Normal ET functions of pressure regulation, protection, and clearance within its normal system are necessary to maintain a healthy middle ear.
The chapter includes
• The functions of the tube related to the middle ear and mastoid gas (air) cell system: pressure regulation (ventilation), protection, and clearance.
• The physiologic gas composition of the middle ear as related to the pressure regulation function of the ET.
• The developmental, as well as daily cyclical, positional, and seasonal, changes related to physiology.
• The possible roles of the tympanic membrane, tensor tympani muscle, and mastoid gas cells in the physiology of the system.
• A comparison of the ET with the human larynx. The anatomy, physiology, and pathophysiology of the larynx have been the subject of intense investigation over the past century and are better understood than these aspects of the ET. Comparisons can be made between these two organs because they both develop from the airway and have similar functions, such as ventilation (pressure regulation of the tube), protection, and clearance, and the failures of these functions are, to some degree, also comparable.
The ET is not just a tube but an organ that is part of a system of organs. This chapter reviews our current understanding of the physiology (functions) of the tube within its system, which includes the nasal cavities, palate, and nasopharynx at the proximal (pharyngeal) end of the ET and the middle ear and mastoid gas cells at its distal end (Figure 3–1). Thus, the functions of the tube must be assessed within this system because the normal ET functions most effectively when the system at either end is also normal. The ET may function normally, but if any part of the rest of the system is abnormal, middle-ear disease can develop; for example, in the face of a normally functioning tube, if the tympanic membrane is not intact or there is persistent nasal or nasopharyngeal obstruction, middle-ear disease can occur. Thus, to maintain a healthy middle ear, the entire ET system should have normal function and structure. Knowledge of normal tubal function, within its system, is necessary to understand the pathophysiology of the system that can lead to middle-ear disease (see Chapter 5, “Pathophysiology”).
Physiology of the ET Within Its System
Three physiologic functions are attributed to the ET:
1. Pressure regulation (ventilation) of the middle ear that equilibrates gas pressure in the middle ear with atmospheric pressure
2. Protection of the middle ear from nasopharyngeal sound pressure and secretions
3. Clearance (drainage) of secretions produced within the middle ear into the nasopharynx (Figure 4–1)
Table 4–1 presents a classification of the physiology of the ET system.
Pressure Regulation
Of the three physiologic functions of the ET, the most important is regulation of pressure (ventilation) within the middle ear because hearing is optimal when middle-ear gas pressure is relatively the same as air pressure in the external auditory canal; that is, tympanic membrane and middle ear compliance is optimal.
ET Mechanics of Opening and Closing
Normally, the ET is passively closed. Intermittent active opening of the ET, which happens when the tensor veli palatini muscle contracts during swallowing, maintains nearly ambient pressures in the middle ear (see Chapter 3, “Anatomy”).1–4 Key experiments from our laboratory, employing the monkey, confirmed that active opening of the ET is related to the contraction of the tensor veli palatini muscle: when the tensor veli palatini was expunged or transected at the hamulus, contraction did not occur5; when botulinum was injected into the tensor through the palate, no tubal opening occurred3; and when the mandibular nerve was electrically stimulated at the base of the skull, the ET dilated (Figure 4–2).2 The role of the levator palatini muscle in opening the ET remains unclear, but we excised the palatal portion of the levator in the monkey and found no demonstrable change in normal ET tubal opening during manometric tubal ET function tests (Figure 4–3).6 We concluded that the levator palatini muscle does not dilate the ET; others have come to the same conclusion.7 Figure 4–4 shows the ET opening during active muscular dilation of the tubal lumen owing to the contraction of the tensor veli palatini muscle, which allows gas to pass in either direction depending on the pressures at either end of the tube.
FIGURE 4–1. Functions of the middle ear (ME)–mastoid (Mast) gas cell system. Pressure regulation function is related to active dilation of the tube by contraction of the tensor veli palatini muscle (TVP) (upper figure). Protective function is dependent, in part, on an intact middle ear and mastoid gas cells to maintain a gas cushion (middle figure). Clearance function is enhanced by mucociliary activity and muscular activity during tubal closing (lower figure). EC = external canal; NP = nasopharynx; TM = tympanic membrane.
TABLE 4–1. Classification of Physiology of ET System
Pressure regulation function |
Protection function |
Anatomic factors |
Immunologic and mucociliary defense |
Clearance function |
Mucociliary clearance |
Muscular clearance (“pumping action”) |
Surface tension factors |
FIGURE 4–2. The distribution of the various branches of the mandibular division of the trigeminal nerve in the rhesus monkey. These branches are (1) the nerve to the tensor veli palatini muscle, (2) the nerve to the internal pterygoid muscle, (3) the external pterygoid nerve, (4) the masseteric nerve, (5) the deep temporal nerve, (6) the auriculotemporal nerve, and (7) the condylar nerve. The letters A to E correspond to various electrode positions on the nerve. Reproduced with permission from Cantekin EI et al.2
FIGURE 4–3. Artist’s drawing of a cross section of the palate in the monkey in which the palatal portion of the levator palatini muscle was excised, which had no effect on ET opening during manometric function testing. This experiment failed to show any effect of the levator palatini on tubal opening. Reproduced with permission from Cantekin EI et al.
FIGURE 4–4. Illustration showing ET dilation (opening) owing to the contraction of the tensor veli palatini muscle (TVP) during swallowing, which allows equilibration of gas pressures within the middle ear (ME) with that of the nasopharynx (NP). EC = external auditory canal; Mast = mastoid gas cells.
As postulated by Sando and colleagues, the lateral lamina of the cartilage of the ET in the superior portion of the lumen most likely dilates the greatest during tensor veli palatini contraction, and the inferior portion of the tubal lumen is related more to the protective function with it mucosal folds, glands, and goblet cells.8,9 Figure 4–5 is an illustration of these hypotheses, which have been postulated following assessments of normal human temporal bone histopathologic specimens.
The sequence of tubal dilation has been assessed by radiography in the human and the animal.10–12 As shown in Figure 4–6, the normal ET is closed at rest and opens first in its proximal cartilaginous portion on swallowing owing to contraction of the tensor veli palatini muscle, which is then followed by distal tubal dilation. Following active dilation, the tube passively collapses to return to its resting position starting from the distal end and then to the proximal end, which is important for the muscular clearance function of the tube (see Clearance). Figure 4–6 shows a suggested spring-like action of ET active muscular opening and then passive closing owing to elastic recoil.
FIGURE 4–5. Illustration depicting the probable superior portion of the ET lumen opening during active dilation (DL) by the tensor veli palatini muscle (TVPM/TVP), which is attached to the lateral lamina (LL) of the cartilage. Sando and colleagues suggested that the superior portion (R) is related to pressure regulation during active dilation and the inferior portion (F) of the tubal lumen is related to protection with its folds, glands (G), and goblet cells (GL).8 Elastin in the hinge portion (i.e., junction between the medial and lateral laminae) aids in returning the medial lamina to the resting position. GL = glands in the folds of the inferior portion of the tubal lumen; ML = medial lamina of ET cartilage; OF = Ostmann’s fatty tissue (i.e., fat pad); R = superior portion of lumen at rest. Reproduced with permission from Sando I et al.8
Recently in our laboratory, Ghadiali and colleagues developed a modified forced-response test of ET function that correlates experimental pressure-flow rate measurements with a standard engineering model of flow in a collapsible tube.13 Correlation between model and experimental data yielded quantitative measurements of ET tubal compliance and hysteresis in the monkey. Using this engineering model of the ET, Ghadiali and colleagues considered a collapsible tube with global parameters of hyperesis and tubal elastance, which they considered to be dependent on interfacial and tissue mechanical properties (Figure 4–7).14 Various physical components, such as the mucosal surface condition and muscle tension, were evaluated in tubal mechanics.15 The investigators concluded that the engineering-based measure of compliance is more accurate than current summary parameters (i.e., tubal compliance index)16 and that knowledge of how specific physical components alter ET function may lead to improved treatments that target the underlying mechanical properties.17 Indeed, this team evaluated the effect of surfactant on tubal mechanics (see Clearance).14
Also from our laboratory, 6-year-old children without middle ear disease were recently tested in the pressure chamber which confirmed normal function.18
Gas Composition of the Middle Ear Related to the Pressure Regulation Function of the ET
As shown in Table 4–2, the gas in the nasopharynx that regulates pressure in the middle ear is 79.2% nitrogen, 14.7% oxygen, 1% argon, and 5.1% carbon dioxide. This is the same composition of the expiratory phrase of the respiratory cycle.19 Under the physiologic condition, fluctuations in ambient pressure are bidirectional (i.e., either to or from the middle ear), relatively small, and not readily appreciated.20,21 These fluctuations reflect the rise and fall in barometric pressures associated with changing weather conditions and elevation or both; however, changes in middle-ear pressure show mass directionality, can achieve appreciable magnitudes, and can result in pathologic changes. This is because the middle ear and mastoid gas-cell system is a relatively rigid (noncollapsible) gas pocket surrounded by a mucous membrane in which gases are exchanged between the middle-ear space and the mucosa. Differential pressure exceeds 54 mm Hg between the middle-ear space at atmospheric pressure and the microcirculation in the mucous membrane. This represents a diffusion-driven gradient from the middle-ear cavity to the mucosa that can produce an underpressure (relative to ambient pressure) in the middle ear of more than 600 mm H2O during equilibration. Doyle devised a mathematical model to explain certain estimates of gas exchanges constants for the middle ear.22 Also, Kanick, Doyle, Ghadiali, and Federspiel following morphometric models of pulmonary gas exchange and using a finite element model, concluded that morphometric techniques underestimate diffusion length by failing to account for the curvilinear gas flow pathways in the middle-ear gas exchange.23
FIGURE 4–6. Illustration of the sequence of events during ET dilation (active opening) owing to contraction of the tensor veli palatini muscle (TVP) during swallowing activity. A, ET at rest is closed. B, Proximal end of the cartilaginous lumen dilates first and is then followed by (C) dilation of the distal end and is open to the middle ear (ME). D, ET passively closes from the distal end to the proximal end to its resting, closed position.
FIGURE 4–7. Engineering model of a collapsible ET in which the global parameters (η and Etube) will depend on interfacial and tissue mechanical properties. Reproduced with permission from Ghadiali SN, et al.14
In the past, some investigators have ascribed the middle-ear mucosal gradient to differences in the partial pressures of oxygen; however, later research into the gas composition of the middle ear indicates that the middle ear and the microcirculation of the mucosa are similar with respect to oxygen and carbon dioxide.24 Ostfeld and Silberberg found that oxygen in the middle ear is slightly higher than in the microcirculation, in contrast to middle-ear carbon dioxide, which is in equilibrium.25 The diffusion gradient is created by differences in in the partial pressures of nitrogen and relatively inert gas with low solubility and permeability in an aqueous environment.26 The hydrops ex vacuo theory predicts that as the equilibrium condition is approached, transudation of liquid will occur, thereby decreasing the volume of the middle ear and consequently the pressure (see Chapter 6, “Pathogenesis”).
In early experiments in our laboratories, gas absorption was studied in the rhesus monkey using politzerization of air or oxygen gas, which showed that when the middle-ear gas composition is changed, middle-ear negative pressures rapidly occur.5 Serial tympanograms were obtained to determine the gas absorption process. During a 4-hour observation period, the middle-ear pressure was approximately normal in alert animals, whereas when the animals were anesthetized and swallowing was absent, the middle-ear pressure dropped to 60 mm H2O and remained at that level. The experiment indicated that, normally, middle-ear gases are nearly in equilibrium with the mucosal blood tissue gases or inner-ear gas pressures. Under these circumstances, the gas absorption rate is small because the partial pressure gradients are not great. In the normally functioning ET, the frequent openings of the tube readily equilibrate the pressure differences between the middle ear and the nasopharynx, with a small volume of air (1 to 5 µL) entering into the middle ear; however, an abnormally functioning tube may alter this mechanism.
TABLE 4–2. Gas Composition and Pressure in the Nasopharynx, Middle Ear, and Microcirculation of the Middle-Ear Mucosa Compared with Air (mm Hg)
Adapted from Felding JU, et al.24 and Ostfeld EJ and Silberberg A.25
In a later study in the monkey, the rate of constants for the middle-ear cavity to middle-ear mucosa exchange of oxygen and carbon dioxide is constant with a diffusion-limited process but not for N2, which indicated a much slower rate of N2 exchange than predicted. These studies showed that for relatively short time periods, middle-ear pressure is controlled by experimentally established oxygen and carbon dioxide gradients.20 In this animal model, the effects of varying oxygen and carbon dioxide tensions in the middle ear influenced the functions of ET pressure regulation of the middle ear, probably through receptors in the middle ear, implying a possible feedback loop.27 In the monkey, rates of exchange of gases across the tympanic membrane were assessed. Reports are that there is a measurable rate, but primarily for nitrogen, and then probably not physiologically under normal circumstances of breathing.28 Using a mathematical model, Doyle showed that mucosal surface area determines the middle-ear pressure response following sniff-induced middle-ear negative pressure, which did not support the previous hypothesis of a pressure regulation function of the mastoid gas cell mucosa.29
The ET provides a potential communication between the nasopharynx and the middle ear. When the tube opens owing to contraction of the tensor veli palatini muscle or other maneuvers, pressure differences between the ambient environment and the middle ear are equilibrated by the inflow and outflow of gases. Inflow of gas from the nasopharynx into the middle ear is end-expiratory gas; thus, the pressure regulatory function of the tube maintains near equilibrium between external and internal pressures, thereby maintaining nearly optimal transducer function of the middle ear and preventing the pathologic consequences that result from unabated middle ear to mucosa gas exchange.
Even though some investigators have postulated that gases can pass to and from the middle ear through the tympanic membrane, Doyle and colleagues conducted experiments that showed there is no oxygen and carbon dioxide transtympanic membrane exchange from the external ear canal into the middle ear.28 There is an exchange of N2, although not at physiologic rates.
ET Function in Adults Without Middle-Ear Disease
Normal ET function has been assessed by investigators in Malmö, Sweden. Using the microflow technique inside a pressure chamber, Elner and colleagues, in a classic study, evaluated 102 adults with intact tympanic membranes and no apparent history of otologic disorders (Table 4–3).30 Patients were divided into four groups according to their abilities to equilibrate static relative positive and negative pressures of 100 mm H2O in the middle ear. Patients in group 1 were able to completely equilibrate pressure differences across the tympanic membrane. Those in group 2 equilibrated positive pressure, but a small residual negative pressure remained in the middle ear. Subjects in group 3 equilibrated only relative positive pressure with a small residual remaining but no negative pressure. Those in group 4 were incapable of equilibrating any pressure. These data probably indicate decreased stiffness (increased compliance) of the ET in the subjects in groups 2, 3, and 4 compared with those in group 1. This study also showed that 95% of normal adults could equilibrate an applied positive pressure and that 93% could equilibrate applied negative pressure to some extent by active swallowing; however, 28% of the subjects could not completely equilibrate either applied positive or negative pressure or both. In an earlier study at the same laboratory, all 36 “healthy” ears could equilibrate over- and underpressures applied to the ears of adults.31
This important study showed that all adults with a negative history of ear disease and a healthy-appearing tympanic membrane can completely equilibrate applied pressure, especially when the pressure is negative. This finding helps explain why some individuals, despite a lack of recurrent or chronic middle-ear disease, have difficulty with equilibration of cabin pressures during descent in an airplane; the middle-ear pressure is negative while in air flight and must be actively equilibrated by swallowing (or other maneuvers, such as Valsalva’s maneuver or jaw movements) when descending. It should be kept in mind that the pressure chamber investigation described previously is not physiologic, but it does help in assessing the function of the ET. Similar to the unphysiologic nature of the testing, flying in an airplane or scuba diving is also not physiologic. We humans did not evolve with the capacity to equilibrate negative pressure developed during these endeavors. In addition, as every pilot and scuba diver knows, the more rapid the descent, the more difficult it is to equilibrate the middle-ear negative pressure, and if extreme, the tube can even “lock.”
TABLE 4–3. ET Function Test Results of 102 Otologically Normal Adults With Intact Tympanic Membranes
Adapted from Elner A, et al.30
Also, the study in adults has bearing on clinical trials that have been conducted in the past. In studies conducted in our department, apparently healthy adult volunteers were recruited for nasal challenge studies that involved virus and allergic antigens. Despite a lack of a history of middle-ear disease and normal tympanic membranes, some of the volunteers could not completely equilibrate applied pressures during the pre-challenge ET function testing in both ears. The subjects in these studies who could not perform all of the testing completely influenced the design and outcome of some of the early clinical trials, but this finding was taken into account for later ones.32–36
In an effort to determine normal ET function in adults, we conducted two studies in the past. In the first study, we tested six subjects (average age 29 years) who had a negative otologic history and previously normal tympanic membranes until they sustained a traumatic perforation of the tympanic membrane. All were tested using the inflation-deflation and forced-response test of function, which was considered normal function, and were compared with patients with otitis media (see Chapter 8, “Diagnosis and Tests of Function”).2
In another study, we recruited nine normal adult volunteers who had no history of middle-ear disease and normal tympanic membranes and who agreed to sign an informed consent form to allow me to perform a myringotomy, using topical anesthesia and a myringotomy knife under the otomicroscope. Of the nine subjects, one did not open the ET during sonometric testing and was excluded from further testing. The remaining eight subjects successfully opened the tube during swallowing when tested by sonometry, equilibrated positive and negative pressure during the inflation-deflation testing, and had normal values for the forced-response testing.37 Table 4–4 shows the normative values for the forced-response ET function testing obtained on these adult volunteers. In a recent study (2011) of tubal function in 20 adult volunteers without middle-ear disease in our laboratory, Swarts and colleagues38 tested ET function following myringotomy and reported that between-day data for the forced response test were highly correlated, but a percentage had constriction of the tube during swallowing, which is an abnormal finding. Bunne and colleagues also tested ET function in normal adult volunteers to establish normative values.39
TABLE 4–4. Results of Forced-Response ET Function Test of Eight Normal Subjects Who Had a Myringotomy
Forced-Response Parameter | mm H2O |
Po | 405 ± 50 |
Pc | 133 ± 77 |
RE = PE/QE | 11.9 ± 7 |
QA | 115 ± 70 |
RA = PA/QA | 2.3 ± 1.1 |
Adapted from S. J. Swarts, unpublished data.
Pc = closing pressure; Po = opening pressure; QA = equilibrium flow; RA = PA/QA = active resistance; RE = PE/QE = equilibrium resistance.
ET Function in Children Without Middle-Ear Disease
At the same laboratory in Malmö and using similar procedures to evaluate tubal function in adults, Bylander and colleagues demonstrated that children have less efficient ET function than adults (Table 4–5).40 The study consisted of 85 children and 92 adults who were considered otologically normal. Evaluation revealed that 34% of the children could not equilibrate applied negative intratympanic pressure by swallowing, whereas only 3% of the adult subjects were unable to perform this function. Children between 3 and 5 years of age had worse function than those aged 6 to 8 years. In this study and a subsequent one reported by the same research team, children who had middle-ear negative pressure evaluated by tympanometry had poor function.41
It is apparent from these studies that even in children, who were assumed to be otologically healthy, ET function is not as good as in adults. However, tubal function does improve with advancing age, consistent with the decreasing incidence of otitis media from infancy to adolescence.42 Because there are several differences in the anatomy of the ETs of a child and an adult, we also found functional differences in the ability to open the tube during swallowing activity to equilibrate pressure differences between the middle ear and the nasopharynx (see Chapter 3).
TABLE 4–5. ET Function Test Results in 85 Otologically Normal Children Compared with 92 Otologically Normal Adults with Intact Tympanic Membranes
Adapted from Bylander A et al.40
Another explanation for finding high negative middle-ear pressure in children is the possibility that some individuals who are habitual “sniffers” actually create underpressure within the middle ear by this act43; however, this mechanism is uncommon in children.
High middle-ear pressures have been identified, by otoscopy and tympanometry, in many children with no apparent middle-ear disease. An inefficient active opening of the tube in children probably explains this frequent finding.44 Brooks studied the parameters of middle-ear pressure using tympanometry and determined that the resting middle-ear pressure in a large group of apparently normal children was between 0 and −175 mm H2O.45 However, pressures outside this range have been reported as normal for large populations of apparently asymptomatic children.46 High negative middle-ear pressure does not necessarily indicate disease. It may indicate only physiologic obstruction of the ET. Pressure regulation occurs, but only after the nasopharynx–middle-ear pressure gradient reaches an opening pressure. It has been suggested that these children probably should be considered at risk of middle-ear problems until more is learned about the normal and abnormal physiology of the ET.47 In normal adults, Alberti and Kristensen obtained resting middle-ear pressures between −50 and +50 mm H2O.48 Again, a pressure outside this range does not necessarily mean that the patient has ear disease.
Because infants have an inefficient active opening mechanism, they most likely compensate in some way to regulate pressure within the middle ear. One possible compensatory mechanism is crying, and high positive pressure is apparent when some infants with no middle-ear effusion cry during otoscopy and tympanometry. This mechanism could also explain why infants cry when they are descending in an airplane; they are most likely insufflating air into their middle ears (Figure 4–8).
FIGURE 4–8. Because the ET is short and floppy in the infant, crying most likely insufflates nasopharyngeal gas into the middle ear to compensate for their inefficient tubal opening mechanism. But during periods of upper respiratory tract infection, nasopharyngeal secretions—and viruses and bacteria—may also be insufflated into the middle ear (see Chapter 5, “Pathophysiology”).
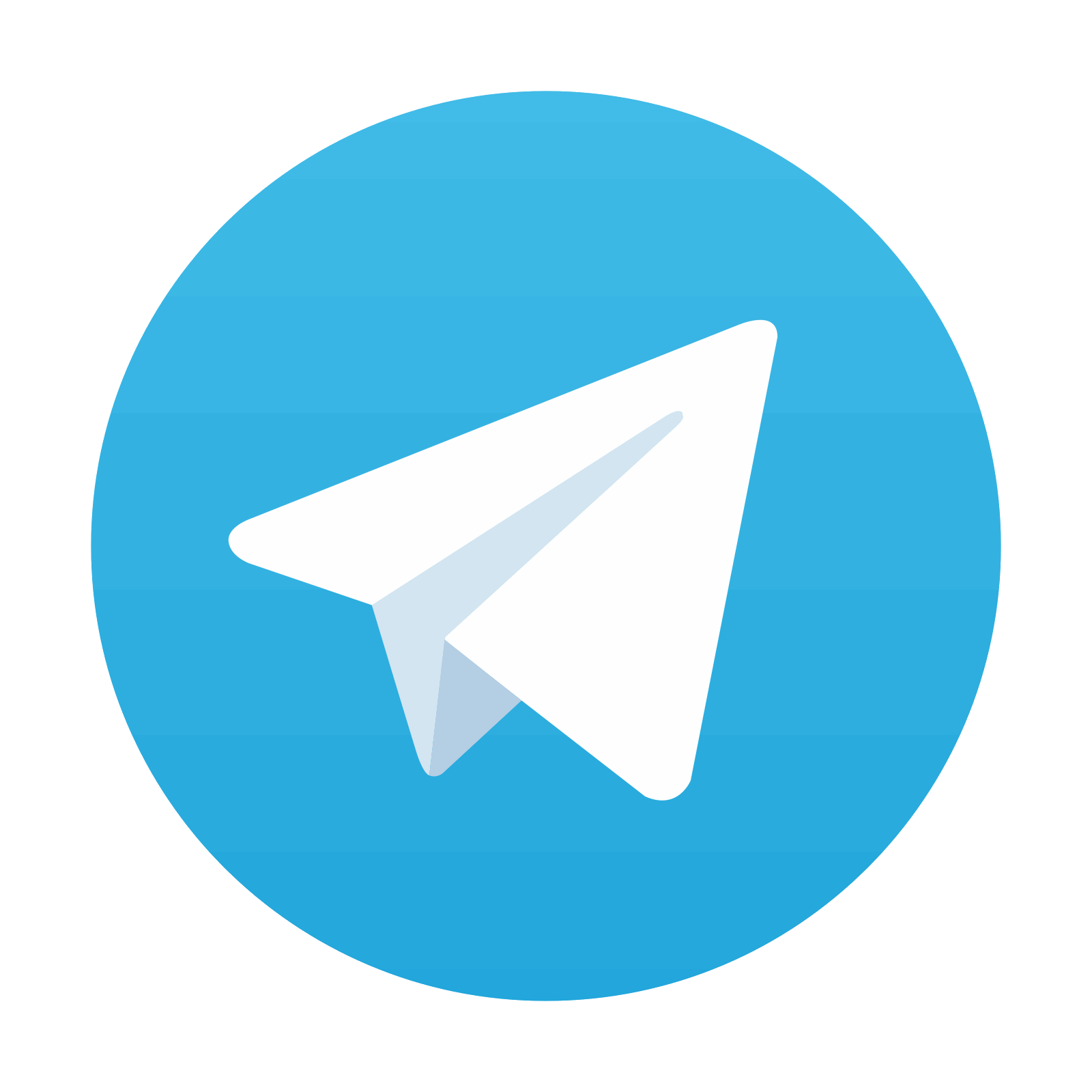
Stay updated, free articles. Join our Telegram channel

Full access? Get Clinical Tree
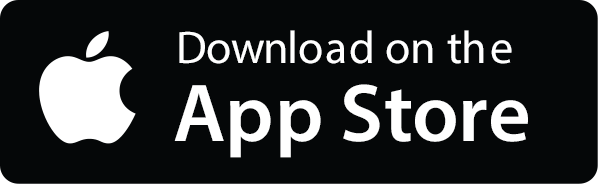
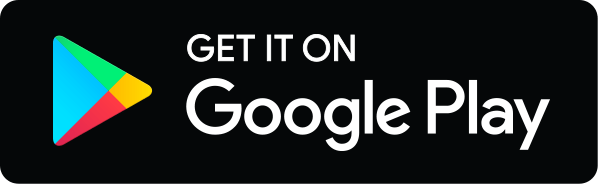