29 Mohamed A. Elkoushy,1 Philippe D. Violette,2 & Sero Andonian3 1 Department of Urology, Suez Canal University, Ismailia, Egypt 2 Departments of Surgery and Health Research Methods, Evidence and Impact, McMaster University, Hamilton, ON, Canada 3 Division of Urology, McGill University Health Center, Montreal, QC, Canada Percutaneous instillation of topical agents started when Crowell disintegrated a cystine stone by instillation of alkaline solution into the kidney [1]. Before the advent of miniaturized fiber‐optic flexible ureteroscopes and nephroscopes, the nephrostomy tube provided a conduit for direct instillation of topical agents into the collecting system, thus minimizing systemic side‐effects. Chemolytic agents for stones were the original and most common agents. Currently, there are two other categories of topical instillation agents used: chemotherapeutic/immunotherapeutic agents as adjuvant treatment for upper tract transitional cell carcinoma (UTTCC) and antifungal agents. The advantages of direct instillation when compared with systemic therapy include increased duration of direct contact of the topical agents with the collecting system, which allows topical agents reach the target organ in appropriate concentrations. This leads to shorter therapy and avoidance of harmful systemic side‐effects [2]. In addition, these topical agents can be administered in patients with compromised renal function, inadequate urinary drainage, and high operative risk due to comorbidities [3]. However, it is important to note that there are several contraindications to initiating or continuing percutaneous instillation of topical agents. These include: positive urine culture, febrile patient, persistent flank pain, extravasation of irrigation fluid, or intrapelvic pressure >25 cmH2O [4]. With recent advances in endourologic technology, indications for instillation of topical agents have evolved and can be very useful for select patients. Although principles of instillation of topical agents into the collecting system are simple, minor errors in technique can result in catastrophic complications. Therefore, in addition to the urologist, both the nursing staff and the patient should be aware of the tell‐tale signs of complications and should inform the treating physician immediately to stop the instillation. Therefore, urologists should be familiar with techniques of instillation, indications and contraindications, success rates, and any potential side‐effects. In the present chapter, chemolytic agents (for stones), chemotherapeutic/immunotherapeutic agents for UTTCC, and antifungal agents will be reviewed. Topical instillation of agents into the collecting system depends on the principle of free flow of the solution throughout the collecting system, thus providing a continuous milieu of irrigant solution to the stone, UTTCC, or fungus ball. This can be achieved through several techniques: either retrograde fashion, percutaneous antegrade fashion, or a combination of both. The retrograde technique is the most simple and least invasive. It is done through a single ureteral catheter that is advanced beyond the level of the lesion or stone. The main disadvantage of this technique is the limitation of outflow through or around this small stent. This can result in a rapid elevation of intrapelvic pressures. Therefore, this technique of irrigation is not recommended. In an attempt to avoid this complication, Kuwahara and colleagues [5] used a computer‐controlled, pressure‐monitor intermittent pump (Figure 29.1a). This pump infuses fluid for 1 minute, holds for 30 seconds, and then drains for 9 seconds. If the pressure increases beyond the preset limit (15 cmH2O), the infusion stops. If the pressure increase is sustained for 5 seconds, the drainage valve opens. This system was not widely used because it was prohibitively expensive; because of this, its safety and efficiency were not properly evaluated. Without such a pump, a single ureteral catheter for irrigation is not recommended. Figure 29.1 Irrigation configurations for chemolysis. (a) Retrograde ureteral catheter with computer‐driven inflow/outflow. Source: Adapted from Fried and Smith [13] with permission. (b) Concentric catheters in kidney, ureter and bladder for transureteral irrigation. Source: Adapted from Dormia et al. [6] and Collins et al. [8]. (c) Dual Malecot catheters irrigation. (d) Nephrostomy inflow and universal/endopyelotomy stent outflow irrigation for struvite stones. (e) Circle tube for irrigation chemolysis. (f) Coaxial technique of ureteral catheter through a Foley catheter. (g) Coaxial technique of ureteral catheter through a Foley catheter. (h) Retrograde ureteral catheter inflow and nephrostomy outflow. (i) Constant‐pressure renal pelvis irrigation system: a 5 Fr open‐end ureteral catheter inserted through a small hole in the urine bag tube while the tip of the ureteral catheter is kept at the end of the nephrostomy catheter. The urine bag tube is placed 20 cm above the kidney while irrigating at 100 ml/h. Source: Adapted from Nakamura et al. [21]. CVP, central venous pressure. Several other techniques of retrograde instillation have been described. One technique proposed by Dormia et al. [6] utilizes concentric catheters placed in the ureter to facilitate the outflow from the kidney into the bladder (Figure 29.1b). Another less‐efficient technique is by vesicoureteral reflux in a patient with an indwelling ureteral stent after ureteral meatotomy [7]. Recently, a high‐flow low‐pressure irrigation system was described that uses an 8 Fr pigtail catheter through a 12/14 Fr ureteral access sheath (Applied Medical Systems, Rancho Santa Margarita, CA, USA). In an in vitro study, this system was found to be associated with significantly higher flow rates and lower pressures when compared with a single nephrostomy tube without ureteral catheters [8]. The ideal inflow and outflow is achieved by percutaneous antegrade fashion through the use of two nephrostomy tubes. For example, two Malecot catheters (16 or 18 Fr) could be used (Figure 29.1c). This configuration is versatile and can be used in most patients. In patients with struvite stones, which tend to form large, irregular pieces on fragmentation, an endopyelotomy stent (14/8.2 Fr) provides excellent two‐way outflow, decreases the volume of fragments that enter the ureter and prevent its obstruction [9] (Figure 29.1d). Another method of preventing ureteral obstruction is insertion of an indwelling double pigtail catheter when two Malecot nephrostomy tubes are used. A simpler method of inflow and outflow in antegrade fashion through two nephrostomy sites is the use of a circular tube (Figure 29.1e). Two coaxial techniques of irrigation have been described. Hare and McOmish [10] described a renal pelvic lavage set to irrigate different areas of the pelvis through a single nephrostomy site allowing both inflow and outflow (Figure 29.1f). Once the Teflon sheath is in place, an inner 6.3 or 8.3 Fr pigtail catheter is directed at the stone for inflow. Outflow, which is limited, occurs through the surrounding sheath and down the ureter. The second coaxial technique of irrigation works well for upper ureteral stones [11, 12]. It is set up by inserting either a 5 Fr ureteral or angiogram catheter over a wire through the lumen of an 18 Fr Foley catheter, which serves as a nephrostomy tube (Figure 29.1 g). The small inner catheter, which provides inflow, can be advanced so that its tip is adjacent to the stone, whereas the Foley catheter provides outflow. This is an effective method for dissolving upper ureteral stones. A simpler technique of irrigating the renal pelvis through a single nephrostomy site is the use of a three‐way Foley catheter [13]. One combination of both antegrade and retrograde techniques can be accomplished through the insertion of a percutaneous nephrostomy (NT) tube and ureteral catheter. The NT acts as an ideal outflow while the ureteral catheter is used for instillation (Figure 29.1 h). However, using the combination of a ureteral catheter, a urethral catheter and a nephrostomy tube severely decreases patient mobility. Therefore, a modified approach can use an NT to instill agents and an indwelling double pigtail ureteral catheter as a drainage catheter. The drawback of this approach is that there is much less efficient outflow through a ureteral catheter than a nephrostomy tube. Therefore, this modification would be ideal for small stones that dissolve in a short period of time, such as uric acid stones. All the techniques described above should follow appropriate safety principles. Patients should have preoperative urine cultures and be treated with culture‐specific antibiotics. Urine cultures should be negative prior to initiating irrigation and patients should be kept on antibiotics throughout the irrigation. In addition, once the tubes are inserted, contrast studies should be performed 24 hours later to rule out extravasation. The irrigation should start slowly with warm saline at a rate of 30 ml/h. When the patient remains pain‐free and afebrile, the rate is doubled every 2–4 hours until the target rate of 120 ml/h is reached [13]. After 12–24 hours of saline irrigation, the active agent is instilled at a rate of 30 ml/h and increased sequentially to 120 ml/h. There are several advantages to warming the irrigation solution. It is more comfortable for the patient, it avoids hypothermia, and it may increase the effectiveness of therapy [14–16]. It is important to have an intrapelvic pressure‐monitoring system in all of the techniques described. If renal pelvic pressures are uncontrolled, pyelovenous and pyelolymphatic backflow leads to systemic absorption. Subsequently, the patient may suffer agent‐specific systemic toxicities or changes in serum chemistries when the irrigant solution is nontoxic [17]. The intrapelvic pressure should not be allowed to rise above 25 cmH2O in order to prevent pyelovenous backflow [18]. The simplest method is the use of a central venous pressure (CVP) manometer connected to the inflow line. This is readily available and familiar to the nursing staff. Unfortunately, this is an open system that is prone to contamination or overflow spills. Furthermore, it requires continuous monitoring since there is no preset pressure alarm or automatic shutoff for high pressures (>15 cmH2O). A better alternative is a closed continuous infusion pump that provides a continuous display of the intrapelvic pressure and will sound an alarm and shut off if the preset limit is exceeded (CareFusion, San Diego, CA, USA) [6]. Regardless of which pump is used, the patient should be taught how to immediately turn off the pump in case of flank pain or fever. Small‐caliber percutaneous instruments (SCPI) provide a new range of tools for kidney stone treatment. The impact of combined high‐pressure irrigation inflow and simultaneous sensor‐controlled suction on irrigation turnover and intrarenal pressure in SCPI was recently investigated. A 9.5 Fr Amplatz sheath was introduced through the lower calyx and an 8 Fr ureteric catheter was inserted through the ureter of a nonperfused porcine kidney. Another 8 Fr ureteric catheter was inserted through the upper calyx, and irrigation was performed using a pressure‐controlled, combined irrigation/suction pump. Analysis of the intrapelvic pressure was performed by different irrigation pressures with and without sensor‐controlled suction through the ureteric catheter [19]. After initiating irrigation flow, the intrarenal pressure reached a plateau within seconds without any pressure peaks during the whole experiment. This sensor‐controlled suction increased fluid turnover and decreased intrarenal pressure in SCPI. In addition, such a purging effect prevented collapse or hyperdistention of the collecting system, especially in small‐caliber instruments. However, SCPI has not been tested in humans yet. Similarly, Colli et al. found that application of a 10 Fr ureteral dual‐lumen catheter in a porcine model produced adequate retrograde drainage with low intrarenal pressures at high infusion rates (up to 80 ml/min) [20]. This experiment supports the use of separate channels for inflow and outflow during retrograde infusion to maintain intrarenal pressures around 5 cmH2O. Nakamura et al. reported a novel constant‐pressure irrigation technique for a case of a left renal pelvic tumor which developed postradical cystectomy, right nephroureterectomy, and left ureterectomy in a patient with multiple urothelial tumors. The tumor was endoscopically ablated through a nephrostomy tract followed by mitomycin C irrigation [21]. The authors designed a novel constant‐pressure irrigation system for effective and safe irrigation into the closed‐space renal pelvis. Through a hole in the urinary drainage bag tube, a 5 Fr open‐ended ureteral catheter was inserted, and the tip of the catheter was kept at the tip of the nephrostomy tube. The ureteral drainage tube was placed 20 cm above the kidney level to continuously irrigate the renal pelvis with mitomycin C for six‐weekly treatments of 1 hour each time. The patient has been recurrence‐free for one year (Figure 29.1i). Stone dissolution through chemolysis remains an option in the treatment of urolithiasis even with the advent of more sophisticated modalities. Chemolytic agents can achieve benefit through either primary or secondary dissolution. Primary dissolution is when chemolytic agents are used as initial therapy to dissolve stones completely or partially. This may be an option in patients unfit for general anesthesia. Secondary dissolution may be useful in patients with residual fragments after some other primary therapy. In this context chemolysis may render the patient stone‐free, thus reducing the potential for recurrences or more invasive interventions. For effective chemolysis, the irrigant and irrigation system must have several important characteristics. First, the irrigant must be compatible with biological tissue and be of limited toxicity. Second, it should have minimal systemic absorption in order to limit potential toxicity. Third, the irrigant should dissolve calculi and their fragments quickly to reduce treatment burden for the patient. Fourth, solutions used for irrigation must not precipitate, causing secondary stone formation. In addition, an ideal irrigation system must provide for free flow of irrigant to a targeted area, while maintaining low renal pelvic pressures to prevent bacteria from entering the bloodstream and to avoid renal damage [17]. Unfortunately, stone dissolution through chemolysis has several limitations. Some stones may require long treatment periods, which may necessitate prolonged hospital admission and patient discomfort. The efficacy of stone dissolution is dependent on many stone‐specific factors. For example, uric acid and struvite amorphous minerals dissolve much faster than highly crystalline salts such as calcium oxalate. Likewise, stone size and shape will determine the rate of dissolution. Large stones will dissolve more slowly than the same volume of smaller stones because the surface area is greater in the latter [22, 23]. These variables can make it difficult to predict the necessary length of treatment a priori. In addition, the choice of chemolytic agent will depend on stone type. This requires that stone composition be determined prior to chemolysis. When stone fragments are not available, surrogate methods of determining stone composition, such as CT characteristics or the composition of previous stones, must be used to determine the agent of choice [17, 24, 25]. Almost all individuals who form uric acid stones have persistently low urinary pH (<5.5) and most excrete normal amounts of uric acid [26, 27]. The acid–base properties of uric acid stones render them amenable to dissolution therapy. Uric acid is a weak organic acid with a pKa of 5.35 in urine at 37 °C. At this pH, half is present as the undissociated acid and half as the urate anion. Since urate is more soluble than uric acid, raising the urinary pH within the physiological range has a profound effect on how much uric acid/urate can be dissolved. For example, a urine sample containing 800 mg/l total urate will have 600 mg/l uric acid at a pH of 5.0 but less than 100 mg/l at pH 6.5. The latter figure is well below the solubility limit of uric acid so that a solution above pH 6.5 can dissolve a uric acid calculus [18]. Any solution above pH 7 is likely to be effective but higher urinary pH solutions may have a tendency to calcify the stone, particularly if sodium bicarbonate is used as the base [28]. Increasing the urinary volume further enhances the therapeutic efficacy of alkaline medications [29]. In addition, decreasing the oral purine load from dietary sources can effectively help treat patients because 40– 60% of excreted uric acid is derived from exogenous sources. Regardless of the method chosen, urine pH should be monitored with pH strips to optimize therapy. Serum and urine uric acid levels are also important to follow, especially in those with recurrent calculi. To identify those most likely to respond to dissolution therapy, Reichard et al. found that patients with 100% uric acid stones were older, heavier, and had a higher serum uric acid level, and lower urinary pH than those with mixed stones, while urinary uric acid was not significantly different among groups [30]. However, it is unclear whether these characteristics alone will provide enough specificity to predict uric acid stones. Systemic alkalinization is generally achieved with oral or intravenous therapy. The most popular agent is sodium bicarbonate, and the dose is 650–1000 mg three to four times daily. An alternative preparation, if the sodium load is too great, is potassium citrate administered as 15–30 mEq three to four times daily. Specifically, oral alkalization therapy for uric acid calculi has been established in the literature as an effective, totally noninvasive therapy [31]. It is generally effective for stones of 8–52 mm long with success rates of 62–72% in adults within six weeks to six months [32, 33]. Furthermore, oral alkalization is well tolerated and effective in children with uric acid stones, where it eliminates the need for shock‐wave lithotripsy (SWL) in up to 73% of those children. One randomized controlled trial (RCT) showed comparable stone‐free rates for dissolution therapy and a single session of SWL (72.9% vs. 82.1%, P = 0.31). Children undergoing dissolution therapy received potassium sodium hydrogen citrate at a dose of 1 mEq/kg per day for 1–3 months [34]. Several other oral therapies have been suggested to facilitate dissolution therapy. Sterrett and colleagues [35] demonstrated that acetazolamide, a carbonic anhydrase inhibitor, was effective in increasing the urinary pH in patients with uric acid and cystine stone formation who were already taking potassium citrate. Caution must be applied when prescribing acetazolamide, because it could be poorly tolerated and can induce calcium phosphate stone formation. A dose of 250–500 mg is taken at bedtime to maintain urine alkalinity during sleep. In cases of hyperuricosemia and hyperuricosuria, allopurinol should be added to inhibit the production of uric acid through inhibition of xanthine oxidase enzyme. Intravenous alkalinization is more effective than the oral route. Urinary pH is elevated more quickly, to a higher degree, and is more reliable. This technique, however, is typically reserved for hospitalized patients with acute episodes of (renal) colic and obstruction. One‐sixth molar lactate solution has been demonstrated to dissolve uric acid stones even when they are causing ureteral obstruction [36]. Lactated Ringer’s solution with one to two ampoules of sodium bicarbonate added per liter is an acceptable alternative. The infusion rate (usually 50–100 ml/h) is titrated to a urinary pH of 7.0–7.5, which occurs within 4 hours. Cardiac and hypertensive patients must be monitored for the large sodium load in these solutions. Since the urinary pH can usually be raised to at least 6.5 with oral or intravenous alkaline salts, medical therapy is ordinarily the treatment of choice for uric acid stones [18, 27, 29]. There are clinical situations in which oral or intravenous therapy is not possible, such as in patients who are noncompliant, or have chronic renal failure or an ileostomy. These patients may benefit from direct chemolytic therapy. Additionally, if a large amount of residual fragments is present after an initial therapy, direct chemolysis is a viable option. The most common alkalinizing agents are sodium bicarbonate solution (pH 7.0–9.0), tromethamine (THAM, pH 8.6), and THAM‐E (pH 10.5). Sodium bicarbonate solution is inexpensive and prepared easily by adding 2–4 ampoules (44 mEq each) to each liter of irrigant solution (normal saline solution or water). With this solution, there is no value in increasing the final urinary pH >7.5. In fact, higher elevations may be detrimental to stone dissolution because phosphatic and sodium urate encrustations may form a coating on stones [37]. The THAM solutions are more effective and do not cause such mineral deposits. It has been shown in animal models that THAM results in more rapid stone dissolution than sodium bicarbonate [28]. THAM‐E is slightly more effective than THAM because of its higher pH but it is also responsible for more irritation and potential damage to the epithelium [38, 39]. Therefore, for uric acid chemolysis, THAM appears to be the agent of choice [18] (Table 29.1). Table 29.1 Results of direct chemolysis for uric acid and cystine stones. Source: Adapted from Fried and Smith, 2007 [13]. a Three patients had only small clinically insignificant fragments. SWL, extracorporeal shock‐wave lithotripsy. If a uric acid staghorn calculus is present, pretreatment with SWL may increase the stone surface area to facilitate dissolution. Unlike struvite and cystine stones, it is unusual to need prolonged irrigation to manage uric acid stones. Cystinuria is an autosomal recessive disorder of dibasic amino acid transport in the kidney that leads to crystallization of poorly soluble cystine in the urine [51]. Between 3% and 59% of those who are homozygous for the trait will produce stones [52]. The disease is more severe in men than in women in terms of early appearance and number of produced stones. Although the incidence of cystine stones is only about 0.9%, cystine stones rank third in terms of rate of recurrence [53]. Treatment of cystine stones continues to be one of the most challenging therapeutic dilemmas in endourology because of a high frequency of recurrence, resistance to SWL, difficulty in localization, and the insufficient effect of oral chemolysis [44]. Because of the difficulties in treating cystine stones, much emphasis has been placed on prophylaxis. The solubility of cystine, like all amino acids, is pH‐dependent. Therefore, the production of alkaline urine can prevent cystine precipitation in the urine. A significant increase in cystine solubility begins to occur when urinary pH exceeds 7. For example, at a pH of 8, cystine solubility increases to approximately 1000 mg/l [54]. However, a significant drawback of urinary alkalinization is increased risk for calcium phosphate precipitation with pH above 7.5 [55]. For this reason, urinary pH of 7.5 is recommended [54]. It is recommended that this alkaline urinary pH must be maintained at night while the patient sleeps. The use of the potassium salts of citrate and bicarbonate may be more effective than the analogous sodium compounds because the presence of a sodium ion increases cystine excretion in the nephron. Because of this, a low‐sodium diet may be useful in the management of cystinuria [56]. Cystine is composed of two cysteine molecules joined by a disulfide bond and its dissolution requires alkalinization and splitting of the disulfide bond. This can be accomplished through either oral therapy or direct chemolytic therapy. D‐Penicillamine, the first oral agent used for cystine stones, is a disulfide exchange resin that facilitates separation at the disulfide bond through the thiol exchange reaction, forming mixed disulfides, and also decreases urinary excretion of cystine. The exchange reaction forms penicillamine disulfide–cysteine, which is 50 times more soluble than cystine [57] and is readily cleared through the urine. D‐Penicillamine is administered as 1–2 g in four divided doses, starting with a small dose and increasing as tolerated. Side‐effects of the drug include leukopenia, thrombocytopenia, gastrointestinal disturbances, skin rashes, proteinuria and nephrotic syndrome, neuropathies, and abnormalities of taste and smell. D‐Penicillamine has been reported to dissolve even large stones when combined with alkalinization but its potential side‐effects severely limit its usefulness. α‐Mercaptopropionylglycine (MPG) is another disulfide exchange resin with fewer side‐effects than D‐penicillamine and appears to be more effective [58]. The usual dose of MPG is 600–1800 mg daily in four divided doses. Simultaneous treatment with oral sodium bicarbonate increases the effectiveness of this preparation because the disulfide exchange reaction is more active at higher pH levels. Oral acetylcysteine, MPG, and D‐penicillamine are much more effective in preventing the formation of cystine calculi than dissolving them. When used for direct chemolysis, however, they are potent dissolution agents. During direct irrigation the solution bathing the stone will have a much higher concentration of the active agent. The pH of the solution is likewise more easily controlled, thereby facilitating the thiol exchange reaction [13]. Chemolysis monotherapy would require lengthy durations of irrigation for most stones. Approximately 9 days are required to dissolve a 1 cm cystine calculus. This irrigation time could be diminished when combined with SWL or percutaneous nephrolithotomy (PCNL), which reduce the stone burden and increase the surface area of the remaining fragments. Stones <1.5 cm may be treated initially with SWL since it increases the stone surface area and decreases dissolution time by nearly 50% [22]. If successful fragmentation occurs, repeat SWL or medical management may be sufficient as follow‐up therapy. Otherwise chemolysis alone or combined with PCNL is appropriate. For stones >1.5 cm, PCNL is the initial procedure of choice. Residual calculi, which are likely to remain, can be effectively treated with chemolysis and additional PCNL or SWL sessions. Simultaneous oral therapy should be implemented in all cases [13] (Table 29.1). If the stones appear extremely resistant to therapeutic measures, the possibility of a mixed stone should not be overlooked. Hydroxyapatite, struvite, calcium oxalate, and calcium phosphate contaminants have been found to be present in 14–46% of cystine calculi analyzed in some studies [59]. In summary, although cystine urinary calculi continue to present a formidable challenge to urologists, successful eradication without open surgery may be accomplished with chemolytic techniques in conjunction with minimally invasive fragmentation procedures. Struvite stone formation typically occurs in patients with recurrent urinary tract infection and retained urine. Predisposing factors include urinary tract obstruction, chronic indwelling catheter, urinary diversion, and neurogenic voiding dysfunction [60]. Most commonly, staghorn stones are composed of a mixture of struvite (magnesium ammonium phosphate) and calcium carbonate apatite. In fact, hypercalciuric patients begin with calcium oxalate stone formation and develop superimposed infection with struvite deposition [61, 62]. Struvite calculi are typically referred to as “infection stones” because of their strong association with urinary tract infections caused by such bacteria. Silverman and Stamey [63] identified Proteus as the dominant microorganism in 72% of isolates from stone formers. Urease produced by such bacteria leads to hydrolysis of urea to ammonium, hydroxide, and bicarbonate, which increases the pH of the urine (pH >7.2). This alkaline urine, in the presence of trivalent phosphate, results in struvite crystal formation [64]. Other urease producers include Klebsiella, Pseudomonas, and Staphylococcus species [63, 65]. The most ubiquitous uropathogen, Escherichia coli, only rarely produces urease and thus is an infrequent cause of staghorn calculi [66]. Struvite stones are characterized by their large size and exceptionally rapid growth. It is not uncommon for a staghorn calculus to involve the entire renal pelvis and calices, nor for it to be formed within a four‐week period [60]. Management of struvite stone disease is one of the most frustrating problems in urology as efforts to eradicate such stones often have suboptimal success rates. The problem is not only ridding the patient of the calculi but also sterilizing the urine. Persistence of infection was estimated to occur in approximately 40% and is believed to be responsible for many of the stone recurrences [67]. The presence of small stone particles with embedded bacteria serves as a nidus for new stone formation and rapid recurrences. Therefore, postoperative irrigation therapy has been proposed to potentially reduce struvite stone recurrences [68, 69]. Medical dissolution therapy for struvite and carbonate apatite stones has been described as a second‐line treatment option. Oral chemolysis is available primarily in the form of urease inhibitors. Acetohydroxamic acid, the most commonly used agent, was identified as an irreversible urease inhibitor by Fishbein and Carbone [70]. The drug acts synergistically with several antibiotics, sterilizing the urine more rapidly, and its clinical usefulness has been demonstrated in double‐blind studies, although serious side‐effects precluded its use in patients even though they are reversible and dose‐related [71–73]. The usual dose is 250 mg orally three times daily to be reduced to twice daily for mild renal impairment, and it is contraindicated in patients with severe renal insufficiency and in pregnancy. Most common side‐effects reported were hemolytic anemia, neurosensory deficits, and thrombophlebitis. Headache, gastrointestinal upset, weakness, and flushing sensation after alcohol ingestion have also been reported [74]. Nevertheless, it is the only US Food and Drag Administration (FDA)‐approved urease inhibitor available today [75]. Systemic oral chemolytic agents are seldom effective in reducing stone burden on their own and direct chemolysis of struvite calculi is a far more effective method of dissolution. Suby and colleagues [76, 77] presented the first application of direct chemolysis to struvite calculi where the initial solution was found to be too irritant for urothelium to be clinically used. Suby’s solution G, formed by addition of magnesium to the previous formulation, has similar efficacy with decreased mucosal irritability (Table 29.2). Hemiacidrin (renacidin) is a multi‐electrolyte solution introduced by Mulvany [78] by modification of Suby’s solution. The acids present in this solution provide hydrogen ions and citrate to form soluble complexes with phosphate (phosphoric acid) and calcium (calcium citrate) from the stone (Table 29.3). Magnesium undergoes ion exchange with calcium present in the stone, enhancing dissolution while reducing irritation. The solubility of struvite is markedly increased at a pH <5.5, which occurs with each of the three variants of Suby’s solution. This finding was confirmed in a recent in vitro study by Jacobs et al. [79]. The success rate of these medications in the literature has been reported to occur in the range of 68–80% [80]. Table 29.2 Composition of different chemolytic agents. Source: Adapted from Fried and Smith, 2007 [13]. Table 29.3 Results of direct chemolysis of struvite calculi. Source: Adapted from Fried and Smith, 2007 [13]. a Includes partial dissolutions. SWL, extracorporeal shock‐wave lithotripsy; NA, not applicable; PCNL, percutaneous nephrolithotripsy. Infectious complications are known to occur as a result of dissolution therapy. Renacidin and Suby’s solutions have been implicated as the cause of urosepsis and death, and the FDA banned the use of renacidin irrigation for upper urinary tract stones on June 13, 1963 [90]. Urosepsis is thought to occur due to the release of large amounts of viable bacteria from stones as they dissolve. Therefore, it is critically important to stop treatment if fever occurs and until any infection has been adequately treated. The presence of catheters and extensive use of antibiotics can also facilitate fungal infection. Replacing the irrigation fluid with amphotericin B (50 mg/500 ml H2O) at a rate of 125 ml/h generally clears the funguria in less than 72 hours. Chemolysis irrigation can resume once urine cultures are negative for 24 hours. Calcium‐based calculi constitute about 80% of all urinary tract stone calculi of which about 80% are calcium oxalate stones [91], and yet they are the least amenable to chemolysis. The strong acids required to dissolve this compound cannot be safely used in humans. Only chelating agents have successfully been used in vivo to dissolve these types of stones. Ethylene‐diamine‐tetraacetic acid (EDTA) is the most commonly used solvent, and has shown moderate success in humans [92, 93] in spite of the significant urothelial injury reported [47]
Percutaneous Instillation of Chemolytic, Chemotherapeutic, and Antifungal Agents
Introduction
Techniques of irrigation/instillation
Retrograde approach
Antegrade approach
Combined approach
New concepts
Chemolysis of urinary calculi
Uric acid stones
Systemic agents
Chemolytic agents
Study
Irrigation solution
Dissolved/attempted
Duration of irrigation (days)
Adjuvant treatment
Uric acid calculi
Chemolysis alone
Hedgcock et al. [12]
Sodium bicarbonate
11/11
3–21
Gordon et al. [40]
THAM
3/3
7–10
Ansari et al. [41]
Sodium lactate
3/3
7–14
Chemolysis with SWL
Lee et al. [42]
Sodium bicarbonate
5/8a
2–7
Cystine calculi
Schmeller et al. [22]
N‐Acetylcysteine + sodium bicarbonate
1/1
26
Smith et al. [43]
1/1
30
Aabech and Andersen [44]
THAM‐E with/without N‐acetylcysteine
2/2
3–9
Surgery with/without SWL
Kachel et al. [45]
3/9
–
3 Partial dissolution, SWL
Dretler et al. [46]
7/11
6–42
with/without PCNL
Kane et al. [47]
Mercaptopropionylglycine
5/5
24–88
1 Pyelolithotomy, 1 bladder stone, urokinase added to irrigant
Hayase et al. [48]
THAM‐E
0/3
25–37
3 Partial dissolutio, 1 nephrolithotomy
Crissey and Gittes [49]
1/2
5
1 Ureteral, 1 renal stone
Stark and Savir [50]
Penicillamine
1/1
180
1 Pyelolithotomy
Cystine stones
Struvite stones
Compound
Suby’s solution
Renacidin
G
M
Citric acid (g)
32.3
32.3
28.2
Magnesium oxide (g)
3.8
3.8
0
Sodium carbonate (g)
8.84
8.84
0
Calcium carbonate (g)
0
0
1.0
D‐Gluconic acid (g)
0
0
5.0
Magnesium hydrocarbonate (g)
0
0
14.8
Magnesium acid–citrate (g)
0
0
2.5
Water (ml)
1000
1000
1000
pH
4.5
4.0
4.0
Irrigating solution
Primary (1°) or
adjuvant (2°)
Dissolved/attempted
Duration of irrigation (days)
Comment
Suby’s solution G
Smith et al., 1979 [11]
1°
2°
2/2
1/1
5–9
NA
Patients had ileal conduits
Suby and Albright, 1943 [77]
1°
2°
3/7
1/1
10–90
Renacidin
Nemoy and Stamey, 1971 [4]
1°
2°
3/3
4/4
12–30
1–14
Prophylactic irrigation used
Dretler and Pfister, 1983 [17]
1°
16/23
6–45
Spirnak et al., 1988 [23]
2°
9/11
2–8
SWL 1.2 times per kidney
Silverman and Stamey, 1983 [63]
2°
5/11
4.5 (avg)
Rudman et al., 1983 [74]
1°
2°
2/2
18–21
14–17
2–26
9% recurrence at 66 mo follow‐up
Mulvaney, 1959 [78]
1°
17/31a
NA
Kidney/bladder/ureteral stones
Dretler et al., 1984 [46]
1°
6/8
7–20
Russell, 1962 [81]
1°
2/2
10–13
Kohler, 1962 [82]
1°
2°
4/5
4/6
14–115
12–120
Brock et al., 1980 [83]
1°
3/4
2–9
Patients had ileal conduits
Palmer et al., 1987 [84]
1°
2°
7/9
7/9
6–69
3–30
Outpatient treatment performed
Blaivas et al., 1975 [85]
2°
8/11
2–21
Jacobs and Gittes, 1976 [86]
2°
7/11
2–22
Nemoy and Stamey, 1976 [87]
2°
2/2
8–15
Kleine et al., 1982 [88]
2°
8/9
4–34
Angermeier et al., 1993 [89]
2°
3/3
1–8
SWL and PCNL irrigation with pressure control pump
Totals
1°
2°
65/96
77/100
14 (avg)
9 (avg)
Calcium stones
Stay updated, free articles. Join our Telegram channel

Full access? Get Clinical Tree
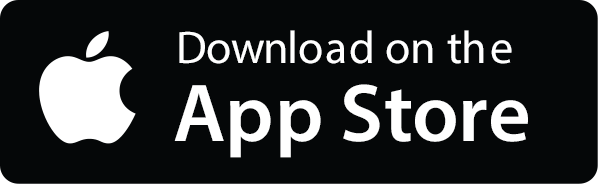
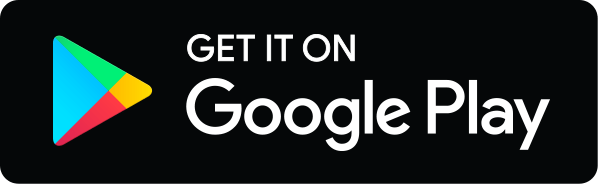