Fig. 3.1
Spinal cord neurons. The afferent and efferent neurons of the spinal cord and relation with interneurons
Specific areas in the brain which are involved in control of micturition include the pontine micturition center, the periaqueductal gray, and also several parts of the cerebral cortex which influence voluntary control. All these areas are interconnected with each other, as well as to relevant regions in the spinal cord. The interneurons from the spinal cord are known to project to the pontine micturition center and periaqueductal gray and projections from the periaqueductal gray and hypothalamus are known to pass to the pontine micturition center [2, 6]. At the peripheral nervous system pathway, the parasympathetic outflow from the sacral segments S2, S3, and S4 involved in the voiding function travels via the pelvic nerves, while the sympathetic from T10, T11, T12, L1, and L2 segments involved in the storage phase of the bladder travels via the hypogastric nerves.
Bladder Filling
Bladder filling is associated with storage reflexes, which are organized primarily at the spinal cord level. Filling is associated with inhibition of parasympathetic stimulation to the detrusor muscle, plus activation of the smooth and striated muscles of the urethral sphincter. This coordinated activity is thought to be mediated by urethral reflexes collectively known as the “guarding reflex,” which is activated by afferent feedback from bladder stretch receptors conveyed via the pelvic nerves [2]. Studies in feline models have demonstrated input of afferent neurons into the periaqueductal gray, which in turn provides inputs into the pontine micturition center [7]. The periaqueductal gray is likely to act as a relay between higher brain centers and the pontine micturition center, allowing modulation of the excitatory input into the pontine micturition center. Functional brain imaging studies in humans have demonstrated that activity in the periaqueductal gray increases during bladder filling and when bladder afferent activity increases [8, 9]. During bladder filling, the activity of parasympathetic efferent neurons to the bladder is downregulated, i.e., turned off [10]. In short, with bladder filling sympathetic activity is increased, parasympathetic activity is inhibited, and the somatic neurons are activated (Fig. 3.2). At a critical level of distension, afferents from tension receptors in the bladder stimulate the parasympathetic pathway to be “switched on” to maximal activity [11]. Despite these involuntary changes in activity, activation of micturition is under strict voluntary control.


Fig. 3.2
Bladder storage reflexes – simplified schematic representation. Bladder filling with stimulation of bladder stretch receptors (SR) associated with inhibition of detrusor and activation of urethral outlet (PAG periaqueductal gray, PMC pontine micturition center)
Voiding
The periaqueductal gray (PAG) integrates the voiding process from bladder afferents and spinal pathways to the pontine micturition center and frontal cortex. PAG is involved in the registration and conscious awareness of bladder filling sensations and in the manipulation of firing of the voiding reflex at the appropriate time [6]. The PAG also receives projections from higher brain centers and modulates primary input into the pontine micturition center, allowing excitation only when voiding is consciously desired.
Meticulous PET functional brain imaging studies have confirmed the activation of a well-defined area in the dorsal pons, which becomes active during the initiation of voluntary voiding [8]. Clinical and functional imaging studies suggest that in humans, the frontal lobes play an important part in assessing the appropriateness of micturition [2]. The prefrontal cortex is thought to be an area central to planning complex behaviors and appropriate social behavior, as well as response selection mechanisms [12]. This region is directly connected to the periaqueductal gray, which suggests that it may be responsible for inhibition of micturition until an individual is in a situation where voiding is wanted and socially appropriate [13] (Fig. 3.3).


Fig. 3.3
Voiding reflexes – simplified schematic representation. After appropriate bladder distension, inhibition of bladder outlet and the sympathetic pathway with activation of parasympathetic and detrusor contraction
There are certain prerequisites which are necessary in order for a void to take place, such as an appropriate degree of bladder distension (i.e., afferent signals from the bladder of sufficient strength to trigger output from the periaqueductal gray) and a socially appropriate and safe situation in which to void (influenced by signaling from the cerebral cortex and hypothalamus) [14]. Once these prerequisites are met, neuronal input from the periaqueductal gray leads to excitation of the pontine micturition center. Voluntary voiding seems to be associated with interruption of the tonic suppression of the PAG input into the pontine micturition center. This in turn activates descending pathways leading to urethral relaxation (via a parasympathetic-mediated release of nitric oxide, which decreases adrenergic and somatic cholinergic and excitatory signaling to the urethra), followed by activation of parasympathetic outflow from the sacral nerves. Functional brain imaging studies have indicated that voiding is associated with activation of a variety of structures including the prefrontal cortex, the insula, the hypothalamus, and the periaqueductal gray [15, 16].
Role of Detrusor Muscle
The detrusor smooth muscle is comprised of small spindle-shaped cells with a central nucleus [17]. Although isolated detrusor strips can show spontaneous mechanical activity, spontaneous fused tetanic contractions (such as those seen in the uterus) are generally not seen in normal bladders. These, together with experiments which have measured the tissue impedance of detrusor muscle, suggest that the detrusor is characterized by poor electrical coupling between the smooth muscle cells [18]. This feature is likely to be necessary in order to prevent synchronous activation of detrusor smooth muscle cells during bladder filling [19]. Indeed, ultrastructural studies of human detrusor muscle have shown that although coupling of smooth muscle cells does occur in asymptomatic volunteers, it is upregulated in patients with urgency symptoms to form limited functional syncytia, suggesting increased intracellular signaling in the detrusor smooth muscle of individuals with detrusor overactivity (DO) [20–22]. Other histological studies have additionally found patchy denervation of the detrusor muscle together with potassium supersensitivity in women with DO compared to healthy controls [23]. There is also evidence that calcium handling in detrusor smooth muscle cells in those with DO is altered, leading to a higher level of intracellular calcium [24, 25].
Localized distortions of the bladder wall due to autonomous activity may contribute to increased bladder sensations and urgency in women with overactive bladder by stimulating sensitive tension receptors in the detrusor smooth muscle. These localized distortions are known as “micromotions.” Physiological experiments in women with increased bladder sensation have shown a higher prevalence of micromotions in the detrusor smooth muscle compared to women with normal bladder sensation [26]. Micromotions can be multifocal with separate areas of the bladder wall contracting independently as modules [27]. This localized activity is thought to stimulate afferent activity in ascending neurons and may therefore lead to urgency, frequency, and reduced functional bladder capacity. A population of cells in the detrusor known as interstitial cells (or myofibroblasts) have been suggested to act as “pacemaker” in the bladder, with respect to the regulation of this spontaneous activity [28]. Interstitial cells in the detrusor are known to be spontaneously active and also stimulate calcium influx in response to muscarinic receptor activation. Interstitial cells are also found in the suburothelium, where they are closely related to C-fiber nerve endings in the submucosal layer of the bladder. It is likely that bladder distension during filling leads to release of cytokines such as adenosine triphosphate (ATP) and acetylcholine (Ach), which activate afferent neurons in the suburothelium via the action of the suburothelial interstitial cells [19].
Role of the Urothelium
The central nervous system reflexes have an overall controlling influence on bladder function. In addition, the afferent nerve output, local mediators and cells found within the urothelium and suburothelium also have a profound influence on the bladder.
Afferent neurons returning from the bladder travel in the pudendal, pelvic, and hypogastric nerves and are comprised of thin myelinated Aδ fibers and nonmyelinated C fibers. The primary afferent neurons of the pelvic and pudendal nerves are contained in the sacral dorsal root ganglia, with afferent innervation of the hypogastric nerves arising in the lumbar dorsal root ganglia. The central axons of the dorsal root ganglia in turn convey sensory information from the lower urinary tract to second order neurons within the spinal cord [29, 30].
The Aδ fiber endings are in the smooth muscle and are the most sensitive nerve endings in the bladder, being activated by increasing bladder wall tension secondary to intravesical filling. The C fibers have their endings in the lamina propria and urothelium and are only activated at greater degrees of bladder distension than Aδ endings (Fig. 3.4). C fibers are activated also by neurotransmitters and mediators in pathological situations such as inflammation and high osmolality [31]. Thus, C fibers may be of more importance in women with pathological urgency and OAB. C-fiber receptors such as the transient receptor potential vanilloid 1 (TRPV1) and P2X3 (ATP receptor) are modulated by mediators such as nerve growth factor (NGF) and are believed to be of importance in afferent activation in the bladder in women with sensory urgency, although there is conflicting evidence on their role in women with DO [32, 33].


Fig. 3.4
Schematic representation of bladder urothelium, interstitial and smooth muscle cells. Myelinated A delta fibres and unmyelinated C-fibre endings depicted
The source of the initiating stimulus to the afferent fibers is thought to be the urothelium [34]. The cells comprising the urothelium are in close proximity to the afferent nerves and activity in this layer is likely to be intimately involved in signal transmission and modulation, apart from its established barrier function. The urothelium is known to express a variety of ion channels and receptors which respond to chemical as well as mechanical stimuli, by releasing mediators which then stimulate afferent nerve fibers. A variety of urothelial mediators, thought to be of importance in afferent function and dysfunction, have been identified. Often the best way of understanding the normal role of these mediators is to understand their role in patients with abnormal bladder function. Patients with OAB are known to have higher levels of ATP, NGF, and prostaglandin E2 (PGE2), when compared to normal controls [35–37]. Some of the more important and better understood signaling pathways are discussed below.
Purinergic Pathways and ATP
ATP is known to facilitate the non-adrenergic, non-cholinergic component of bladder contraction. It acts on the P2X family of receptors, participating in efferent bladder signaling via the P2X1 receptor on detrusor smooth muscle membranes and in afferent signaling via the P2X2/3 receptors expressed on afferent neurons [38, 39]. Studies of P2X3 in knockout mice reveal detrusor hyporeflexia with a decreased frequency of voiding and an increased bladder capacity [40]. Patients with DO have been found to have substantially elevated levels of P2X3 receptors [41] and ATP [42]. Bladder distension as well as various physiological insults to the bladder stimulates ATP release [43], and the presence of ATP-degrading enzymes in rats is seen to prevent activation of P2X receptors and the manifestation of OAB symptoms [44]. The same study showed that intravesical introduction of ATP conversely induced DO. A study of human subjects undergoing urodynamic studies showed an inverse correlation between voided volume and levels of ATP in voided urodynamic fluid in both individuals with DO and normal controls and an inverse correlation between voided fluid ATP levels and first desire to void in those with DO, but not in the control group [45]. This suggests that abnormal purinergic signaling may be implicated in modulating early filling sensations in pathological states (Fig. 3.5).


Fig. 3.5
Signaling in urothelial pathways
Acetylcholine and Muscarinic Receptors
Acetylcholine (ACh) has been classically associated with motor function in the bladder, promoting detrusor contraction. In addition, there is a nonneuronal release of ACh from the urothelium which leads to modulation of sensory bladder function. This concept is reinforced by studies showing that antimuscarinic drugs exhibit activity during the bladder storage phase when efferent nerve pathways are silent [46]. The majority of experimental work proving the nonneuronal release of ACh has been carried out in animal models and has shown a distinct storage and release mechanism from the neuronal cells [47]. Yoshida and colleagues carried out a study using strips of bladder tissue from human subjects, demonstrating that removal of the urothelium substantially decreases release of ACh, as well as an age-related and stretch-induced increase in non-nerve-evoked ACh release [48]. Further studies using antimuscarinic drugs have suggested that blocking of muscarinic receptors reduces afferent stimulation. Studies examining the effect of oxybutynin on single bladder afferent units from rats have shown a desensitizing effect on C-fiber afferents [49, 50]. Similar results have been seen after administration of the M3-selective antimuscarinic darifenacin [51]. A subsequent study by Matsumoto and colleagues [52] suggested that it is M2 receptors that mediate cholinergic stimulation of afferent activity. An animal study using different dosing protocols for antimuscarinic drugs following carbachol-induced DO showed an increase in bladder capacity and inter-contraction interval, with no accompanying decrease in bladder contractility, suggesting a local inhibitory effect for antimuscarinic drugs. Collectively, the evidence suggests a role for muscarinic pathways in the modulation of afferent signaling, although the exact interaction is yet to be determined.
Nitric Oxide Pathways
Nitric oxide (NO) is synthesized within the urothelium and appears to act as an inhibitory transmitter within afferent pathways [53]. Intravesical administration of an NO substrate and an NO synthase inhibitor leads to inhibition and stimulation of both Aδ and C fibers, respectively. In support of this, in vivo studies have shown a decrease in bladder hyperactivity with administration of PDE5 inhibitors, which potentiate the NO/cyclic GMP pathway [54]. Intravesical administration of oxyhemoglobin, (which decreases NO concentration) to healthy rats leads to an increase in bladder pressure and a decrease in bladder capacity, with the converse being observed with administration of an NO substrate [55].
Adrenergic Pathways
The bladder storage phase is predominantly governed by the parasympathetic nervous system, which inhibits parasympathetic nerve-mediated detrusor contractions. Noradrenaline release induces detrusor relaxation via adrenergic β3 receptors [56] and β3 receptors are highly expressed in the bladder, particularly in the detrusor muscle and urothelium [57]. Administration of β3 receptor agonists to detrusor muscle strips leads to smooth muscle relaxation [58]. However, possible afferent effects are also suggested by studies showing that β3 agonists have an inhibitory effect on afferent nerve firing [59], as well as inducing urothelial NO release [60].
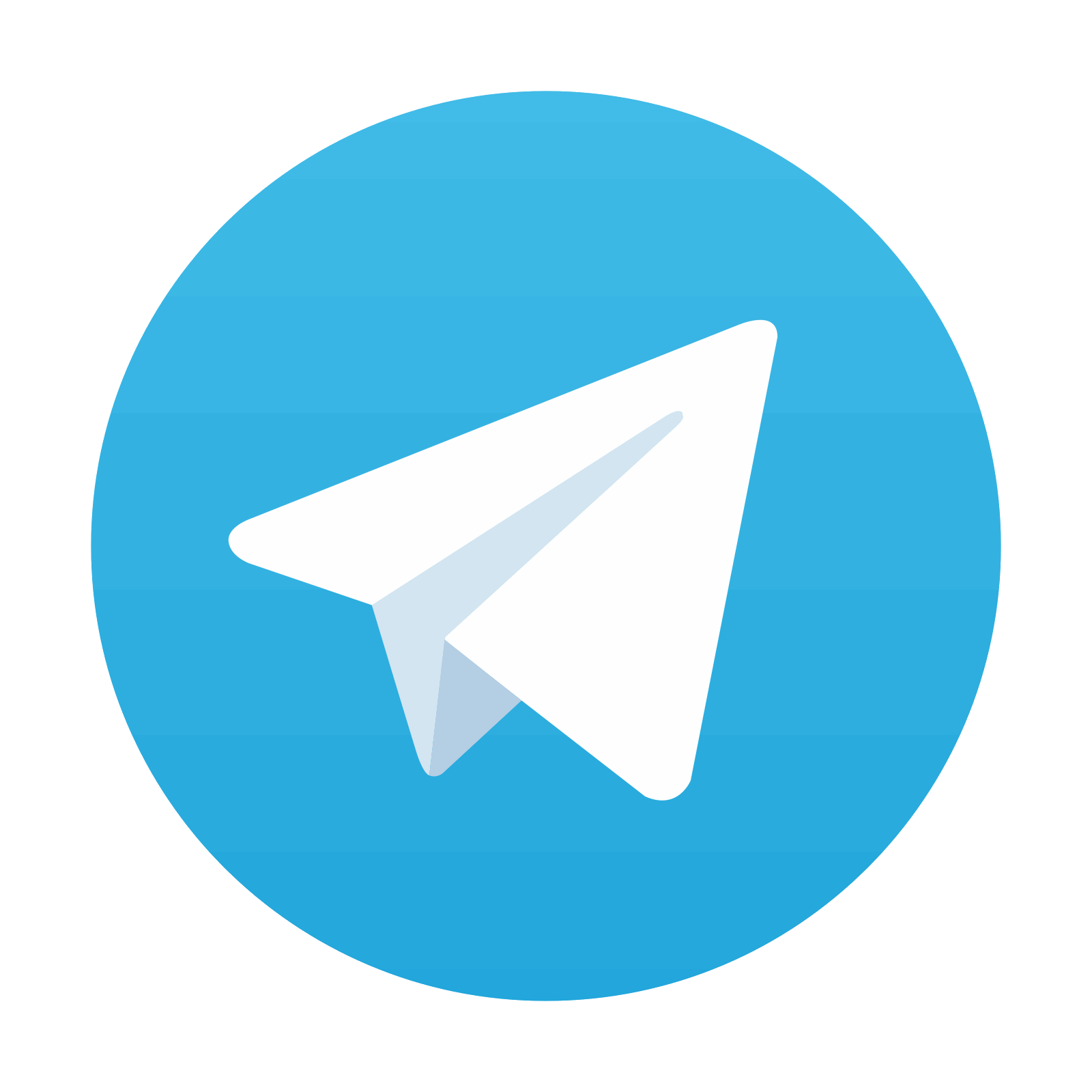
Stay updated, free articles. Join our Telegram channel

Full access? Get Clinical Tree
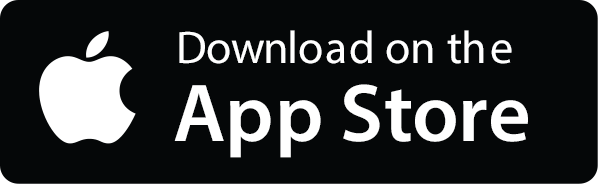
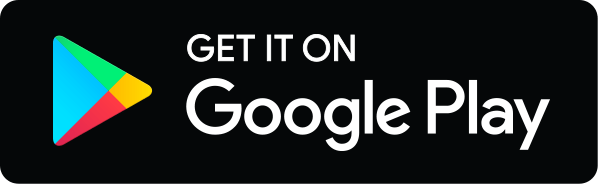