Key points
- •
Testosterone synthesis and male fertility are controlled by a negative feedback mechanism between the hypothalamus, the pituitary, and the testis.
- •
Congenital or acquired conditions leading to a failure of hormone synthesis or action at any level of the hypothalamic-pituitary-gonadal axis result in the clinical syndrome of hypogonadism.
- •
Medications and contemporary diseases are major causes of impairment of male reproductive function. Novel therapies may improve spermatogenesis along with elevating testosterone levels in men.
Introduction
Reproductive function changes markedly during life in humans. Impeccable coordination of the hypothalamic-pituitary-gonadal axis is required for normal testicular function in the male, including normal testosterone production and male fertility. Pulsatile secretion of gonadotropin-releasing hormone (GnRH) by the hypothalamus stimulates the biosynthesis of pituitary gonadotropins, luteinizing hormone (LH), and follicle-stimulating hormone (FSH) that, in turn, sustain intragonadal testosterone production and spermatogenesis. A negative feedback mechanism, controlled by sufficient levels of testosterone, is responsible for decreasing both hypothalamic GnRH secretion into the portal circulation and gonadotropin release from the pituitary into the bloodstream.
Congenital or acquired conditions leading to a failure of hormone synthesis or action at any level of the axis result in the clinical syndrome of hypogonadism. Hypogonadism may be caused either by a primary testicular disease or by a secondary (or central) cause (eg, a hypothalamic or pituitary disorder). In the setting of acquired hypogonadism, comorbidities and use of medications are common causes of low testosterone and must be ruled out before making the diagnosis.
Despite the cause, end organ replacement therapy with natural testosterone is recommended for chronic use but with the understood general caveat that this treatment does not improve fertility. If medications that stimulate hypothalamic or pituitary function are successful, these agents may be used for several-month intervals to enhance spermatogenesis; however, their long-term use needs further investigation. In addition, novel therapeutic agents have been proposed to stimulate both testosterone and spermatogenesis. The understanding of hypothalamic-pituitary-gonadal axis physiology is the first step for the correct diagnosis and treatment of hypogonadism, a frequent condition affecting quality of life and causing other comorbidities including osteoporosis in men.
This article reviews the physiology of the brain-hypothalamic-pituitary-gonadal axis, to correlate it to disorders that can induce male hypogonadism, and discusses available and potential future treatment modalities.
Introduction
Reproductive function changes markedly during life in humans. Impeccable coordination of the hypothalamic-pituitary-gonadal axis is required for normal testicular function in the male, including normal testosterone production and male fertility. Pulsatile secretion of gonadotropin-releasing hormone (GnRH) by the hypothalamus stimulates the biosynthesis of pituitary gonadotropins, luteinizing hormone (LH), and follicle-stimulating hormone (FSH) that, in turn, sustain intragonadal testosterone production and spermatogenesis. A negative feedback mechanism, controlled by sufficient levels of testosterone, is responsible for decreasing both hypothalamic GnRH secretion into the portal circulation and gonadotropin release from the pituitary into the bloodstream.
Congenital or acquired conditions leading to a failure of hormone synthesis or action at any level of the axis result in the clinical syndrome of hypogonadism. Hypogonadism may be caused either by a primary testicular disease or by a secondary (or central) cause (eg, a hypothalamic or pituitary disorder). In the setting of acquired hypogonadism, comorbidities and use of medications are common causes of low testosterone and must be ruled out before making the diagnosis.
Despite the cause, end organ replacement therapy with natural testosterone is recommended for chronic use but with the understood general caveat that this treatment does not improve fertility. If medications that stimulate hypothalamic or pituitary function are successful, these agents may be used for several-month intervals to enhance spermatogenesis; however, their long-term use needs further investigation. In addition, novel therapeutic agents have been proposed to stimulate both testosterone and spermatogenesis. The understanding of hypothalamic-pituitary-gonadal axis physiology is the first step for the correct diagnosis and treatment of hypogonadism, a frequent condition affecting quality of life and causing other comorbidities including osteoporosis in men.
This article reviews the physiology of the brain-hypothalamic-pituitary-gonadal axis, to correlate it to disorders that can induce male hypogonadism, and discusses available and potential future treatment modalities.
Anatomic overview of the hypothalamus and pituitary
The hypothalamus lies at the base of the brain, below the thalamus and the third ventricle, just above the optic chiasm and pituitary gland. It synthesizes and secretes certain neurohormones, often called releasing hormones or hypothalamic hormones , and these in turn stimulate or inhibit the secretion of pituitary hormones. The neurovascular link between hypothalamus and pituitary gland is the pituitary stalk, which comprises mainly neural and vascular components.
The pituitary gland, also known as the hypophysis , is located immediately beneath the hypothalamus, resting in a depression of the base of the skull called the sella turcica (Turkish saddle). The pituitary gland is entirely ectodermal in origin but is composed of 2 functionally distinct structures that differ in embryologic development and anatomy: the adenohypophysis (anterior pituitary) and the neurohypophysis (posterior pituitary). The adenohypophysis develops from an upward invagination of oral ectoderm named Rathke’s pouch , whereas the neurohypophysis derives from a downward extension of neural ectoderm, the infundibulum. Because the pituitary is just below the crossing of the visual nerves at the optic chiasm, pituitary tumors enlarging superiorly may affect superior temporal vision selectively.
The adenohypophysis is the manufacturer of an array of peptide hormones—gonadotropins (FSH and LH), adrenocorticotropin, growth hormone, prolactin, and thyroid-stimulating hormone (TSH)—and makes up roughly 80% of the pituitary gland. The release of these pituitary hormones is mediated by hypothalamic neurohormones that reach the adenohypophysis via a portal venous system.
Unlike the adenohypophysis, the neurohypophysis is not glandular and does not synthesize hormones. It stores and releases oxytocin and vasopressin, which are synthesized by neurosecretory cells of the hypothalamus. The neurohypophysis comprises axons of hypothalamic neurons; the neurohypophysis is, therefore, considered an extension of the hypothalamus.
Control of hypothalamic secretion
In recent years, kisspeptin, a 54-amino-acid peptide, encoded by the KiSS-1 gene, was identified. Kisspeptin activates the G protein–coupled receptor (GPR54) of the hypothalamus. During pregnancy, kisspeptin levels increase 7000 times. Human placenta secretes varying lengths of the peptide, but the C-terminal 10-amino-acid portion is sufficient to activate GnRH receptors in the fetus, initiating function of the hypothalamic-pituitary-gonadal axis. Kisspeptin also provides the major trigger for puberty. In rat studies, chronic infusion of kisspeptin triggers precocious puberty and enables pubertal development in undernourished animals.
Normal hypothalamic regulation of gonadotropins
Secretion of pituitary gonadotropic hormones is regulated by the hypothalamic decapeptide hormone, GnRH, which binds to a membrane receptor on pituitary gonadotrophs, stimulating synthesis and secretion of both FSH and LH, the 2 pituitary gonadotropic hormones ( Fig. 1 ).
Animal studies found that, in GnRH-deficient mice, pretreatment with GnRH led to both an increase in the gonadotropin content of the pituitary gland and an induction of the expression of pituitary GnRH receptors. Under certain physiologic conditions, GnRH receptor number varies and usually directly correlates with the gonadotropin secretory capacity of pituitary gonadotrophs.
Besides the number of GnRH receptors, pulsatile regimens of GnRH are required for the precision of pituitary gonadotropin signaling. GnRH pulsatility seems to be an intrinsic function of hypothalamic cells, dependent on calcium, with communication similar to nerve synapse conduction. Studies find a sequential response of gonadotropin secretion after exogenous GnRH administration in GnRH-deficient mice; there is an immediate and persistent increase in plasma FSH concentrations during the period of GnRH injections, whereas LH secretion requires a more prolonged and pulsatile GnRH therapy before LH is detected in the circulation. Furthermore, these important data indicate that FSH continues to be synthesized and stored even in the absence of sustained GnRH administration, but continued GnRH stimulation is required for LH synthesis.
Follicle-stimulating hormone, luteinizing hormone, and testicular function
FSH and LH are heterodimers with structural similarities; each consists of α and β peptide chain subunits, produced in the pituitary of both men and women. The α subunit is identical in both hormones; the β subunit, therefore, provides structural and biochemical specificity for receptor interactions and also determines the biologic specificity of the hormone. To date, hypogonadotropic hypogonadism (HH) owing to selective mutations in the FSH β or LH β subunit genes is rarely reported.
FSH is required for the determination of the testicular Sertoli cell number and for induction and maintenance of spermatogenesis. In testes with a sufficient level of testosterone, LH stimulates the secretion of gonadal steroids through Leydig cell activity. The same LH molecule stimulates estradiol synthesis in the ovary in women. By activating the FSH receptor, FSH has specific function within the Sertoli cells of the testis, resulting in an increase in cyclic adenosine monophosphate, thereby activating a cyclic adenosine monophosphate–dependent protein kinase. This increase, in turn, increases protein synthesis of androgen-binding protein (sex hormone–binding globulin [SHBG]) and the aromatase enzyme CYP19, which converts testosterone to estradiol.
Steroidogenesis, under the influence of LH acting through specific receptors found on the surface of the testicular Leydig cells, stimulates enzymatic conversion of precursor cholesterol into testosterone. Leydig cell secretion creates a high local concentration of testosterone in the testis; testosterone is also secreted into the circulation, with circulating testosterone levels occurring in a steep downhill concentration gradient from the testes, producing characteristic androgenic effects on distant androgen-sensitive target tissues. When testosterone levels are sufficient, the pituitary gland decreases the production and release of LH via a negative feedback mechanism, which also decreases GnRH and LH, thereby, decreasing testosterone levels. Apparently, much of the negative feedback regarding FSH occurs via the gonadal peptide hormones, inhibins, and activins, members of the transforming growth factor–β super family of molecules. Sertoli cells of the adult testes secrete both inhibins A and B, but the more important hormone is inhibin B, which suppresses FSH secretion, modulating the FSH stimulation by activins. Follistatins, produced within the pituitary, bind activins and further decrease their function. Beyond their negative pituitary feedback, inhibins function also throughout the reproductive hormonal axis and act locally as paracrine hormones within the testes.
Peripheral metabolism of testosterone
The testis contributes more than 95% of total circulating testosterone in the postpubertal man (the adrenal contributes the remainder). Testosterone is secreted into the circulation down a concentration gradient, where it equilibrates between protein-bound (98%) and free hormone (2%) fractions. Protein-bound testosterone binds either to low-affinity, high-availability proteins (primarily albumin) or to the high-affinity, low-abundance SHBG, a glycoprotein. The free hormone fraction is generally believed to be the biologically active form of testosterone. Once released in the bloodstream, testosterone reaches its peripheral sites of action, where it undergoes reversible and irreversible metabolism to other steroids with different activities ( Fig. 2 ).
Although only approximately 5% of serum testosterone produced in men undergoes 5α-reduction to dihydrotestosterone (DHT), many of the important functions of testosterone are mediated by this potent metabolite. Testosterone and DHT bind to a common androgen receptor; however, DHT has 2 to 3 times greater androgen receptor affinity than testosterone; moreover, the dissociation rate of testosterone from the androgen receptor is 5-fold faster than DHT. Also, DHT cannot be aromatized to estrogen. During embryogenesis, DHT has an essential role in the formation of the male external genitalia, whereas in the adult, DHT acts as the primary androgen in the prostate and in hair follicles. If there is insufficient conversion of testosterone to DHT, owing to deficient 5α-reductase enzyme, as in the genetic “penis at 14” syndrome (5α-reductase deficiency), the infant male will have a feminine appearance with small phallus and internal testes. However, after puberty, the penis enlarges and the male appearance ensues under the influence of mature testosterone levels. Interestingly, this gender transformation is completely accepted socially and psychologically in certain inbred communities of the Dominican Republic and Greece, and the adult male has normal reproductive function. (As a matter of fact, this issue is accurately addressed in the Pulitzer Prize-winning novel Middlesex, by Jeffrey Eugenides published in 2002.)
In the adult, as testosterone is converted to DHT, the prostate gland grows and male pattern hair loss proceeds. Understanding this biology, drug blockade with a 5α-reductase antagonist like finasteride may be used to decrease prostate size in benign prostatic hypertrophy and to slow male pattern balding. This drug is dangerous for a pregnant woman to use, as it would also impair the development of a phallus in the male fetus, similar to the block in the previously mentioned “penis at 14” syndrome.
In peripheral tissues, testosterone can also be converted to estrogens through the action of microsomal P450 enzyme CYP19 aromatase, expressed in many sites, including placenta, gonads, brain, and fat. More than 80% of circulating estradiol in men is derived from the aromatization of testosterone ; thus, male hypogonadism leads to a consequent hypoestrogenism. In men with deficient aromatization of testosterone to estrogen, there are high levels of testosterone, yet decreased levels of estrogen lead to increased levels of LH, a demonstration that lack of estrogen feedback to the pituitary is more important than feedback from testosterone. Together with evidence of the important role of estrogen in bone metabolism, Finkelstein and colleagues found that estrogens also play a fundamental role in the regulation of body fat and sexual function in men. On the other hand, in situations of fat accumulation, such as obesity or insulin resistance, the increased adiposity is associated with increased aromatase conversion of testosterone to estrogens in fat tissue, often resulting in gynecomastia, sometimes with lower levels of testosterone.
Sexual development in males
Pulsatile secretion of GnRH from the hypothalamus is required for the maintenance of reproductive function; however, the pattern of GnRH-induced gonadotropin secretion is constantly changing during sexual development. Neuroendocrine stimulation of the reproductive axis is initiated during fetal development. Although GnRH neurons are seen earlier in fetal life, the connection between these neurons and the portal system of the hypothalamus and pituitary becomes functional around 16 weeks of gestation. Hypothalamic GnRH neurons are functional at birth but, after the perinatal androgen surge, remain tonically suppressed during infantile life. During childhood, the hypothalamic-pituitary axis is characterized by low pulse frequency and low amplitude GnRH secretion.
The precise neuroendocrine trigger of puberty is not fully understood. Nevertheless, its onset is marked by sleep-entrained reactivation of the reproductive axis characterized by a remarkable increase in the amplitude of LH pulses with a lesser change in frequency. Initially, the nighttime elevation of LH secretion stimulates gonadal secretion of sex steroids, which return to prepubertal levels during the daytime. As puberty progresses, gonadotropins are secreted during both day and night, allowing sexual development to be completed. The testicular output of testosterone gains control over the hypothalamic discharge of GnRH, maintaining a constant frequency of LH pulses, and the sleep-entrained differences in testosterone and LH become negligible. By this time, the increased level of testosterone, along with its conversion to the active metabolite DHT, causes deepening of the voice, secondary sex hair distribution, enlargement of testicles, growth of the penis, and increased libido. Higher levels of testosterone itself, and by its conversion to estrogen, lead to development of muscle mass, bone maturation, and accelerated bone growth until closure of the epiphyses of long bones.
During adulthood, pulsatile GnRH continues to stimulate biosynthesis of LH and FSH that, in turn, sustain intragonadal testosterone production and spermatogenesis as well as systemic testosterone secretion and virilization.
Hypogonadotropic hypogonadism
Failure of the episodic GnRH secretion or action, or disruption of gonadotropin secretion, results in the clinical syndrome of HH. This condition can be caused by either pituitary or hypothalamic disorders and is referred to as secondary or tertiary hypogonadism, respectively. Secondary and tertiary HH can be distinguished from primary or testicular causes by the demonstration of low/normal gonadotropin levels in the setting of low testosterone concentration ( Table 1 ).
Primary (Increased LH/FSH, Low T) | Secondary (Normal/Low LH/FSH, Low T) | ||
---|---|---|---|
Congenital | Acquired | Congenital | Acquired |
Klinefelter syndrome Androgen synthesis disorder Mutation of FSH receptor gene Cryptorchidism Undescended testicles Varicocele Myotonic dystrophy | Chronic renal failure Hepatic cirrhosis Hemochromatosis Human immunodeficiency virus/AIDS Infections (eg, mumps orchitis) Radiation Trauma Toxins (eg, ethanol) Autoimmune damage Drugs | KS DAX-1 mutation GPR-54 mutation Leptin or leptin receptor mutation Prader-Willi syndrome Gonadotropin subunit mutation | Hyperprolactinemia Critical illness Chronic systemic illness Diabetes mellitus Obesity Neoplasms Infiltrative diseases (eg, sarcoidosis, histiocytosis, tuberculosis) Pituitary apoplexy Pituitary trauma Ethanol Drugs |
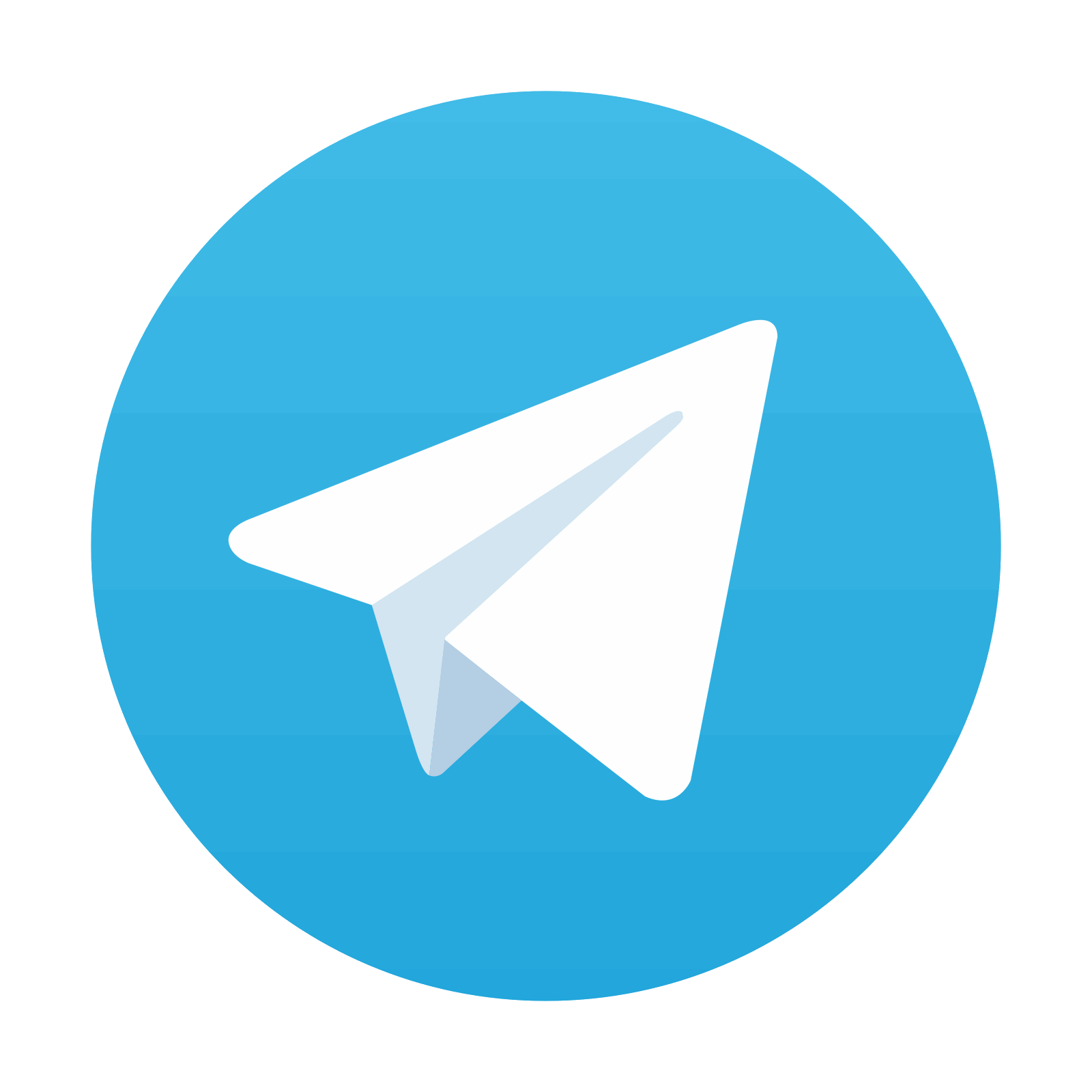
Stay updated, free articles. Join our Telegram channel

Full access? Get Clinical Tree
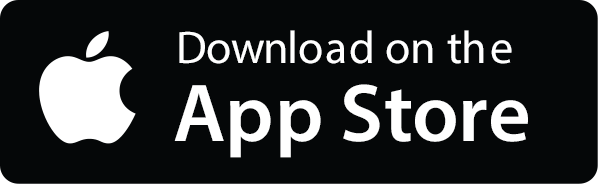
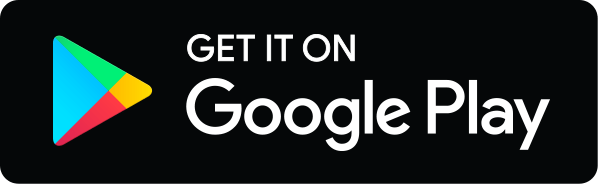
