div class=”ChapterContextInformation”>
15. Upcoming Pharmacological and Interventional Therapies for the Treatment of Physical Frailty and Sarcopenia
Keywords
TestosteroneHypogonadismMyostatinGrowth hormoneAmmoniaRifaximinPortal hypertensionTIPSIntroduction
In recent years there has been a surge in research investigating mechanisms underlying muscle wasting in cirrhosis. The central role of hormonal alterations, ammonia, and myostatin in muscle wasting is now well established. However, despite these gains, few interventional trials have been conducted, and there are as yet no proven therapies that lead to a sustained improvement in muscle mass and strength. All treatments are therefore still considered investigational and require further validation in large-scale randomized controlled trials.
Interventional trials for sarcopenia and frailty have several inherent challenges. These include a lack of consensus on diagnosis, optimal definition of primary and secondary endpoints, and the subjectivity of performance and strength measures. Furthermore, whether reversing sarcopenia in cirrhosis has a meaningful impact on patient outcomes is still yet to be determined. Given the multifactorial nature of sarcopenia in cirrhosis, it is also likely that a unique pattern of alterations in skeletal muscle biology are present in an individual patient with cirrhosis, meaning that specific single pathway interventions may impact each patient to varying degrees.
Future interventional therapies for sarcopenia in cirrhosis
Physiological abnormality | Mechanism of muscle loss | Therapeutic target |
---|---|---|
Testosterone deficiency | Testosterone deficiency common in cirrhosis due to downregulation of the HPA due to systemic inflammation and chronic disease Reduced activation of androgen receptors in muscle cell nuclei and satellite cells | Testosterone replacementa,b Selective androgen receptor modulatora |
Portal hypertension | Malnutrition: Early satiety from ascites, intestinal dysmotility, malabsorption, increased protein losses from paracentesis Hypermetabolism: Promotion of a systemic inflammatory response, increased rates of bacterial translocation and endotoxemia | TIPSc Continuous terlipressin infusionc |
Hyperammonemia | Cataplerosis: Detoxification of ammonia in skeletal muscle depletes TCA cycle of key intermediate resulting in mitochondrial dysfunction and reduced protein synthesis Creation of reactive oxygen species leading to increased autophagy of muscle cells Cellular stress response as seen in amino acid starvation | Rifaximind LOLAd BCAA therapyb |
Growth hormone resistance | Reduced IGF-1 and IGFBP-3: Decreased production due to hepatocyte dysfunction Reduction in GH receptors in hepatocytes IGF-1 inhibits myostatin IGF-1 involved in muscle stem cell proliferation, muscle cell repair, and protein synthesis | Growth hormone replacementa IGF-1b |
Upregulation of myostatin | Elevated ammonia, low IGF-1, follistatin, testosterone upregulates myostatin Myostatin inhibits mTORC1 signaling leading to increased muscle cell autophagy, reduced protein synthesis, and proteasome-mediated proteolysis | Inhibitors of myostatin signaling pathwaya |
Malnutrition | Anorexia: Early satiety, systemic inflammatory response, chronic disease | Ghrelin agonista |
Testosterone Therapy
Testosterone Replacement
Low testosterone is frequently observed in cirrhotic men and has been shown to correlate with severity of sarcopenia in this cohort [1, 2]. The cause of low testosterone is multifactorial and poorly understood but likely relates to downregulation of the hypothalamic-pituitary-gonadal axis due to systemic inflammation and chronic disease [3]. In addition to low testosterone, sex hormone-binding globulin is elevated in cirrhosis, leading to a further reduction in free testosterone, with low free testosterone observed in up to 90% of cirrhotics [4].
Signs of low testosterone are common in cirrhosis, including impaired sexual function, low libido, fatigue, osteoporosis, gynecomastia, anemia, and of course muscle wasting. However, the specific contribution of low testosterone to these factors is unclear. Testosterone is a known anabolic hormone, and therefore it is reasonable to expect that returning the level of this hormone to normal physiologic levels should improve muscle mass. However only a small number of trials have investigated the use of testosterone for this purpose with varying methodologies.
In 1960, a study was published in the Lancet that randomized 97 male patients with cirrhosis to either prednisolone 20 mg per day, intramuscular testosterone, or standard therapy on admission to hospital for liver-related disease [5]. Cirrhosis was confirmed by biopsy, but patients were not required to be hypogonadal for study entry. Treatment duration and follow-up were not standardized, and patient attendance was sporadic, making it difficult to compare groups. Patients in both the testosterone and prednisolone arm did appear to have improved morbidity and mortality. Mortality was significantly higher at 55% in the placebo arm compared to 31% in the testosterone arm. However, given the lack of a clear protocol and lack of blinding, these results therefore should be interpreted with caution.
A smaller study was published by Puliyel et al. in 1977 [6] that showed similar but less dramatic results. This study recruited 21 patients with biopsy-proven cirrhosis; however one third of these patients were women. Twelve patients were placed on active therapy with intramuscular testosterone, and nine controls received standard of care. Over only a short period of 4 weeks, albumin and energy levels improved, and edema decreased in the active arm. There was no mortality benefit. Again, the trial was not blinded, patients were not required to be hypogonadal to enter this study, and there was no controlling for baseline confounders. In addition, the very small patient numbers and inclusion of females into a study using male hormones mean that strong conclusions cannot be drawn.
A Cochrane review in 2006 identified trials that examined the use of testosterone in men with alcoholic liver disease [7]. Only five randomized controlled trials were identified that met criteria, which included a total of 499 patients. Overall there was no significant impact on liver histology or mortality and no increase in adverse effects in men on testosterone. The sole positive finding was a significant reduction in gynecomastia. The interpretation of this review is limited by the fact that only two of the five studies recruited men with cirrhosis, with three studies examining acute alcoholic hepatitis, limiting the applicability to chronic liver disease. Furthermore, all studies examined men with alcohol-related liver disease who received counseling regarding alcohol intake during the study period, and thus many ceased alcohol during this time. Alcohol cessation can itself improve survival as well as testosterone levels, potentially confounding results. None of the trials required that men have low testosterone for study entry, which may reduce the expected beneficial effect of testosterone.
Of these two trials included in the Cochrane review, one constitutes the largest published study to date on testosterone therapy in men with cirrhosis [8]. This multicenter trial included 221 men with alcohol-related, biopsy-proven cirrhosis, with 134 randomized to active treatment with oral testosterone 3 times a day and 87 to placebo. The median duration of treatment was 30 months. The findings of this study included increased testosterone and estradiol levels on treatment and an increased testosterone-to-estradiol ratio. There was a significant reduction in gynecomastia and increase in hemoglobin. No difference in liver biochemistry or histology was seen, and there was no mortality benefit. Limitations of this study include failure to randomize by severity of liver disease and use of oral testosterone, which is now considered unsafe, particularly in the context of liver disease. In addition, patients were not required to have low testosterone levels for trial entry, and almost all patients in the placebo group had normal testosterone levels. The median on-trial testosterone level was well above normal with 25% of patients achieving testosterone levels >100 nmol/L, which increases the risk of adverse effects.
The other trial in the Cochrane review included 32 men with alcohol-related cirrhosis who were randomized into 1 of 3 different arms [9]. Patients were not required to have low testosterone levels for trial entry. Arm 1 included nine men on intramuscular testosterone. Arm 2 included 12 men on the synthetic anabolic steroid methenolone. Arm 3 was a placebo arm of 11 patients. Treatment duration was only 1 month. There was no significant difference in mortality, ascites, encephalopathy, hepatocellular carcinoma (HCC) incidence, liver biochemistry, or liver histology. The small numbers meant the trial was underpowered to show any significant differences between patient groups. In addition, the short duration of 1 month may not have been adequate to show any difference if it was present.
In 2011, Yurci et al. published a very small study including only 12 men with cirrhosis of any etiology [10]. This was the first trial to require men to have low testosterone levels for study entry. Testosterone gel was administered to all 12 men for 6 months. At trial completion, muscle strength by hydraulic hand dynamometer had significantly improved, and gynecomastia had reduced. There were no significant adverse events. There was no change in bone mineral density. Unfortunately, there was no control arm, so these results must be interpreted with caution, particularly in the context of such low patient numbers, as this increase in muscle strength may have been due to other factors.
Our group recently published a 12-month randomized placebo-controlled trial of 3-monthly testosterone undecanoate in 101 men with established cirrhosis and low baseline testosterone levels [1]. This was the first study with both, a requirement for cirrhosis and low testosterone at baseline and randomization of patients to appropriate testosterone replacement therapy as compared to placebo. In addition, it remains the only trial to specifically quantify changes in muscle mass on testosterone therapy in this cohort. This study showed a significant improvement in lean mass as measured by dual-energy X-ray absorptiometry (mean adjusted difference) (MAD) in appendicular lean mass of +1.69 kg (CI +0.40; +2.97 kg, p = 0.021) and MAD in total lean mass of +4.74 kg (CI +1.75; +7.74 kg, p = 0.008). Additional positive benefits observed in this study included a significant increase in bone mass in treated subjects (MAD +0.08 kg, CI +0.01; +0.15 kg, p = 0.009) and a reduction in fat mass (MAD −4.34 kg, CI −6.65; −2.04, p < 0.001). This remains the only pharmacological therapy for sarcopenia that is supported by randomized controlled data.
Selective Androgen Receptor Modulator
The androgen receptor is expressed in many tissues, and therefore the nonselective effects of testosterone therapy limit its widespread use. Of particular concern is the association with increased cardiovascular risk, clot formation, fluid retention, benign prostatic hypertrophy, and its virilization effects, particularly in women. Selective androgen receptor modulators (SARMs) are a novel class of androgenic drug which provide tissue-specific activation of the androgen receptor. They are an attractive alternative anabolic agent as they are orally active without the systemic effects of testosterone therapy. SARMs have been investigated as a potential therapy for osteoporosis, stress urinary incontinence, and breast cancer with a favorable side effect profile [11].
There is also emerging evidence for their use in muscle wasting disorders. In animal models of glucocorticoid-induced muscle wasting, SARMs were effective at blocking the atrophic effects of dexamethasone on muscle cells [12]. In a phase II clinical trial of cancer-related cachexia, a SARM, enobosarm, improved muscle mass compared to placebo [13]. However, in unpublished data from two phase III clinical trials, results have been inconsistent. In the POWER 1 and 2 clinical trials investigating enobosarm in lung cancer patients, the co-primary endpoints were an improvement in muscle mass and stair climb power. While lean muscle mass improved compared to placebo in the POWER 1 trial, stair climb power did not. In POWER 2, neither endpoint met significance compared to placebo [14]. A phase IIA randomized placebo-controlled trial studied the effects of the SARM, MK-0773, in 170 sarcopenic elderly females [15]. MK-0773 improved lean muscle mass after 6 months of therapy; however, there was no significant increase in performance measures compared to placebo. More research is required to establish the anabolic effects of SARMs. Furthermore, their safety and efficacy are untested in a cirrhotic population.
Reduction in Portal Hypertension
Portal hypertension contributes significantly to malnutrition in cirrhosis. Malnutrition is a major driving factor for muscle depletion and sarcopenia. Ascites, a common complication of portal hypertension, leads to reduced gastric reserve and early satiety. It has also been shown to increase the resting energy expenditure in cirrhosis [16]. Large volume paracentesis for control of ascites is associated with protein losses and may further exacerbate protein malnutrition [17]. Portal hypertension is also thought to contribute to malabsorption through gastric and intestinal dysmotility [18]. Elevated portal pressures also result in gut edema, endotoxemia, and increased rates of bacterial translocation. These factors lead to a systemic inflammatory response and hypermetabolism and are associated with anabolic resistance. Endotoxemia in cirrhosis has been shown to have direct effects on protein breakdown and reduced synthesis in rat models [19]. Therefore, therapies to reduce portal pressures mechanistically might increase muscle mass through improved nutrition and reduced endotoxemia, inflammation, and hypermetabolism.
Continuous Terlipressin Infusion
Terlipressin, a synthetic vasopressin analogue, is a potent splanchnic vasoconstrictor. It is used to decrease portal pressures in acute variceal hemorrhage and in the treatment of hepatorenal syndrome (HRS). Splanchnic vasoconstriction promotes increased relative circulating blood volume and improves renal perfusion. Terlipressin as a treatment for HRS has demonstrated superiority over octreotide and midodrine and similar efficacy to noradrenaline [20, 21]. However, it has failed to gain Food and Drug Administration (FDA) approval in the USA primarily due to issues with trial design. The short half-life of terlipressin has also traditionally limited its use beyond the acute inpatient setting.
Our center has recently published data on the novel use of continuous outpatient terlipressin infusions for the treatment of refractory ascites and HRS as a bridge to transplantation [22–24]. This work identified significant secondary benefits on nutrition and muscle function [25]. After receiving a continuous outpatient terlipressin infusion for HRS or refractory ascites for a median duration of 51 days, 19 patients who had previously been shown to have progressively deteriorating muscle function had a median increase in handgrip strength of 11% [25]. Energy and protein intake also improved significantly across the cohort. There was a significant reduction in large volume paracentesis during therapy without any safety issues identified. Whether improvements in handgrip strength were solely attributable to improved protein and calorie intake remains unclear; however, it is possible that nutrient absorption and bacterial translocation were also improved. Larger clinical trials are clearly required to validate these positive findings.
Transjugular Intrahepatic Portosystemic Shunt (TIPS)
Transjugular intrahepatic portosystemic shunt (TIPS) is a radiological procedure used to decrease portal pressures. TIPS procedures are performed most commonly for secondary prevention or salvage therapy following variceal hemorrhage and for the management of refractory ascites. There is emerging evidence that TIPS may also improve muscle mass. Plauth et al. prospectively examined changes in body composition in 21 patients who underwent TIPS procedure [26]. Over 12 months of post-procedure follow-up, there was a significant increase in body cell mass measured by bioelectrical impedance. Tsien et al. assessed the effect of TIPS on quantitative measures of muscle and fat mass on serial CT scans at the mid-L4 vertebrae [27]. In patients undergoing TIPS, muscle area improved (psoas muscle area; 22.9 ± 0.9 to 25.1 ± 0.9 cm2, paraspinal muscle area; 54.5 ± 1.3 to 57.9 ± 1.5 cm2), and visceral fat mass decreased. Seventy-two percent of patients had an increase in muscle mass. Interestingly, patients who demonstrated an improvement in lean muscle mass had improved overall survival compared to patients who had no change in muscle area. While this study was retrospective, this is the only study to our knowledge that suggests that successful treatment of sarcopenia in cirrhotic subjects may lead to improved overall survival.
The mechanisms underlying the changes in body composition following TIPS placement may be similar to terlipressin therapy via the reduction in portal hypertension and subsequent control of ascites. This may improve gut function and nutrient absorption and reduce the hypermetabolic state associated with ascites and endotoxemia. Improved caloric intake following TIPS has been examined with inconsistent results. Plauth et al. reported an increase in mean protein and caloric intake in 21 patients who underwent TIPS [26]; however, two other prospective studies showed no significant change [28, 29]. Hormonal changes have also been explored. These include plasma leptin, IGF-1, IGF-binding protein, growth hormone, glucagon, insulin, and cortisol levels, all of which showed no significant change following TIPS in small prospective studies [30–32]. Nevertheless, in contrast to terlipressin, by creating a direct portosystemic shunt, the TIPS procedure bypasses hepatic metabolism leading to an increase in circulating levels of ammonia. The increase in incidence of hepatic encephalopathy post-TIPS is well documented, yet the changes in muscle mass following TIPS are incongruous with the cellular effects of hyperammonemia. Clearly sarcopenia in cirrhosis is a complex interplay between multiple factors, and the relative impact of portal hypertension as compared to hyperammonemia remains unclear.
Ammonia-Lowering Therapies

Metabolism of ammonia through the TCA cycle. Ammonia (NH4+) detoxification in non-hepatic tissue occurs through the formation of glutamine and glutamate from α-ketoglutarate (α-KG), a key intermediary for the tricarboxylic acid (TCA) cycle. The TCA is critical series of chemical reactions that occurs as part of aerobic respiration to produce ATP for energy. The conversion of glutamate to glutamine is catalyzed by glutamine synthetase which is found in high concentrations in muscle. States of hyperammonemia deplete the TCA cycle of α-KG (cataplerosis), resulting in mitochondrial dysfunction and reduced ATP production, ultimately contributing to muscle loss. Metabolism of branched-chain amino acids (BCAAs) assists in replenishing the TCA cycle of its intermediates through production of acetyl-CoA
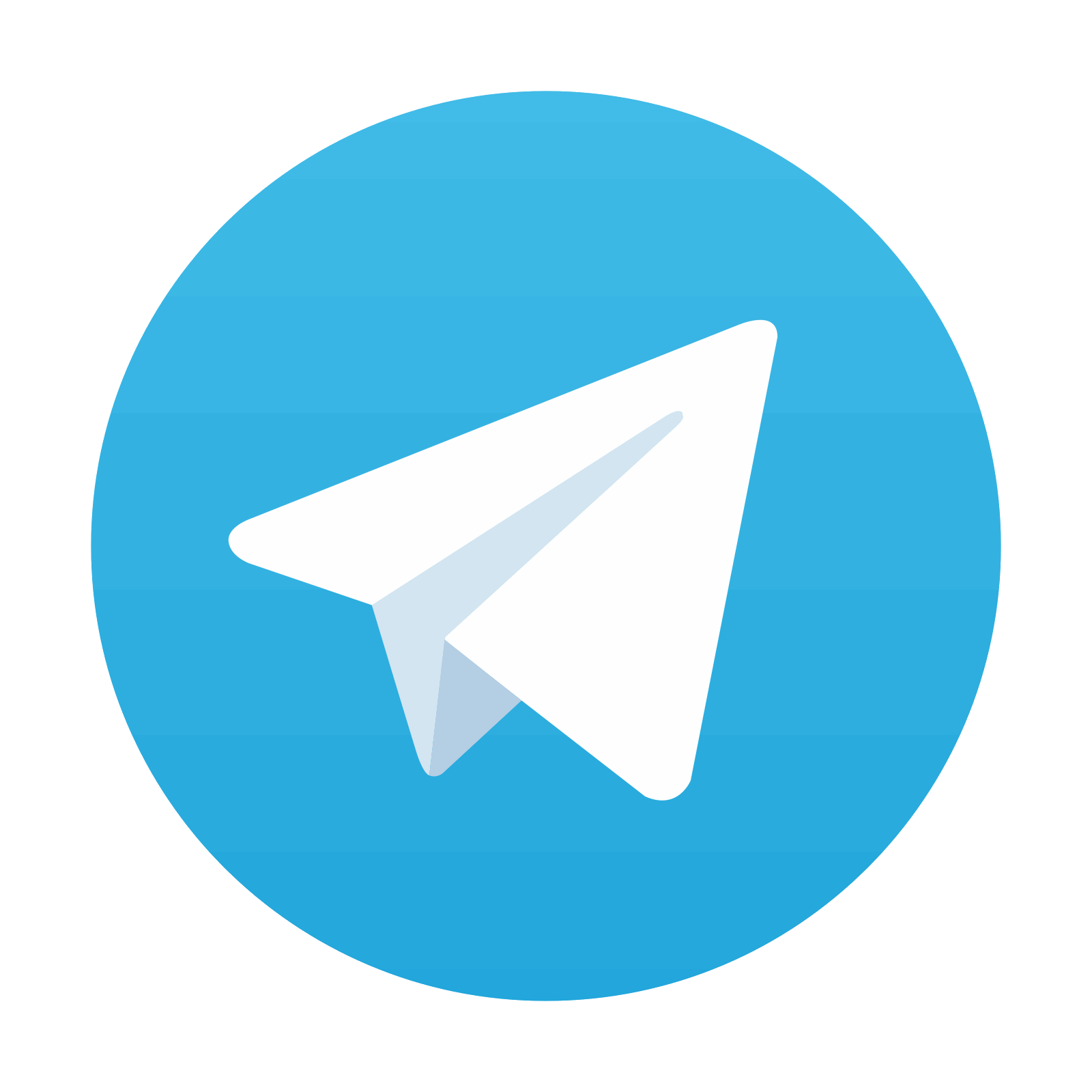
Stay updated, free articles. Join our Telegram channel

Full access? Get Clinical Tree
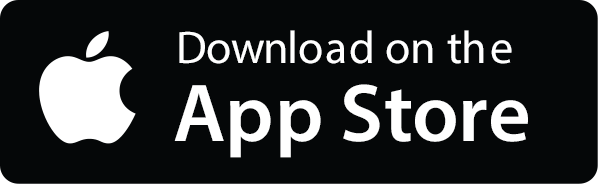
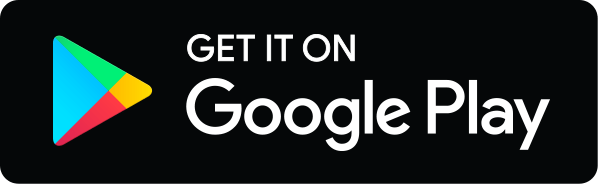