Abstract
Peritoneal dialysis (PD) is an important kidney replacement therapy option and is often the modality of first choice in a patient’s journey through end-stage kidney failure. PD relies on cycler-assisted or manual exchange of specialized dialysis solutions through a self-retaining Silastic tube into the peritoneal cavity with the peritoneal lining acting as the dialysing membrane across which exchange of metabolic end products, electrolytes, and free water takes place. PD works with residual kidney function to provide adequate clearance of metabolic waste, the clearance of which depends on the nature of the peritoneal membrane and the type, volume, and frequency of exchange of dialysis solution used. PD complications include malfunction or infection, and strict adherence to aseptic technique is needed. I is recommended that adequacy tests that include assessment of residual kidney function be carried out on a regular basis and, if targets are not met, a change in PD dose or potentially in kidney replacement modality be considered without delay.
Keywords
peritoneal dialysis, peritoneal dialysis fluids, peritoneal dialysis catheter, peritoneal effluent, peritoneal dialysis adequacy, peritonitis, CAPD, CCPD, ultrafiltration, assisted peritoneal dialysis
Peritoneal dialysis (PD), hemodialysis (HD), and kidney transplantation are the cornerstones of kidney replacement therapy (KRT). These modalities are not mutually exclusive, and, during a lifetime of therapy, patients may transfer from one to the other. In the 1950s and 1960s, at its inception, PD was used predominantly to manage acute kidney injury, while patients with end-stage kidney failure were treated almost exclusively by HD. The introduction of continuous ambulatory peritoneal dialysis (CAPD) in 1976 transformed this situation, and there was a dramatic rise in the use of PD internationally during the 1980s and 1990s. PD use among incident patients in the United States declined steadily from the mid-1990s to 2007, when only approximately 6% of incident dialysis patients performed PD; these have rebounded somewhat since then, with PD utilization of approximately 10% in 2014 among incident US patients undergoing dialysis. On December 31, 2014, the prevalence of various KRT modalities in the United States was approximately 64% HD, 7% PD, and 29% functioning kidney transplant.
Although in the past many comparisons have been made between peritoneal and HD, focus has shifted more recently to the site of dialysis delivery, namely home-based or in-center. Home-based therapies have advantages for many patients and are particularly popular in Canada, the Netherlands, Iceland, Finland, Denmark, Australia, New Zealand, Mexico, and Hong Kong, where more than 20% of the dialysis population receive home therapies. Among these countries, PD accounts for almost 80% of dialysis in Hong Kong and about 65% in Mexico. In the United States, while home dialysis has increased in recent years, its use remains low, particularly for home HD. Contrary to prior concepts of self-care requiring a fully able patient, PD has expanded in some countries to become the therapy of choice for the elderly and those with multiple comorbidities. In many regions, this has largely been possible by the introduction of assisted PD, with patients given varying degrees of assistance by a trained health professional in carrying out dialysis in their home. It frees them from the tedium of hospital or center-based HD and the travel involved.
Acute or “urgent-start” PD is used in many parts of the world, especially for single-organ acute kidney injury requiring dialysis support and in “late-presenters” with chronic kidney failure. Most major kidney units around the world have developed pathways for rapid peritoneal access to facilitate an early start of PD.
Principles of Peritoneal Dialysis
The Peritoneal Membrane
In PD, the visceral peritoneum serves as the dialyzing membrane. The visceral peritoneal membrane tightly covers the intestine and mesentery, whereas the parietal peritoneum lines the insides of the abdominal cavity. The membrane consists of a single layer of mesothelial cells overlying an interstitium in which the blood and lymphatic vessels lie. The mesothelial cells are covered by microvilli that markedly increase the nominal surface area of the peritoneum, which is approximately 2 m 2 . The effective peritoneal surface area available for dialysis, however, is estimated to be about one-third of this.
Solute Movement
PD occurs across the peritoneal membrane with solute and water exchange between the peritoneal capillary blood and the dialysis solution that is instilled into the peritoneal cavity. There is also some net fluid and solute resorption via the intraperitoneal lymphatics. Solute movement occurs as a result of “diffusion” and “convective transport,” whereas fluid shifts relate largely to “osmosis” created by the addition of osmotic agents to the dialysis solutions. During PD, solutes such as urea, creatinine, and potassium move from the peritoneal capillaries across the peritoneal membrane to the peritoneal cavity, while other solutes, such as lactate, bicarbonate, and potentially calcium, move in the opposite direction. Solute movement is mainly by diffusion and is therefore based on the concentration gradient between dialysate and blood. Solutes also move across the peritoneal membrane by convection, which is described as the movement of solutes as a result of fluid flux.
Fluid Movement
Standard PD fluids contain glucose in varying concentrations as the principal osmotic agent making the dialysate hyperosmolar in relation to plasma. When instilled in the peritoneal cavity this hyperosmolar solution causes net fluid removal (ultrafiltration) to occur. Dialysis fluid in the peritoneum is separated from capillary blood by three layers of tissue. These include the mesothelial cell layer, which offers little resistance to fluid and solute transport, the interstitium, which offers resistance mainly to large molecules, and the endothelium of the peritoneal capillaries, which is most active in restricting and regulating solute and fluid movement. The endothelial lining of the peritoneal capillaries has pores of three different sizes often referred to as the “three pore model.” Small pores (radius 40 to 50 A°) located between the endothelial cells are responsible for transport of low-molecular-weight solutes. These account for most of the pore area available for solute transport and contribute significantly toward peritoneal ultrafiltration. The large pores (radius 250 A°), thought to correspond to interendothelial gaps, account for just 5% to 8% of the ultrafiltration coefficient and occupy less than 0.5% of the total pore area. The ultra-small pores (radius 3 to 5 A°), composed mainly of Aquaporin-1, are permeable only to water and are located within the endothelial cells. These account for up to 40% of the total ultrafiltration. The volume of ultrafiltration depends on the concentration of glucose in the solution used for each exchange, the length of time the fluid dwells in the peritoneal cavity, and the individual patient’s peritoneal membrane characteristics (discussed later). With increasing dwell time, transperitoneal glucose absorption diminishes the dialysate glucose concentration and the osmotic gradient. Ultrafiltration is consequently decreased with longer dwell times, such as with the overnight exchange in CAPD or the long daytime dwell in automated peritoneal dialysis (APD), the most common form of which is referred to as continuous cycling peritoneal dialysis (CCPD).
The crucial physiologic components of the PD system are the magnitude of peritoneal blood flow and the permeability of the peritoneal membrane, neither of which is amenable to clinical manipulation. Also important are dialysate volume, dwell time, and number of exchanges per day, and these three variables can be manipulated to maximize solute and fluid removal. Various techniques and regimens have emerged as a consequence of increased understanding of peritoneal membrane transport characteristics or permeability in relation to the amount of solute and fluid to be removed.
Assessing Peritoneal Membrane Characteristics
PD effectively removes substances with low molecular weights, such as creatinine, urea, and potassium, that are not in the infused dialysis fluid. With increasing dwell time, solutes move across the peritoneal membrane toward concentration equilibrium, and the ratio of dialysate to serum urea levels approaches 1.0. Because the peritoneal membrane has a net negative charge, negatively charged solutes, such as phosphate, move across it more slowly than positively charged solutes of similar size, such as potassium. Macromolecules such as albumin cross the peritoneum by mechanisms that are not completely understood, but probably via lymphatics and through large pores in the capillary membranes. During a dwell period, the osmotic gradient created by standard dialysate within the abdominal cavity declines as the glucose is absorbed. In time this can result in net fluid reabsorption back into the systemic circulation because of the added effects of intraperitoneal hydrostatic pressure and intravascular oncotic pressure. Continuous lymphatic absorption also diminishes net fluid removal.
The rate of movement of small solutes between dialysate and blood differs from one patient to another. Peritoneal function characteristics are assessed by the peritoneal equilibration test (PET; Fig. 58.1 ). Classically, 2 L of dialysate containing 2.5 g/dL glucose is infused, and the ratio of dialysate to plasma creatinine (D/P Cr ratio) at the end of a 4-hour dwell is calculated. With this test, each patient’s peritoneal membrane can be categorized as having high (D/P Cr >0.81), high-average (0.65 to 0.81), low-average (0.50 to 0.65), or low (<0.5) peritoneal transport characteristics. Use of 2 L of dialysate containing 4.25 g/dL glucose during the same dwell period as in the PET permits assessment of ultrafiltration failure; an effluent volume of less than 2.4 L is diagnostic of ultrafiltration failure; accordingly, 4.25% glucose dialysis is often substituted for 2.5% in the PET.

Removal of fluid and solutes is highly dependent on transport characteristics as described by the PET ( Fig. 58.2 ). Patients with a high D/P Cr ratio (high or fast transporters) have rapid clearance of small molecules but poor ultrafiltration because of rapid glucose absorption and dissipation of the osmotic gradient between dialysate and blood. These patients require short-dwell PD regimens to achieve adequate fluid removal. In addition, because the volume of fluid removed also contributes to the solute clearance of equilibrated dialysate via convection, fast transporters also have reduced solute clearance over long dwells because of low drain volumes. Patients with a low D/P Cr ratio (low or slow transporters) have low clearance rates for solutes and usually require more dialysis exchanges or increased volume per exchange, or both, to avoid uremic symptoms once residual kidney function is lost. Ultrafiltration in this category of patient is relatively better. By virtue of the definition, most patients have high-average to low-average peritoneal transport, achieving adequate clearance and ultrafiltration on either CAPD or APD.

Peritoneal Catheters
Access to the peritoneal cavity for dialysis is achieved by means of a self-retaining catheter inserted into the abdominal cavity, usually under local anesthetic with or without sedation. General anesthesia often is reserved for patients with previous abdominal surgery and complicated insertions, particularly those patients in whom laparoscopic insertion is planned. The catheter can be inserted under direct vision through a mini-laparotomy, percutaneously using the Seldinger technique with a guide-wire, or with peritoneoscopic or laparoscopic guidance. Although there are numerous catheter designs, such as the Swan-neck catheter (said to undergo less catheter tip migration and fewer exit-site infections) and curled catheters, none offers a significant proven advantage over the double-cuffed Silastic Tenckhoff catheter. This original and simple design is still the most commonly used catheter. The intraabdominal portion of the catheter has multiple perforations in addition to the hole at the end through which dialysate flows. The deep cuff placed at the rectus muscle in the mid-line or just laterally and the extra-peritoneal portion of the catheter are tunneled through the subcutaneous tissue to exit the skin, pointing laterally and caudally. The superficial cuff is located inside the subcutaneous tunnel at least 2 to 3 cm from the exit site.
PD can be initiated immediately after catheter placement if it is urgently required; in this circumstance, exchange volumes are kept small, and the patient is kept recumbent to avoid dialysate leaks. Ideally, dialysis should be deferred for a few weeks after insertion to allow the surgical wound and exit site to heal properly. The surgical wound is secured in an occlusive dressing postoperatively and left untouched usually for 10 days, at which point patient training for exit-site care commences and training to perform CAPD or APD begins.
Techniques of Peritoneal Dialysis
Continuous Ambulatory Peritoneal Dialysis
CAPD is perhaps the simplest method for performing dialysis, involving the manual instillation of up to 3 L of dialysis fluid in the peritoneal cavity, through an indwelling abdominal catheter, three to five times a day. Typically this results in three to four shorter dwells during the day and a long dwell overnight. In adults the total volume of fluid exchanged in a day typically ranges from 8 to 10 L. Thus dialysis occurs continuously throughout the entire 24-hour period, and patients are free to go about their business in between exchanges. The prescription specifies the type of dialysis fluid, volume to be used, dwell time, and number of exchanges, and this prescription often varies according to patient size, peritoneal permeability, and residual kidney function.
PD fluid is instilled by gravity into the peritoneal cavity and drained out after a dwell period of several hours depending on the number of exchanges planned in the 24-hour period. The basic CAPD system, which has remained largely unchanged for almost 3 decades, consists of a plastic bag containing 0.5 to 3.0 L of dialysis fluid, a transfer set (tubing between the catheter and the plastic bag), and the permanent, indwelling Silastic catheter, which is implanted so that the tip of the intraperitoneal portion lies in the pelvis. Because the connection between the bag and the transfer set is interrupted three to five times a day to facilitate fluid exchange (approximately 1500 exchanges per year), the procedure must be carried out using a strict, aseptic, nontouch technique, which the patient or helper performs at home. The most common device in current use is based on a Y -shaped connection system. It comprises a filled bag and an empty bag of the same size with a Y -shaped junction, which can be connected to the PD catheter. Once the connection has been made, this device allows drainage of the effluent from the abdomen through the connection into the empty bag, before fresh dialysate is instilled. This ensures “flushing out” of any accidental “touch contamination” in the tubing before infusion of new fluid into the peritoneal cavity ( Fig. 58.3 ). This system reduces the risk of infection and also obviates the need for carrying an empty bag and transfer set, thus improving the psychologic aspects and quality of life of CAPD patients.

Automated Peritoneal Dialysis
APD is a broad term that is used to refer to all forms of PD that use a mechanical device (called a cycler ) for instillation and drainage of dialysis fluid. In its simplest form, APD was delivered in hospital, intermittently for prolonged periods of up to 24 hours, exchanging 20 to 60 L of dialysis fluid either in acute kidney failure or as a maintenance therapy in end-stage disease; this process was termed intermittent peritoneal dialysis (IPD). The technique is quite uncommon now, with most cycler-assisted exchanges carried out overnight by patients at home while asleep, thereby allowing freedom of movement during the day. APD regimens (illustrated in Table 58.1 ) can be tailored to individual need and include:
- •
CCPD, which provides three to four exchanges during the night and one during the day (a reversal of the CAPD regimen)
- •
Nocturnal intermittent peritoneal dialysis (NIPD), which provides rapid exchanges during the night, with no fluid in situ during the day (NIPD with “dry day”)
- •
Nocturnal PD plus two exchanges during the day to allow for increased small-solute and fluid clearance (NIPD with “wet day”)
- •
Tidal peritoneal dialysis (TPD), in which only a proportion of fluid in the abdomen is drained at the end of a cycle before it is filled again. The proportion of fluid removed can be set on the cycler and is usually between 50% and 85%. The lesser the proportion of fluid removed the more rapid is the cycling required and greater the total volume of dialysate used. The concept behind tidal PD is to allow continuous fluid-membrane contact to improve dialysis efficiency. In practice, however, tidal settings are often used with CCPD to relieve abdominal pain at the end of the drain cycle and to prevent catheter malfunction, rather than improve dialysis efficacy. When used for this purpose, 90% or more of the expected dialysate volume is typically removed.
Type of Dialysis a | Typical Number of Daytime Exchanges | Typical Number of Nighttime Exchanges | Volume of Exchanges (L) |
---|---|---|---|
CAPD | 2–3 | 1–2 b | 1.0–3.0 |
CCPD | 1 | 3–4 | 1.0–3.0 |
NIPD | 0 | 3–5 | 2.0–3.0 |
NIPD with “wet day” | 1–2 | 3–5 | 2.0–3.0 |
TPD | 0 | 4–20 | 1.0–2.0 |
IPD | 5–10 | 5–10 | 1.0–2.0 |
a All regimens except continuous ambulatory peritoneal dialysis use a cycler machine and are therefore variants of automated peritoneal dialysis.
b If an additional exchange is needed during continuous ambulatory peritoneal dialysis to achieve adequate dialysis, a mechanical exchange device can be used to perform the exchange during the night while the patient is asleep.
APD regimens usually entail an increased number of short-dwell exchanges to enhance solute and fluid removal. The cycler delivers a set number of exchanges over 8 to 10 hours, with the last fill constituting the long day dwell, which may be necessary to provide additional dialysis to achieve solute and fluid removal targets. The most obvious advantage of APD is that it eliminates the need for intensive manual involvement, because most of the dialysis occurs at night during sleep. In essence, APD entails only two procedures daily: an initial connection of the catheter to the machine and a disconnection at the end of dialysis. APD is increasingly being used in the United States and Europe in lieu of CAPD and is gaining popularity in developing economies as well. This trend may be related to the convenience of performing the dialysis connections and to newer cycler models, which are smaller, lighter, and less expensive than previously, and more attractive to patients. In the past, APD tended to be offered only to patients who had been identified by PET as “high transporters” and who therefore required shorter dwell times. However, lifestyle issues and freedom from daytime exchanges are now major factors in patient modality choice.
Assisted Peritoneal Dialysis
Although patients have been receiving assistance one way or another for as long as PD has been in existence, assisted peritoneal dialysis (aPD) is increasingly recognized as a distinct dialysis modality. Assistance may be provided by trained members of the family, paid nurses, or health care professionals, depending on the set-up of the health care system. Often, staff members at intermediate care facilities, assisted-living centers, and nursing homes are trained to carry out aPD for their residents. The advantages of aPD over in-center HD for the elderly include independence from hospital and slower removal of solute and fluid, reducing the likelihood of cardiovascular instability. In addition, older adult patients treated with aPD are less likely to suffer from malnutrition. Other patients who may be suitable for aPD include those with physical disabilities (e.g., rheumatoid arthritis), patients requiring terminal care (end-stage chronic disease, including malignancy and heart failure), those with learning disability, and others who are simply slow to learn because of other barriers.
Assistance can also be provided for CAPD, although this requires three or four visits daily. This model has been prevalent in France since the late 1970s. Assisted automated peritoneal dialysis (aAPD) is gaining in popularity as increased numbers of older patients are treated with dialysis. Varying degrees of assistance can be provided; one model includes one visit by the health care worker to the patient’s home every day. During the visit, the health care worker will strip down and set up the cycler (each morning); review and dress the PD catheter exit site if required; check blood pressure, weight, and blood glucose if the patient has diabetes; document patient progress and communicate any variance to the dialysis unit; and maintain adequate stocks of dialysis fluids and consumables. This level of assistance greatly simplifies the patient role to connecting himself or herself to the machine before going to bed at night and then disconnecting in the morning.
Peritoneal Dialysis Solutions
Glucose-Based Solutions
Standard PD solutions ( Table 58.2 ) contain varying concentrations of glucose as the osmotic agent, electrolytes such as sodium, magnesium, calcium, and chloride and buffers like lactate. Lactate was initially used as the buffer in preference to the more physiologic bicarbonate for technical reasons, specifically that the low pH of lactate prevents caramelization of the glucose while autoclaving for sterilization during the manufacturing process. The biocompatibility of these solutions has been intensively studied. There is no doubt that their unphysiologically low pH, high osmolarity, and presence of glucose degradation products (GDPs) generated during manufacture and autoclaving are harmful to peritoneal cells in vitro and are implicated in peritoneal neovascularization, collagen production, and peritoneal thickening, all of which may contribute to loss of function.
Type | Conventional | Low GDP a | Low GDP | Glucose Free | Glucose Free | Bicarbonate-Based | Bicarbonate-Based |
---|---|---|---|---|---|---|---|
Brand Name | Dianeal | Balance | Gambrosol Trio | Nutrineal | Extraneal | Physioneal | BicaVera |
Solute | Glucose | Glucose | Glucose | Amino Acid b | Icodextrin | Glucose | Glucose |
Solute Concentration c | 1.5%, 2.5%, 4.25% | 1.5%, 2.3%, 4.25% | 1.5%, 2.5%, 3.9% | 1.1% | 7.5% | 1.5%, 2.5%, 4.25% | 1.5%, 2.3%, 4.25% |
pH | 5.2 (4–6.5) | 7 | 5.5–6.5 | 6.6 (5.7–6.8) | 5.0–6.0 | 7.4 | 7.4 |
Osmolarity (mosm/L) | 345, 395, 483 d | 356, 399, 509 d | 357, 409, 483 | 365 | 282–286 | 344, 395, 483 | 358, 401, 511 |
Sodium (mmol/L) | 132 | 134 | 131–133 | 132 | 132 | 132 | 134 |
Chloride (mmol/L) | 96 | 100.5–101.5 | 96 | 105 | 96 | 95 | 104.5 |
Calcium (mmol/L) | 1.25–1.75 | 1.25–1.75 | 1.70–1.79 | 1.25 | 1.75 | 1.25 | 1.75 |
Magnesium (mmol/L) | 0.25 | 0.5 | 0.24–0.26 | 0.25 | 0.25 | 0.25 | 0.5 |
Lactate (mmol/L) | 40 | 35 | 39–41 | 40 | 40 | 15 | 0 |
Bicarbonate (mmol/L) | 0 | 0 | 0 | 0 | 0 | 25 | 34 |
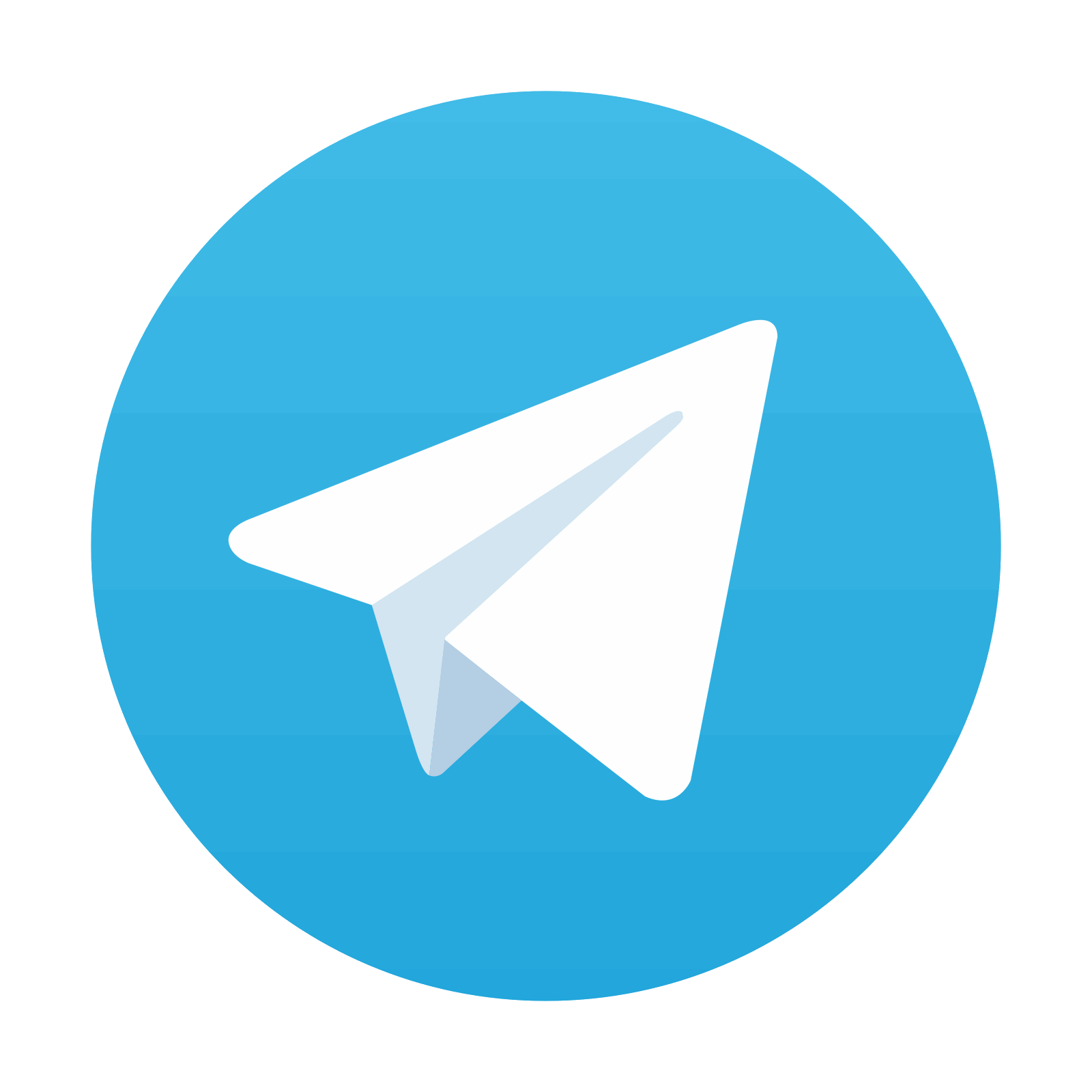
Stay updated, free articles. Join our Telegram channel

Full access? Get Clinical Tree
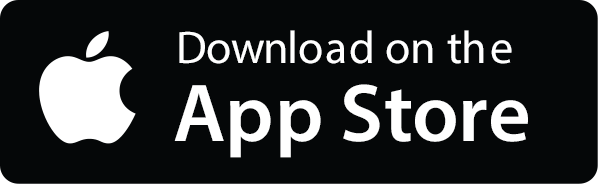
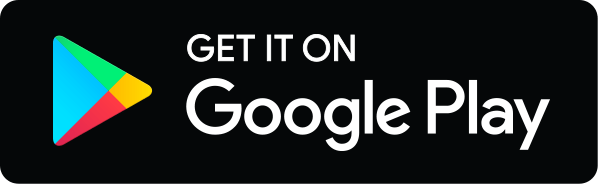
