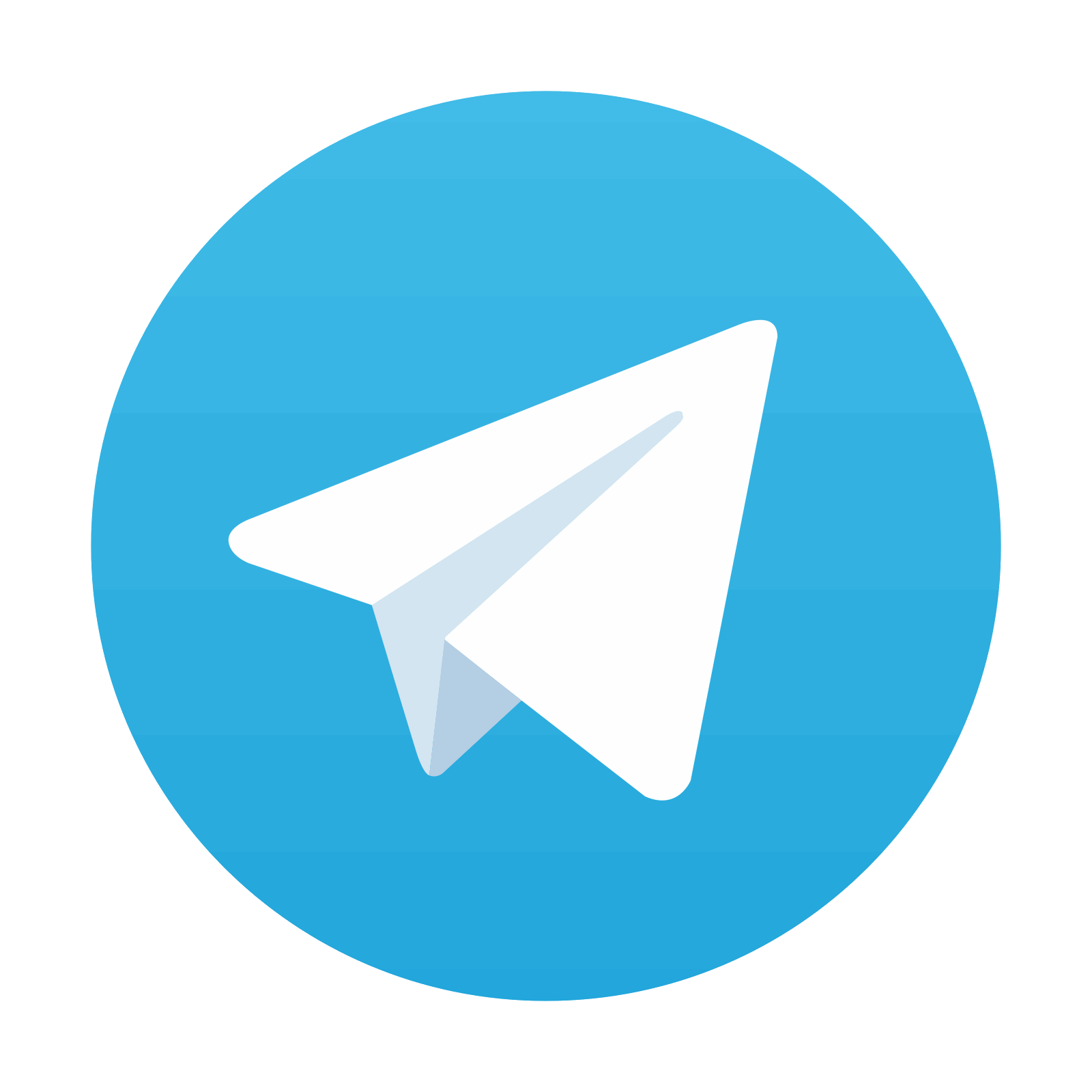
Stay updated, free articles. Join our Telegram channel

Full access? Get Clinical Tree
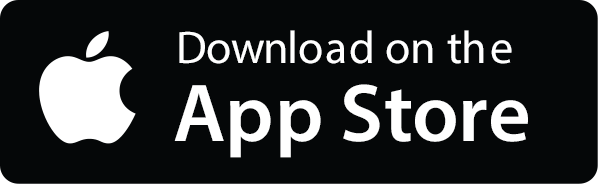
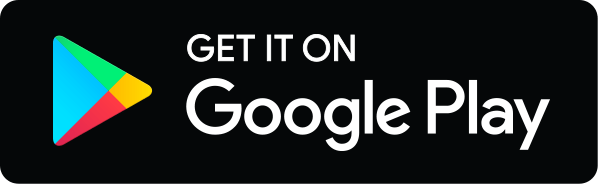
