The viewpoints of enthusiasts and skeptics in relation to the role of probiotics should not be allowed to distract clinicians from the bigger issue, which is the pivotal role of the microbiota in the protection against many disorders and in the pathogenesis of others. However, all probiotics, like all bacteria, are not created equal, and therapeutic deployment in a generic sense is as absurd as the administration of pills or tablets without regard for the nature of the active ingredient and the intended effect. The rationale for therapeutic manipulation or supplementation of the microbiota is sound in conditions where the intestinal ecosystem is poorly developed, such as in low birth weight neonates, or where it is profoundly disturbed, such as after broad-spectrum antibiotics. In other conditions, such as irritable bowel disorder (IBD), the efficacy of some, but not all, probiotics has been a welcome surprise. However, the impact of probiotics is likely to be modest and is probably more complicated in IBD. In choosing a probiotic strategy, clinicians should adhere to the principles of evidence-based therapeutics. These include: selection from a reputable supplier, with appropriate documentation of contents and shelf life; anticipation of strain-specific effects; avoidance of cocktails without documentation of the activities of each ingredient with absence of interstrain antagonism; and published evidence of efficacy from clinical trials.
An inescapable truism is that the human genome does not encode sufficient information for full development. For optimal maturation, particularly of mucosal tissues and sensory organs, including the immunosensory system, stimulation from the environment is required. In the gut, this is derived from the microbiome. The microbiota colonizing the neonate exerts trophic, metabolic, and protective effects on developing intestinal and extraintestinal organs and is critical for homeostasis throughout life. This inner biomass is an asset for health, protective against disease, and yet, depending on the context, some of its components may become a risk factor for disease. Therein is the rationale for exploring the potential benefit of managing or manipulating the human microbiota.
The most striking example in the developed world of successfully targeting a component of gut microbiota in the treatment of disease has been the story of Helicobacter pylori with peptic ulcer disease and gastric cancer. The real lesson of that story was that some chronic human diseases can never be solved by research and therapeutics focused exclusively on the host, without due regard for interactions with environmental microbiota. Despite this, most current therapeutic strategies for many disorders including inflammatory bowel disease (IBD) still focus on modifying the host response, while the microbiota, an essential contributor to the pathogenesis, receives limited attention. The significance of this therapeutic omission is striking when considered in light of increasing evidence suggesting that elements of a modern lifestyle represent environmental risk factors for IBD, which act by modifying the alimentary microbiota and thence, the developing immune system. Thus, modern lifestyle factors may link two of the main contributory factors to the pathogenesis of IBD (immunity and microbiota) and, in effect, represent proxy markers of microbial exposure during mucosal immune maturation.
Although antibiotics have served for targeting specific organisms, a bigger challenge is the manipulation of the broader microbial community and whether its composition might even be controlled in neonates at the time of colonization, to confer maximal biodiversity for optimal host–microbe interactions during development. Whether it be practicable, naïve, or futuristic, boosting natural microbial defenses is conceptually appealing to patients and represents a commercial growth area. Clinicians, therefore, need to be equipped with an essential understanding of the current status of the science to give an authoritative response to questions from informed and misinformed patients. The intent here is to present a brief overview of the potential scope for therapeutic manipulation of the microbiota.
Tyranny of terminology
Current strategies for manipulating the microbiota involve probiotics, prebiotics, and combinations (synbiotics), although phage viruses or topically active, narrow-spectrum antibiotics might in the future have a role in certain circumstances. Unfortunately, words and labels often outlive their usefulness or are rendered inaccurate by new discoveries. Recall that the term antibiotic, as originally defined, was a secondary metabolite of microbial origin, but in modern usage, it now covers naturally occurring compounds of nonmicrobial origin, such as sulfonamides, in addition to various synthetic and semisynthetic agents. Similarly, accepted definitions of probiotics are probably too restrictive, because they do not reflect usage of dead organisms, bacterial constituents including cell proteins, cell wall polysaccharides, and probiotic DNA or genetically modified organisms. To portray the wider scope of beneficial microbes and their products, the authors have adopted the umbrella term alimentary pharmabiotics. In the current context, an alimentary pharmabiotic is any material with potential health benefit that can be mined from host-microbe-dietary interactions in the gut. In practice, most pharmabiotics (and probiotics) are live whole organisms, usually lactobacilli or bifidobacteria, but other components of the commensal microbiota can be harnessed for their beneficial impact on the host.
Action in vivo—seeing is believing
Almost every component of probiotics from DNA to cytosolic protein and cell wall has been shown to influence mucosal immune or epithelial cells in vitro. This is hardly surprising, and there is no shortage of reports of such phenomena. However, exposure of cultured host cell lines or isolates to bacteria with which the responding cell has never seen or encountered before is hardly proof of a probiotic mechanism of action. Experiments demonstrating probiotic action in vivo are more convincing. Perhaps the best mechanistic demonstration of probiotic action has been in protection against infection. For example, in a murine model of infection with Listeria monocytogenes , the protective effect of the probiotic L salivarius UCC118 was shown conclusively to be due to production of an antimicrobial bacteriocin. More importantly, by using a luciferase-based reporter system and bioluminescence imaging, it was possible to track translocated Listeria infection in vivo and to visualize the protective influence of the probiotic in vivo. However, the same probiotic also protects against Salmonella infection. This is not due to the antimicrobial bacteriocin, however, because an engineered knockout of the bacteriocin was equally protective. To account for this aspect of probiotic action against pathogens, one has to look elsewhere: competitive inhibition and stimulation of innate and acquired immunity.
Not surprisingly, probiotics mimic the indigenous commensals, which are engaged in continual dialog with the host, particularly with enterocytes and dendritic cells. Remarkably, probiotics use a language that is similar to that used by pathogens when engaging with the host. They express microorganism-associated molecular patterns (MAMPs) that engage with the same pattern recognition receptors (PRRs) as do pathogens. This provides the molecular basis of protection by competitive exclusion. For other probiotics, protection against pathogen-induced tissue damage is conferred by stimulation of innate and acquired immunity and in particular, the generation of regulatory T cells. Once again, this aspect of probiotic action has been demonstrated elegantly in vivo. In a murine model of Salmonella infection, it was possible to image NF-κB activation in vivo and its attenuation by the probiotic Bifidobacterium infantis . The response was not specific to Salmonella and was also seen when injected lipopolysaccharide (LPS) was the inflammatory stimulus. It was associated with upregulation of enteric and systemic regulatory T cells, confirmation of which was shown in vivo by transferring the cells to naive recipients in whom LPS-induced activation of NF-κB was suppressed when the cells were derived from probiotic-fed, but not from placebo-fed, mice.
Skeptics of probiotic mechanism have argued that the average daily dose of most probiotics (10 9 –10 12 organisms) is such a tiny fraction of the total resident bacteria, that a meaningful impact on the intestinal ecosystem must be unlikely. Elegant studies with gnotobiotic mice, however, have shown that the resident bacteria adapt to the introduction of a probiotic species and to host dietary changes with alterations in gene expression.
Regrettably, in contrast to the consistent efficacy of probiotics in experimental animals, evidence for clinical efficacy of probiotics in people is relatively sparse, and confounded by a failure by many to appreciate that probiotics are not created equal. The complexity of host–flora interactions needs to be taken into account and tailored to suit the challenges of human disease. Despite this, there are success stories with probiotics in people.
Action in vivo—seeing is believing
Almost every component of probiotics from DNA to cytosolic protein and cell wall has been shown to influence mucosal immune or epithelial cells in vitro. This is hardly surprising, and there is no shortage of reports of such phenomena. However, exposure of cultured host cell lines or isolates to bacteria with which the responding cell has never seen or encountered before is hardly proof of a probiotic mechanism of action. Experiments demonstrating probiotic action in vivo are more convincing. Perhaps the best mechanistic demonstration of probiotic action has been in protection against infection. For example, in a murine model of infection with Listeria monocytogenes , the protective effect of the probiotic L salivarius UCC118 was shown conclusively to be due to production of an antimicrobial bacteriocin. More importantly, by using a luciferase-based reporter system and bioluminescence imaging, it was possible to track translocated Listeria infection in vivo and to visualize the protective influence of the probiotic in vivo. However, the same probiotic also protects against Salmonella infection. This is not due to the antimicrobial bacteriocin, however, because an engineered knockout of the bacteriocin was equally protective. To account for this aspect of probiotic action against pathogens, one has to look elsewhere: competitive inhibition and stimulation of innate and acquired immunity.
Not surprisingly, probiotics mimic the indigenous commensals, which are engaged in continual dialog with the host, particularly with enterocytes and dendritic cells. Remarkably, probiotics use a language that is similar to that used by pathogens when engaging with the host. They express microorganism-associated molecular patterns (MAMPs) that engage with the same pattern recognition receptors (PRRs) as do pathogens. This provides the molecular basis of protection by competitive exclusion. For other probiotics, protection against pathogen-induced tissue damage is conferred by stimulation of innate and acquired immunity and in particular, the generation of regulatory T cells. Once again, this aspect of probiotic action has been demonstrated elegantly in vivo. In a murine model of Salmonella infection, it was possible to image NF-κB activation in vivo and its attenuation by the probiotic Bifidobacterium infantis . The response was not specific to Salmonella and was also seen when injected lipopolysaccharide (LPS) was the inflammatory stimulus. It was associated with upregulation of enteric and systemic regulatory T cells, confirmation of which was shown in vivo by transferring the cells to naive recipients in whom LPS-induced activation of NF-κB was suppressed when the cells were derived from probiotic-fed, but not from placebo-fed, mice.
Skeptics of probiotic mechanism have argued that the average daily dose of most probiotics (10 9 –10 12 organisms) is such a tiny fraction of the total resident bacteria, that a meaningful impact on the intestinal ecosystem must be unlikely. Elegant studies with gnotobiotic mice, however, have shown that the resident bacteria adapt to the introduction of a probiotic species and to host dietary changes with alterations in gene expression.
Regrettably, in contrast to the consistent efficacy of probiotics in experimental animals, evidence for clinical efficacy of probiotics in people is relatively sparse, and confounded by a failure by many to appreciate that probiotics are not created equal. The complexity of host–flora interactions needs to be taken into account and tailored to suit the challenges of human disease. Despite this, there are success stories with probiotics in people.
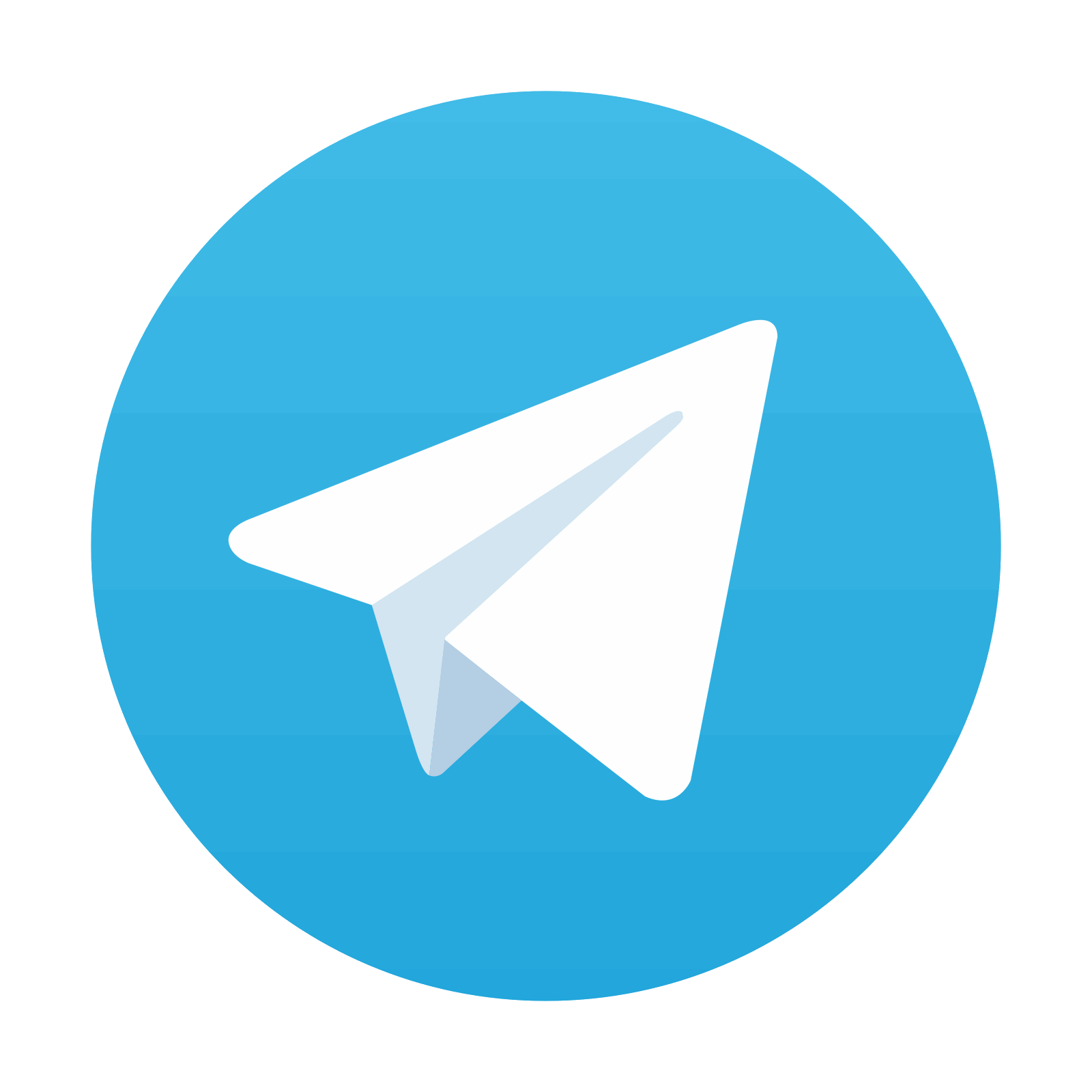
Stay updated, free articles. Join our Telegram channel

Full access? Get Clinical Tree
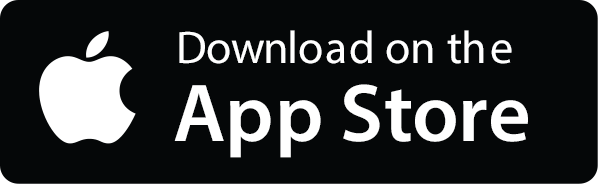
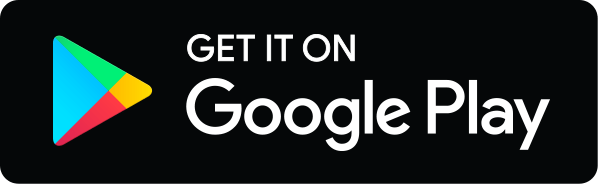