Fig. 3.1
Preoperative hepatobiliary scintigraphy in a 56-year-old male patient with a large resectable HCC in the right liver segments. Summed dynamic scintigraphy (a) showing hypertrophy and function of the left liver segments. The future remnant liver function was determined at 3.07%/min/m2. Transverse (b), coronal (c) and sagittal (d) SPECT-lowdoseCT planes of the liver showing a large non-functional mass in the right liver and hypertrophy of left liver segments with sufficient function for safe right hemihepatectomy
99mTc-mebrofenin HBS with SPECT-CT is gaining applicability in patients undergoing PVE. Recent reports have indicated that the increase in the FLR function is more pronounced than the increase in the FLR volume [125]. This finding suggests that the time interval between PVE and liver resection should not be determined by volumetric parameters alone. Another possible application of HBS in this group of patients is the selection of candidates for PVE, as prediction of liver failure on the basis of function of the FLR can be done more accurate by HBS.
Monitoring of regeneration of liver function after resection is another potential application of HBS. As Bennink et al. already described, volumetric regeneration after partial liver resection does not correlate with functional regeneration measured with HBS, while the latter has been shown to correlate with ICG clearance [120].
Other Modalities for Assessment of Liver Function
Bioenergetic Tests
A key determinant of liver functional status and reserve is the energy state of the organ. The availability of adenosine triphosphate (ATP) is therefore critical for the maintenance of integrity and function of liver cells, particularly since the liver is the most metabolically active organ. When the ATP-generating ability of liver cells is compromised, as is the case in chronic parenchymal disease, the energy status of the liver decreases. This in turn leads to compensatory suppression of energy-consuming processes such as active ion transport and protein and nucleic acid synthesis [128]. The latter is important for liver cell proliferation, which is a key feature of liver regeneration. Assessment of the energy state of the liver therefore provides direct information on the liver functional reserve.
The liver functional reserve can be estimated by measurement of ketone bodies, which reflect the redox state in liver mitochondria (i.e., the site of ATP production) [129]. These are determined by the redox tolerance index (RTI), which is reflected in a 100-fold cumulative enhancement of ketone body ratio relative to glucose level (100 × AKBR/A glucose) [129]. Furthermore, it can be estimated by 31-phosphorus (31P) magnetic resonance spectroscopy. The naturally abundant 31P isotope constitutes an important element in molecules such as tri- and diphosphate nucleotides that play a central role biological energy metabolism [130, 131].
13C-Methacetin Breath Test, LiMAx
There is a broad spectrum of 13C-breath tests available. The principle of the 13C-methacetin breath (LiMAx) test is based on the activity of cytochrome P450 1A2 (CYP1A2) system, an enzyme system that is exclusively expressed in the liver. The activity of this enzyme system proved to be reduced in patients with severe chronic liver disease, regardless of cholestasis [132]. CYP1A2 is distributed through the whole functional unit of the liver [133], and is not affected by drugs or genetic variation [133]. 13C-methacetin, the agent used to measure the activity of CYP1A2, is exclusively metabolized by the CYP1A2 [134]. 13C-methacetin is instantly metabolized into paracetamol and 13CO2, after which 13CO2 is excreted through the lungs. This causes alternations in the normal 13CO2/12CO2 ratio in patients’ breath [135]. In this manner, the 13C-methacetin breath test provides quantitative information on hepatic function.
After a minimum of 6 h fast, the base line of 13CO2/12CO2 ratio is measured. Subsequently, 2 mg/kg body weight (BW) 13C-labeled methacetin is intravenously administered to the patient. Changes in the 13CO2/12CO2 ratio are analyzed by a modified, non-dispersive, isotope-selective infrared spectroscopy-based device during 60 min after injection of the agent. The expired air is collected using a specially designed face-mask. The results are expressed as μg/kg/h [136].
The LiMAx test is a non-invasive and easy to perform test which makes it an attractive option in clinical practice. The cut-off value of normal LiMAx readout is set at 311–575 μg/kg/h [136]. While LiMax assesses total liver functional capacity, the test can be used to measure the FRL function by combining LiMAx test with CT-volumetric analysis of FLR [136]. The authors assume that the percentage of liver function attributed to the FLR equals the percentage of FLR volume; however, this method does not take into account regional differences in liver function. On the other hand, preoperative FLR LiMAx values correlated with the LiMAx values measured on the first postoperative day. LiMAx value on postoperative day 1 has also been described as a predictor of post-resectional liver failure and liver failure related mortality. The same research group proposed a decision tree based on the LiMAx results which is supposed to help the surgeon to decide between resection and alternative or additional therapies such as PVE, neoadjuvant treatment, and palliative therapy [137]. The value of this algorithm and the proposed cut-off values await further clinical assessment in a prospective setting.
The LiMAx test has also been proposed as a tool in the monitoring of functional recovery after hepatic resection. Test readouts showed that functional recovery of the liver remnant was completed significantly faster compared to volumetric recovery. With this knowledge, the authors suggested tailored management for patients with sufficient recovery [138]. Because this test is based on the activity of an enzyme system, it is uncertain, however, if the readouts are influenced by the resection. In order to validate LiMAx in this setting, the expression of the enzyme system should be investigated.
The LiMAx test has recently been explored in patients undergoing PVE [139]. In this study, the LiMax was used to visualize the changes in FRL function in the time between PVE and major liver resection, showing an increase in FLR function after PVE. Furthermore, they found that function of FLR post-resection was lower in comparison to the preoperatively calculated function, which they explain as loss of function due to intraoperative injury. The authors plead that an overestimation-margin of the FLR is needed preoperatively in order to compensate for this loss, which is an interesting point that could contribute to safety management in liver surgery, especially in patients who are scheduled for complex resections.
The major limitation of the 13C-breath tests is the assumption that the contribution of FLR to total liver function is equal to the proportion of FLR to total liver volume. Malinowski et al. advocate in their study that the distribution of liver function does not change after PVE [139]. The FLR function measured with LiMax shortly after PVE did not differ from FLR function measured before PVE. Furthermore, they found that overestimation of FLR function preoperatively was not different between PVE and non-PVE patients. However, both arguments attest to the fact that the test is based on indirect measurement of liver function. Inhomogeneous distribution of liver function throughout the liver has been demonstrated using scintigraphic methods [105, 140, 141] and MRI as well [142].
Another difficulty in the application of the Limax test is that the test results are potentially affected by several factors such as hemodialysis, smoking, nutrition, and visceral hemodynamics [137]. Also, members of the CYP1A family are considerably downregulated in hepatocellular carcinomas, rendering the test less universal in use for the whole population of patients requiring liver resection [143].
The greatest advantage of the LiMax test is its non-invasive character. This permits a more intensive frequency of measurement of total liver function in the setting of prospective studies. Currently, little is known of the changes in total liver function in the course of the work-up before resection, e.g., neoadjuvant chemotherapy. Using Limax, a mild impairment of liver function has been shown [144]; however, this study should be repeated in a lager cohort of patients before any conclusions can be drawn.
Assessment of Liver Function Using Magnetic Resonance Imaging
Magnetic resonance imaging (MRI) with gadolinium ethoxybenzyl diethylenetriaminepentaacetic acid (Gd-EOB-DTPA) is well established as a liver imaging technique. MRI provides accurate anatomical information and has recently also been introduced as a potential technique for preoperative assessment of liver function [145–147]. The use of contrast-enhanced MRI (CE-MRI) with gadolinium-based contrast agents allows more accurate depiction of benign or malign liver lesions than with CT [148]. Contrast-enhanced MRI is already part of the standard preoperative work-up in patients scheduled for major liver resection in various centers over the world.
Gd-EOB-DTPA is a liver-specific contrast agent. Approximately 50% of the circulating agent is excreted by the hepatocytes. The excretion of the remaining 50% is managed by the kidneys. The uptake of Gd-EOB-DTPA from the liver sinusoids is managed by the OATPs and the NTCPs [149–153], while the MRP2 excrete Gd-EOB-DTPA into the bile canaliculi [154, 155]. Excretion occurs without prior biotransformation. The pharmacokinetic properties of Gd-EOB-DTPA, including the uptake and excretion transporter proteins, are similar to those of mebrofenin as used in 99mTc-HBS, suggesting that this technique is of potential use in the assessment of liver function.
The concept of using CE-MRI with Gd-EOB-DTPA in the evaluation of liver function was first introduced in 1993 [151]. Subsequently, several studies have been published showing correlation between MR imaging with Gd-EOB-DTPA and liver function in an animal model [156–161]. Recently, data on assessment of liver function using MRI with Gd-EOB-DTPA in humans have been published, all of them confirming the possibility of liver function assessment using MRI [162–169].
In a preliminary study, Saito et al. retrospectively reviewed data of 28 patients who had undergone several quantitative functional tests as well as a standard 5-phase CE-MRI with Gd-EOB-DTPA during work-up for liver resection [147]. They compared the intracellular contrast agent uptake rate and extracellular volume with the results of ICG and GSA tests, and found statistically significant correlations between the uptake rate and the reference tests. These data indicate that Gd-EOB-DTPA CE-MRI, even in its simplest form, may already be of use for estimation of liver function. Future studies should target the additional value of dynamic contrast enhanced MRI. This would allow a more thorough analysis of the time versus signal intensity curve, as more data are acquired during and after administration of the contrast agent.
Functional imaging with MRI-Gd-EOB-DTPA facilitates assessment of total and regional liver function in a similar way as scintigraphic modalities [169]. The latter, however, require additional CT imaging examinations in order to reach sufficient resolution which forms an additional burden for the patient. Since MRI does not use ionizing irradiation, the patient burden is lower. Furthermore, CT imaging used in combination with scintigraphic methods is usually insufficient for diagnostic purposes, while MR imaging provides high-quality information that can be used in the preoperative work-up of the patient. Given that MRI now allows the segmental assessment of steatosis and can be used to assess fibrosis, this makes it a potential one-stop-shop modality for both liver anatomy and function [170–173]. Another advantage is that Gd-EOB-DTPA uptake is reliable in patients with and without compromised liver parenchyma [162, 166, 167]. Hence, although the use of MRI with Gd-EOB-DTPA for liver function assessment is still under investigation, the evidence up to now shows promising results, and offers the attractive prospect of combining diagnostic and functional imaging in one procedure.
Discussion
Improvement of short-term and long-term survival after extensive liver surgery has been the main focus of liver surgeons during the last two decades. Modern surgical techniques have not only contributed to the reduction of procedure-related morbidity and mortality, but have also led to undertaking more extensive and even extreme hepatic resections in specialized centers. In parallel with these developments, postoperative liver failure has remained the most feared complication, as the treatment options are very limited and outcome often turns out to be lethal. Accurate preoperative assessment of FLR is essential in order to foresee postoperative liver dysfunction and to install alternative strategies, such as resection after portal vein embolization or two-stage resection.
In patients with liver-specific diseases, accurate assessment of liver function is critical for the selection of treatment options. Treatment of HCC in cirrhotic patients, i.e., by liver resection or transplantation, is determined by the severity of underlying liver disease. In cirrhosis, fibrosis is accompanied by a reduction of functional hepatocytes that is characterized by fibrous tissue septa that separate hepatocyte nodules, leading to altered resistance to blood flow in the liver and portal hypertension [174, 175]. The most commonly used liver function tests in cirrhotic patients include plasma aminotransferases, bilirubin clearance, albumin levels, PT, HA uptake, the Child–Pugh classification, and the ICG test.
Liver steatosis and steatohepatitis are associated with an increased risk of partial liver resection of intrahepatic tumors, especially after neo-adjuvant chemotherapy, or in living donor liver transplantation [3]. When CT volumetry is used as a prognostic tool for surgical outcome, a functional overestimation can be made in patients with steatosis. The accumulation of triacylglycerols in hepatocytes leads to hepatocyte enlargement in combination with steatosis-induced perfusion defects; i.e., phenomena that distort the actual liver function when deduced from CT scans. Increases in liver fat infiltration reduce liver blood flow and hepatic microcirculation, which in turn affect the extent to which molecules such as ICG can reach hepatocytes. ICG clearance and 99mTc-mebrofenin HBS therefore possess the potential to assess hepatic function in steatotic livers, because of the combination of impaired parenchymal perfusion and liver dysfunction [176].
Prolonged cholestasis produces hepatocellular injury and fibrosis. The uptake of 99mTc-mebrofenin and ICG is impaired under these conditions, due to competitive uptake of bilirubin and ICG/mebrofenin by the same cellular transporter systems. Although this impaired uptake still reflects the uptake function of the liver at that specific time, it does not represent the function of the liver after surgery once the biliairy obstruction is resolved. Preoperative assessment of liver function using the ICG clearance test or 99mTc-mebrofenin HBS therefore requires complete biliary drainage in patients, with concomitant obstruction of (part of) the biliary tree, as seen in hilar cholangiocarcinoma. Alternatively, when percutaneous transhepatic biliary drainage has been performed, ICG or mebrofenin excretion can be measured directly in the drained bile.
The current gold standard, CT volumetry, uses volumetric parameters in the prediction of post-resectional outcome. However, FLR volume does not necessarily correlate with FLR function, especially in patients with a compromised liver parenchyma. Three quantitative liver function tests, i.e., 99mTc-GSA, 99mTc-mebrofenin HBS, and the LiMAx test, have shown a discrepancy in functional versus volumetric increase after PVE. From this we can assume that judgement of FLR should not be based on volumetric parameters only. Furthermore, routine preoperative liver biopsy is considered controversial due to possible complications and a high probability of sampling errors. Given the fact that the quality of FLR parenchyma remains unknown until the resection specimen has been examined, additional quantitative liver function tests are advised in the preoperative selection of patients for major resection, or for timing of resection after preoperative PVE. The exception obviously is the patient with FLR volume that greatly exceeds the minimum volume and in whom no parenchymal disease is anticipated.
The ICG clearance test was the first quantitative liver test to be introduced. Even though it has found wide applicability in liver surgery, it is reliable for preoperative assessment of liver function only in a select patient population (with cirrhosis) which makes the ICG clearance test less universally applicable. With this knowledge, hepatobiliary surgeons should focus on newer methods that are able to overcome the shortcomings of the older methods.
As mentioned above, underlying parenchymal disease is one of two major challenges in the assessment of hepatic function, making most of the available tests less suitable in the overall patient population. 99mTc-GSA, 99mTc-mebrofenin scintigraphy, and possibly the LiMAx test have brought solutions for this problem. Both 99mTc-GSA and 99mTc-mebrofenin have been validated as preoperative liver function tests and correlated with post-resectional outcomes in several clinical studies involving patients with normal livers, as well as patients with parenchymal liver diseases.
The second major limitation of most quantitative liver function tests, such as the ICG clearance test and the LiMAx test, is the lack of accurate measurement of regional liver function, i.e., function of specifically the FRL. 99mTc-GSA and 99mTc-mebrofenin HBS can be performed together with a single proton emission computed tomography CT (SPECT-CT), which offers the possibility to obtain at the same time anatomical as well as functional information of the FLR. The information is crucial in the setting of hepatic surgery. The choice which of the scintigraphic methods is to be preferred for the preoperative assessment of FLR function depends on the facilities available. Although the two tests are based on different principles, both offer the possibility of measuring FLR function in both normal and compromised liver parenchyma, and are able to measure FLR function apart from total liver function. The only drawback of 99mTc-GSA is that it is not available in Western countries, whereas 99mTc-mebrofenin is inexpensive and freely available throughout the world. Both gamma camera and SPECT-CT possibilities are usually available in centers treating patients with hepatic disease, rendering implementation of the scintigraphic techniques less demanding.
Future opportunities in preoperative liver function assessment possibly lie in the field of MRI. The absence of radiation burden and the multi-purpose character of MRI potentially replace current quantitative liver function tests and CT volumetry, reducing costs at the same time. The similarity between the kinetics of scintigraphic agents and contrast agents used with MRI encourages further investigation of functional MRI, Notwithstanding the outlook on new modalities, the current quantitative liver function tests offer a chance to reduce postoperative liver failure, and therefore should be implemented in the regular preoperative work-up of patients considered for major liver resection.
Because of the complexity of liver function, one single test cannot represent overall liver function and accurately predict operative risk in any given patient considered for major liver resection [177, 178]. We still rely on the combination of clinical parameters and quantitative liver function tests to estimate liver functional reserve and to decide whether we can perform a safe resection in any patient presented to us. Scoring methods need to be developed in which clinical parameters, CT volumetric criteria, and the results of dynamic quantitative liver function tests guide our decision-making in patients requiring major liver resection [59]. Objective functional criteria are necessary to define patients at increased risk. Until appropriate scoring methods and objective functional criteria have become available, multiple tests measuring different components of liver function should be combined for the optimal assessment of liver function.
In conclusion, liver function involves a spectrum of metabolic functions, and there is not one test that can measure all functions at the same time. Laboratory blood assays and clinical scoring systems are unreliable in predicting post-resectional outcomes. Quantitative liver function tests mostly provide information on global liver function. Scintigraphic methods such as 99mTc-GSA and 99mTc-mebrofenin HBS in combination with SPECT permit regional assessment of specifically, the FLR. MRI using Gd-EOB-DTPA has potential as a combined diagnostic and functional imaging technique in patients considered for liver resection. The ideal method for evaluation of liver function and surgical risk in patients considered for extreme liver resection should combine clinical parameters, volumetric data, and the results of dynamic quantitative liver function tests.
References
1.
van den Broek MA, Olde Damink SW, Dejong CH, et al. Liver failure after partial hepatic resection: definition, pathophysiology, risk factors and treatment. Liver Int. 2008;28:767–80.PubMed
2.
Cucchetti A, Cescon M, Ercolani G, et al. Safety of hepatic resection in overweight and obese patients with cirrhosis. Br J Surg. 2011;98:1147–54.PubMed
3.
Vetelainen R, van Vliet A, Gouma DJ, et al. Steatosis as a risk factor in liver surgery. Ann Surg. 2007;245:20–30.PubMedPubMedCentral
4.
Cieslak KP, Runge JH, Heger M, et al. New perspectives in the assessment of future remnant liver. Dig Surg. 2014;31:255–68.PubMed
5.
Eriksson S, Fraser JR, Laurent TC, et al. Endothelial cells are a site of uptake and degradation of hyaluronic acid in the liver. Exp Cell Res. 1983;144:223–8.PubMed
6.
Fraser JR, Alcorn D, Laurent TC, et al. Uptake of circulating hyaluronic acid by the rat liver. Cellular localization in situ. Cell Tissue Res. 1985;242:505–10.PubMed
7.
Schindl MJ, Millar AM, Redhead DN, et al. The adaptive response of the reticuloendothelial system to major liver resection in humans. Ann Surg. 2006;243:507–14.PubMedPubMedCentral
8.
Demetriou AA, Brown Jr RS, Busuttil RW, et al. Prospective, randomized, multicenter, controlled trial of a bioartificial liver in treating acute liver failure. Ann Surg. 2004;239:660–7.PubMedPubMedCentral
9.
Van de Kerkhove MP, Hoekstra R, Chamuleau RA, et al. Clinical application of bioartificial liver support systems. Ann Surg. 2004;240:216–30.PubMedPubMedCentral
10.
Field KM, Dow C, Michael M. Part I: Liver function in oncology: biochemistry and beyond. Lancet Oncol. 2008;9:1092–101.PubMed
13.
Lockwood AH, McDonald JM, Reiman RE, et al. The dynamics of ammonia metabolism in man. Effects of liver disease and hyperammonemia. J Clin Invest. 1979;63:449–60.PubMedPubMedCentral
14.
Engstrom-Laurent A, Loof L, Nyberg A, et al. Increased serum levels of hyaluronate in liver disease. Hepatology. 1985;5:638–42.PubMed
15.
Frebourg T, Delpech B, Bercoff E, et al. Serum hyaluronate in liver diseases: study by enzymoimmunological assay. Hepatology. 1986;6:392–5.PubMed
16.
Guechot J, Laudat A, Loria A, et al. Diagnostic accuracy of hyaluronan and type III procollagen amino-terminal peptide serum assays as markers of liver fibrosis in chronic viral hepatitis C evaluated by ROC curve analysis. Clin Chem. 1996;42:558–63.PubMed
17.
Garcea G, Ong SL, Maddern GJ. Predicting liver failure following major hepatectomy. Dig Liver Dis. 2009;41:798–806.PubMed
18.
Nagashima I, Takada T, Okinaga K, et al. A scoring system for the assessment of the risk of mortality after partial hepatectomy in patients with chronic liver dysfunction. J Hepatobiliary Pancreat Surg. 2005;12:44–8.PubMed
19.
Schneider PD. Preoperative assessment of liver function. Surg Clin North Am. 2004;84:355–73.PubMed
20.
Freeman Jr RB, Wiesner RH, Roberts JP, et al. Improving liver allocation: MELD and PELD. Am J Transplant. 2004;4(Suppl. 9):114–31.PubMed
21.
Inaba K, Barmparas G, Resnick S, et al. The model for end-stage liver disease score: an independent prognostic factor of mortality in injured cirrhotic patients. Arch Surg. 2011;146:1074–8.PubMed
22.
Kamath PS, Kim WR. The model for end-stage liver disease (MELD). Hepatology. 2007;45:797–805.PubMed
23.
Schroeder RA, Marroquin CE, Bute BP, et al. Predictive indices of morbidity and mortality after liver resection. Ann Surg. 2006;243:373–9.PubMedPubMedCentral
24.
Cholongitas E, Marelli L, Shusang V, et al. A systematic review of the performance of the model for end-stage liver disease (MELD) in the setting of liver transplantation. Liver Transpl. 2006;12:1049–61.PubMed
25.
Heymsfield SB, Fulenwider T, Nordlinger B, et al. Accurate measurement of liver, kidney, and spleen volume and mass by computerized axial tomography. Ann Intern Med. 1979;90:185–7.PubMed
26.
Shoup M, Gonen M, D’Angelica M, et al. Volumetric analysis predicts hepatic dysfunction in patients undergoing major liver resection. J Gastrointest Surg. 2003;7:325–30.PubMed
27.
Kubota K, Makuuchi M, Kusaka K, et al. Measurement of liver volume and hepatic functional reserve as a guide to decision-making in resectional surgery for hepatic tumors. Hepatology. 1997;26:1176–81.PubMed
28.
Shirabe K, Shimada M, Gion T, et al. Postoperative liver failure after major hepatic resection for hepatocellular carcinoma in the modern era with special reference to remnant liver volume. J Am Coll Surg. 1999;188:304–9.PubMed
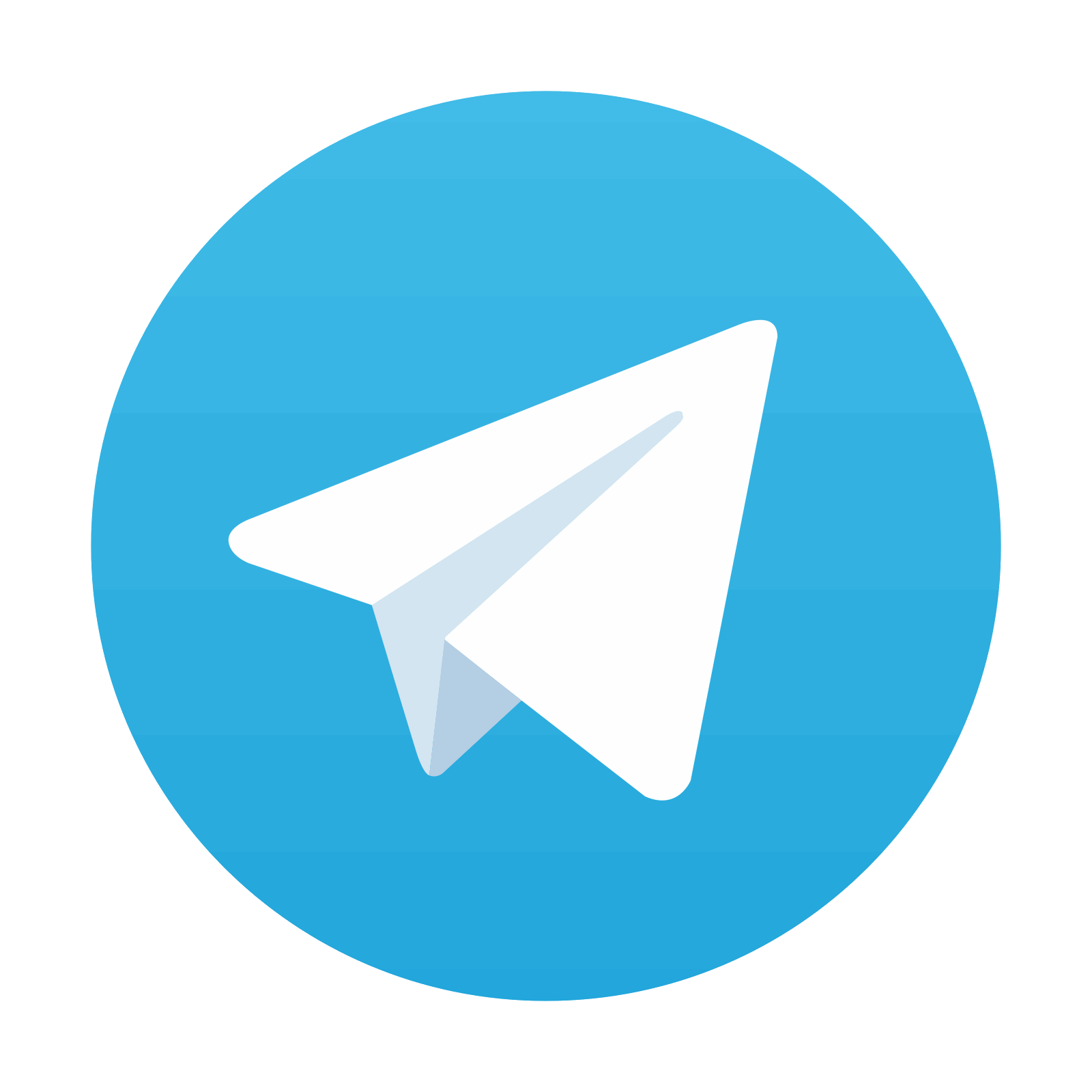
Stay updated, free articles. Join our Telegram channel

Full access? Get Clinical Tree
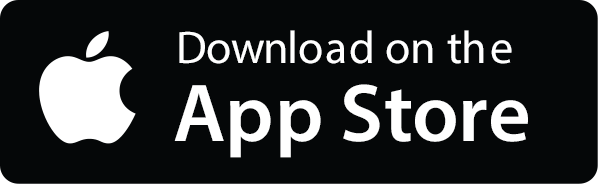
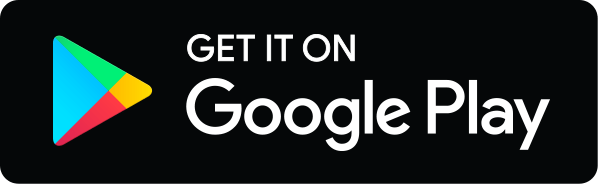