78 Adam G. Kaplan & Michael N. Ferrandino Division of Urologic Surgery, Duke University Medical Center, Durham, NC, USA Laparoscopic and robotic surgery challenge the patient with unique physiologic stresses. These stresses are primarily due to abdominal insufflation, but may be due to positioning requirements. Pneumoperitoneum, the result of abdominal insufflation, is considered necessary for visualization of the target organs, and may be maintained for prolonged periods of time. This can result in physiologic disturbances both during and after surgery as a result of increased abdominal pressure and absorption of the insufflated gas. The introduction of laparoscopic cholecystectomy in 1990 and laparoscopic nephrectomy in 1991 proved pivotal for the future of laparoscopy, as for the first time solid organs could be removed with laparoscopic instrumentation [1, 2]. Following these advances, two major changes occurred in the field of laparoscopic surgery: first, the complexity and – consequently – the duration of minimally invasive surgical cases increased dramatically. Second, the patients selected for laparoscopic and robotic surgery covered an increasingly broader spectrum of disease states, with many patients having underlying cardiac and pulmonary comorbidities. As a result, the minimally invasive surgeon today must give significant consideration to the physiologic impact of prolonged pneumoperitoneum. While most patients tolerate the physiologic changes associated with laparoscopy well, there are occasional life‐threatening complications as a result of the physiologic reaction to the laparoscopic environment. Pneumoperitoneum and patient positioning required for laparoscopy have several well‐understood physiologic effects which will be reviewed extensively in this chapter, along with the complications of pneumoperitoneum and consideration of methods of altering pneumoperitoneum that mitigate certain unwanted side effects. A number of patient positions are used during laparoscopy to more easily target the organ of interest. In urology, the lateral position is commonly used for upper urinary tract and retroperitoneal procedures; this position has little effect on hemodynamics unless there is extreme flexion or improper use of the kidney rest such that the vena cava is compressed, wherein venous return to the heart can be reduced [3]. The lateral position can lead to ventilation–perfusion mismatch and changes to pulmonary compliance for the dependent lung, as well as over‐inflation and potential barotrauma to the nondependent lung. During upper abdominal surgery, which is uncommon for urologic procedures, the patient can be placed head up (reverse Trendelenburg) to drop the bowel away. In most cases, this position demonstrates improved pulmonary mechanics [4–6]. However, this position also decreases cardiac output [7, 8] and can lead to profound hypotension and cerebral hypoperfusion, particularly in patients with pre‐existing dysfunction of cerebral autoregulatory mechanisms, such as those with chronic hypertension, history of cerebrovascular accident, or carotid occlusive disease. A position more commonly used by the urologist is Trendelenburg (head down), which can facilitate pelvic, lower urinary tract surgery such as radical prostatectomy. This position increases cardiac output modestly [9–12]. Additionally, it restricts diaphragmatic movement and increases ventilation–perfusion mismatch [5, 13, 14]. As one might expect, the effects on cardiovascular and respiratory physiology are stronger with steeper head‐down angles [15]. Table 78.1 compares the cardiovascular effects of the head‐up and head‐down positions. Table 78.1 Effects of patient position on hemodynamic parameters compared to the supine position. Today, the vast majority of laparoscopic and robotic cases are performed with carbon dioxide (CO2) pneumoperitoneum. During the development of laparoscopy, the early cases were performed using oxygen and room air for insufflation [16]. These proved to be dangerous and quickly abandoned due to the significant potential for venous air embolism, intra‐abdominal explosion, and combustion. Carbon dioxide was selected as it met a number of important criteria for the ideal gas for insufflation: it is readily available, inexpensive, non‐combustible, rapidly soluble in plasma, and removed via respiration [17]. Due to the favorable properties of CO2, it was adopted quickly. The rapid absorption of CO2 decreased the chance of postoperative abdominal distention, and lowered the probability of developing a gas embolism. These same properties have dangers as well: they can lead to hypercapnia, hypercarbia, and cardiac dysrhythmia. While these sequelae are typically self‐limiting, they can be problematic for patients with poor cardiopulmonary function and those with chronic obstructive pulmonary disease, as the minute ventilation may not be able to be increased sufficiently to compensate for the increased CO2 absorption. Other insufflation agents such as nitrogen dioxide (N2O) and helium are possible alternatives in the select patient. While N2O came into favor in the 1970s and 1980s due to low cost, decreased peritoneal irritation, and fewer cardiovascular adverse effects compared to CO2, case reports of intraoperative explosions due to its combustibility severely limited its use in the therapeutic setting [18–21]. In fact, it should be limited to cases where electrosurgical equipment is not needed. Helium, in contrast, is both inert and noncombustible. Rademaker et al. demonstrated favorable effects of helium on atrial partial pressure without hypercarbia or acidosis in a porcine model [22]. Helium insufflation may be useful in select patients with pulmonary disease who would not tolerate the hypercarbia associated with CO2 insufflation [23]. The main drawback of helium is its low blood solubility, which may portend to a higher risk of gas embolism [24]. Considering the widespread, nearly exclusive use of CO2 insufflation for laparoscopic procedures, the remainder of the chapter will focus on pneumoperitoneum with CO2. Pulmonary physiology is affected by a number of aspects during laparoscopic surgery. Patient size and the existence of underlying pulmonary pathophysiology certainly affect how the patient will respond to insufflation. The mechanical effects of pneumoperitoneum and the absorption of CO2, however, are the main drivers of the physiologic changes seen during laparoscopy (Table 78.2). Table 78.2 Respiratory effects of laparoscopy with intra‐abdominal pressure of 10–20 mmHg. As pneumoperitoneum increases the intra‐abdominal pressure (IAP), it exerts a mechanical limitation to excursion of the diaphragm. This limitation results in decreased lung capacity and compliance, with eventual ventilation–perfusion mismatch and shunting [25, 26]. Consequently, there is an overall decrease in vital capacity, functional residual capacity, total lung volume, and pulmonary compliance, alongside increases in peak inspiratory pressure [4, 5, 27]. These changes are exacerbated by obesity or other causes of pre‐existing atelectasis and decreased lung volumes [4, 28]. Carbon dioxide has many properties, including its rapid solubility and quick absorption into the circulation, making it the ideal gas for insufflation. As such, it must be eliminated efficiently by the lungs. While normal CO2 production in the average adult ranges from 150 to 200 ml/min [29, 30], the rate of CO2 absorption during laparoscopy is 14–48 ml/min [31–33]. Adjustments must be made in minute ventilation by either increasing tidal volume or respiratory rate to mitigate the effects of increased blood levels of CO2. If not corrected for, hypercarbia can lead to respiratory acidosis, which can depress cardiac function and cause arrhythmia. Subcutaneous emphysema, increased IAP, extraperitoneal insufflation, and prolonged surgery can also increase the rate of CO2 absorption [34]. The minimally invasive surgeon must be aware of the compounding effects of CO2 absorption over time, and how they can linger in the postoperative period. In a prospective study of changes in end‐tidal CO2 for healthy patients undergoing laparoscopic cholecystectomy, Meftahuzzaman et al. showed that significant alteration occurs 40 minutes after insufflation during cholecystectomy [35]. Many urologic cases can extend well past this period of time, and close monitoring of end‐tidal CO2 is imperative. Additionally, some degree of CO2 is also sequestered in the skeletal system, which is the largest reservoir in the body, and it is eliminated slowly in the postoperative period. This can be particularly problematic for patients with pre‐existing cardiopulmonary dysfunction, and these patients must be monitored closely [36]. Several hemodynamic effects are seen as a result of increased IAP, including decreased cardiac output, increased mean arterial pressures (MAP), increased systemic vascular resistance (SVR), and increased pulmonary vascular resistance (Table 78.3). At low insufflation pressures (<10 mmHg) there is an initial increase in venous return; this “auto‐transfusion” causes the cardiac output and cardiac index to increase. Conversely, above 20 mmHg, venous return is hindered and there is a considerable decrease in cardiac output [37]. Table 78.3 Hemodynamic effects of laparoscopy with intra‐abdominal pressure of 10–20 mmHg. Patient factors can attenuate the cardiac response to pneumoperitoneum. Volume status is important as hypovolemic patients tend to have a more exaggerated drop in cardiac output in response to increased IAP. This was demonstrated by Ho et al. using a porcine model of acute hemorrhage [38]. Therefore generous volume loading can be recommended for patients undergoing laparoscopic procedures. Additionally, there is evidence that vagal stimulation from peritoneal distention can also decrease cardiac output. In fact, pneumoperitoneum causes excessive stretching of the vagal nerve endings, which are richly distributed throughout the abdominal cavity, which can lead to bradycardia [39]. Patient positioning, particularly for deep pelvic surgery, also has a significant impact. The Trendelenburg position decreases venous return. This exacerbates the effect of increased IAP, which further decreases venous return due to caval compression [40] and increased venous resistance [41, 42]. Of note, the opposite effect occurs with reverse Trendelenburg. Increased SVR and MAP are also attributable to the sympathoadrenal effects of increased IAP and CO2 insufflation [43]. There is increased catecholamine production, presumably from both surgical stress and directly from the increased IAP. Early hypercarbia may also exacerbate this sympathomimetic effect; however, as hypercarbia progresses and acidosis becomes more profound, a cardiodepresent effect predominates. The renin‐angiotensin system and vasopression are also postulated to be contributors to the increases in SVR [44–48]. There is a rapid increase in plasma renin activity during induction of pneumoperitoneum, and increased plasma renin activity positively correlates with MAP [49–51]. Additionally there is a significant increase in anti‐diuretic hormone (ADH), which controls arterial blood pressure via receptors in vascular smooth muscle; this can provoke intense vasoconstriction and likely plays a key role in regulating hemodynamics during laparoscopy [46, 48]. A recent study by Rosendal et al. [52] sought to determine changes in preload, afterload, and cardiac function in the different phases of robotic prostatectomy, including induction of anesthesia, initiation of pneumoperitoneum, immediately after a 45° head‐down tilt, 15 minutes later into the head‐down tilt, after release of pneumoperitoneum, and 5 minutes after returning to a horizontal position. Central venous pressure increased threefold from baseline after induction of anesthesia, but no other preload parameters showed overload or demand. There was no change in cardiac contractility over time. Afterload increased significantly during CO2 pneumoperitoneum and decreased compared with baseline after the release of pneumoperitoneum at the end of the case. Heart rate and cardiac index increased significantly during robot‐assisted laparoscopic prostatectomy [52]. Oliguria is a well‐characterized side effect of sustained pneumoperitoneum. This is important to know, as relying on urine output alone as an indicator of fluid status can lead to fluid overload in overzealous hands. There are several possible causes of oliguria in this setting, which appear to occur at an IAP around 15 mmHg [53]. It is well documented that elevated IAP causes significant renal hypoxia and decreased renal blood flow [54]. One theory as to why this occurs is that there is a Page kidney effect, whereby applying renal parenchymal compression causes a decrease in urine output, glomerular filtration rate (GFR), and effective renal blood flow. Razvi et al. proved this experimentally by placing a pressure cuff at 15 mmHg on the left kidney of canines, thereby causing overall urine output to decrease [55]. Using a porcine model, Chiu et al. demonstrated that pneumoperitoneum‐induced oliguria may also be due to increased serum aldosterone levels [56]. Elevated IAPs are thought to stimulate endogenous catecholamine release, another potential contributor to oliguria. Circulating levels of endothelin, a catecholamine, is increased in response to pneumoperitoneum [57]. Studies of unilateral renal vein compression in a rat model and a canine model showed decrease in GFR and urine osmotic pressure in both kidneys. In each case, circulating endothelin increased [53, 58]. Vasopressin has also been implicated as a contributor to oliguria. Krisch et al. demonstrated in a rat model that using vasopressin antagonists during periods of increased IAP improved renal function compared to control [59]. Other possible causes of oliguria have been studied and disproven. The decreased cardiac output seen with increased IAP is unlikely to affect renal output; studies have shown that normalization of the cardiac output with fluid resuscitation does not lead to increased urine output [60, 61]. Ureteral obstruction, similarly, is unlikely to contribute significantly. Patients with ureteral stents, which presumably remain unobstructed, do not have increased urine output [62]. Additionally, animal imaging studies with intra‐operative urography show no evidence of ureteral obstruction during pneumoperitoneum [56]. Importantly, the renal effects from increased IAPs tend to subside in the immediate postoperative period in healthy patients with normal renal function [63]; however, those with pre‐existing renal dysfunction may experience longer delays to return to baseline GFR [64]. A rabbit model of severe hydronephrosis showed that obstructed kidneys were more likely to suffer acute kidney injury when exposed to pneumoperitoneum [65]. While pneumoperitoneum was certainly feasible in rats with impaired baseline renal function, it appeared worse for those with acute kidney injury than chronic renal insufficiency [66]. Patients with significant cardiovascular dysfunction such as decompensated congestive heart failure also appear to be more susceptible to the adverse renal effects of pneumoperitoneum [67]. Changes in cerebral blood flow during laparoscopy can occur even at a mildly increased IAP, and are due to several factors: cardiovascular changes, alterations in PaCO2, patient positioning, and the increased intra‐abdominal and intra‐thoracic pressures. Rosenthal et al. demonstrated increases in intracranial pressures (ICPs) and IAPs above 8 mmHg in a large‐animal model [68]. This is thought to be due to compression of the vena cava, which increases central venous pressures and decreases drainage from the central nervous system. Studies have also shown that cerebral blood flow velocity increases due to the increase in PaCO2 that results from CO2 pneumoperitoneum [69]
Physiologic Considerations in Laparoscopic and Robotic Surgery
Introduction
Impact of patient positioning
Hemodynamic parameter
Head up
Head down
Heart rate
↑
↓
Mean arterial pressure
↓
↑
Systemic vascular resistance
↑
↓
Cardiac output
↓
↑
Intracranial pressure
↓
↑
The gaseous medium: carbon dioxide and other insufflation agents
Physiologic organ changes caused by insufflation with carbon dioxide
Effect on the lungs
Respiratory parameter
Change
Chest wall mechanical resistance
↑
Peak inspiratory pressure
↑
Pulmonary compliance
↓
Alveolar dead space
↔ or ↑
Functional reserve capacity
↓
Forced vital capacity
↓
FEV1
↓
Peak expiratory flow
↓
Effect on the heart and hemodynamics
Hemodynamic parameter
Change
Central venous pressure
↑
Systemic vascular resistance
↑
Heart rate
↑
Mean arterial pressure
↑
Cardiac output
↓
Effect on the kidneys
Effect on the brain
Stay updated, free articles. Join our Telegram channel

Full access? Get Clinical Tree
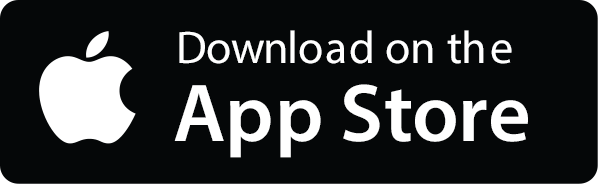
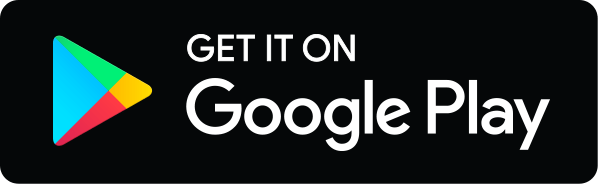