126 David N. Siegel1 & Alok A. Anand2 1 Zucker School of Medicine at Hofstra‐Northwell, New Hyde Park, NY, USA 2 Department of Radiology – Eastern Connecticut Health Network, Manchester, CT, USA Renal cell cancer (RCC) represents 2% of adult cancers and is the third most common urinary tract malignancy [1–3]. Disease epidemiology is poorly understood, although smoking, obesity, and acquired cystic renal disease have been identified as risk factors [1]. The natural history of renal cancer is typically one of a long period of indolent growth in asymptomatic patients. Clinical presentation on the basis of symptomatology usually indicates advanced disease, with metastasis likely, portending a poor prognosis. The advent of modern imaging, combined with changing diagnostic algorithms and practices, has led to a serendipitous increase in diagnosis of small renal tumors. It is estimated that as many as 70% of diagnosed renal tumors are stage T1a. Small renal masses within this size range have been shown to grow at an average rate of 0.3–0.4 cm/year and exhibit a very low rate of progression to metastatic disease [1–4]. Of small renal masses, 80% represent RCC, but approximately 20% are benign [1]. This raises the management dilemma of active surveillance or extirpative therapy; the more aggressive approach, while definitively treating the tumor, would lead to the treatment of many indolent tumors of uncertain malignant potential [5]. An important concept in the management of renal tumors is cytoreductive therapy. No matter how large or the extent of metastatic disease, there still appears to be benefit to treatment in most situations. The Southwest Oncology Group 8948 and European Organization for Research and Treatment of Cancer (EORTC) 30947 trials demonstrated superiority of nephrectomy + interferon‐α over interferon‐α alone with 3‐ and 10‐month survival benefit [6]. Patient selection is important for optimization of therapeutic benefit and efficacy. Currently, in most settings, ablation patients are typically poor surgical candidates, or those require nephron sparing, usually on the basis of prior resections and/or renal insufficiency. Typical patients have tumors of TNM staging T1a (limited to the kidney and less than 4 cm) [1]. In addition, it is the opinion of the authors that based on the current data, even good surgical candidates with lesions less than 2.5–3 cm in ideal anatomical locations, are also appropriate candidates for cryoablation. Finally, efficacy has also been demonstrated in treating T1b tumors (limited to the kidney and between 4 and 7 cm) but there is no question that based on oncological outcomes, patients in this category, who are likely to tolerate surgery with general anesthesia, should undergo nephrectomy [5]. The first account of the use of cryotherapy to ablate tumors was by James Arnott in the 1800s using combination of ice and salt applied topically to palliate breast and cervical cancer [7, 8]. Topical techniques were further refined in the early 1900s with the use of compressed air, and eventually compressed liquified CO2 to treat dermatologic conditions [9]. By 1967 Ortved et al. published a report of 100 cases using intense cold from liquid nitrogen to ablate tissue from the obstructed prostate gland [10]. Renal cryosurgery followed in 1968 by Lymberopoulos [11]. A shift from liquid nitrogen‐based systems to argon gas‐based ones occurred in the 1970s and entered mainstream use by the 1990s. This enabled a substantial reduction in probe size, allowing for percutaneous applications [7]. While gaining traction in the treatment of liver tumors in the early 1990s, popularity declined soon after, which some have attributed to complications such as cryoshock or tissue “craking.” Cryoshock is believed to be a cytokine‐mediated systemic response to cryoablation that results in hypotension, decreased renal function, coagulopathy, respiratory dysfunction, and multiorgan failure. Although this phenomenon was believed to be relegated to hepatic tumor ablations, usually during open procedures, it was not until the late 1990s that tumor cryoablation, in all organs, saw the resurgence in interest that has resulted in its routine use today. Cryoablation utilizes the Joule–Thomson effect, by which a high‐pressure gas cools as it expands [7]. A closed circuit of high‐pressure argon or nitrogen is passed through a metallic probe, resulting in formation of an iceball around the probe. In contrast, helium warms during expansion, enabling its use in some of the available systems for thawing and to facilitate probe removal [7]. The destruction of tissue is achieved via a number of mechanisms, both direct and indirect, which occur at various phases in the freeze–thaw cycle. During the freeze phase, temperatures rapidly reach −165 °C to −190 °C in the region immediately surrounding the probe. Ice crystals form predominantly in the extracellular space. The resultant osmotic gradient draws water out of the cell, leading to cellular dehydration. The osmotic pressure that develops during freezing due to water flux is sufficient to cause rupture of cellular plasma membrane. As intracellular ice crystals form, protein denaturation damages organelles, leading to cellular death. During the warming phase, ice crystals coalesce into larger crystals, which disrupt the cellular membrane. In addition, laboratory biochemical analyses suggest that decoupling of oxidative phosphorylation during this phase effectively halts the cellular machinery. Warming initially affects extracellular ice, causing a reversal of the osmotic gradient, which causes an influx of water, increasing cellular volume and leading to membrane rupture and cellular necrosis. Histopathologic analysis suggests that lethal intracellular ice crystal formation predominates in the region closest to the probe, while osmotic effects predominate at the periphery of the freezing zone [12]. Indirect effects occur via microvascular occlusion during freezing, with subsequent glomerular congestion and tubular necrosis [12]. During freezing, vasoconstriction and eventual cessation of blood flow to the ablation zone damages the vascular endothelium. During warming, vasodilatation occurs, increasing vascular permeability. Platelet aggregation occurs due to activation by the damaged endothelial lining, triggering microvascular thrombosis, acute ischemia, and hypoxia [12]. These mechanisms result in local ischemia and necrosis of the tumor and surrounding margin. In the long term, scarring and fibrosis have been demonstrated in the ablation zone. Successful tissue ablation requires close monitoring of many factors, including the number of freeze–thaw cycles, rapid cooling, lowest temperature achieved, duration of ablation, and slow thawing. The lethal temperature of normal tissue is −19.4 °C, whereas the fibrous nature of tumor enables it to tolerate lower temperatures. Studies of different tissue and tumor types have shown a range of −20 °C to −40 °C to be ideal for tumor lethality when used with multiple freeze–thaw cycles. The additional benefit of multiple freeze–thaw cycles is attributed to more rapid cooling during successive cycles and increase of the frozen tissue volume. For over 50 years, radical nephrectomy had been the standard treatment for RCCs. As high‐resolution cross‐sectional imaging has gained widespread use, renal tumors are diagnosed at an earlier stage, which some have referred to as a staging migration. This staging migration of renal cancer diagnoses has been accompanied by a shift in the treatment algorithms to include minimally invasive and nephron‐sparing techniques [13]. Guidelines from the American Urological Association (AUA) published in 2009 reflect this shift and recommend partial nephrectomy for solitary renal masses if technically feasible. Radical nephrectomy, ablation, or active surveillance are recommended as alternatives based on tumor characteristics or patient‐specific factors or preference [14]. Huang et al. wrote in 2015 that the number of partial nephrectomies eclipsed radical nephrectomies by 2009 [15]. Although partial nephrectomy currently remains the treatment of choice, ablation has become an attractive alternative in patients with comorbidities. Cryoablation, when compared to robot‐assisted partial nephrectomy, demonstrated somewhat lower 5‐year disease‐free, cancer‐specific, and overall survival rates of 83.1%, 96.4%, and 77.1%, respectively, in the cryoablation cohort versus 100%, 100%, and 91.7% in robot‐assisted partial nephrectomy [16]. Cryoablation offers practical advantages over more invasive treatments. One such advantage over surgery is reduced intraoperative blood loss and postoperative hematoma. This has been attributed to absence of a need for renorrhapy or dissection of the hilum with vascular clamping. Ablation probes can be placed under image guidance in a peripheral manner, thereby avoiding larger vessels. Other complications are also fewer, namely urinary leaks and requirement for ureteral stenting. Adverse effects on renal function following either partial nephrectomy or cryoablation appear to be equally rare [17]. Short hospital stays have also been reported of 72 hours on average for partial nephrectomy compared to the usual practice of cryoablation being performed on an outpatient basis [17]. Finally, cryoablation offers easier localization and access to endophytic and posterior lesions [1]. The most studied minimally invasive ablation technologies are radiofrequency and cryoablation, although newer technologies are gaining traction, such as microwave ablation and irreversible electroporation. Cryoablation differs from these less in its clinical efficacy, and more in practical matters, which affect the overall precision and risk profile of the procedure. One such distinction between cryoablation and other ablative techniques is the relative imaging conspicuity of the iceball; and laboratory studies have confirmed that cryoablation provides superior imaging of the forming iceball with both CT and grayscale ultrasound [12]. Although we understand that the very peripheral aspect of the visualized iceball is nonlethal, the ability to see the applied energy as opposed to just predicting this from the probe geometry, as in other thermal ablation techniques, allows for better confirmation of accurate probe placement and therefore lesion coverage during the freezing process. Another advantage of cryoablation is the reduced anesthesia requirements when compared to heat technologies. A retrospective clinical trial performed by Allaf et al., comparing anesthesia data for both radiofrequency ablation (RFA) and cryoablation in populations with similar demographic and tumor characteristics, showed an overall decreased need for nacrotics in the patients undergoing cryoablation [18]. A much larger retrospective study by Truesdale et al. also noted overall less sedation (midazolam) and narcotic (fentanyl) medications required for cryoablation compared to RFA [19]. This is thought to reflect partial anesthetic effects of cold. Some theorize that the microvascular occlusive effects of ice formation may also mitigate the heat sink effects of ablation of tumors near vessels. Both studies anecdotally mention the requirement for sedation reversal in the RFA groups, which may support a higher level of patient safety and comfort with cryoablation. Ablation procedures are often performed with CT guidance. As mentioned in the Procedure description section, with each pass, the patient is moved in and out of the CT gantry. Vigilance is thus required to ensure that the cables connecting the needles, as well as the gantry itself, do not dislodge or deflect the probes from their intended trajectory. Cryoablation probes are equipped with a stick mode, which lowers the temperature around the probe just enough to provide traction, further securing it within the patient [20].
Percutaneous Cryoablation of Renal Masses
Introduction
Historical perspectives
Physical principles of cryoablation
Direct cellular injury
Indirect effects
Treatment and techniques
Extirpative therapy
Other ablative technologies
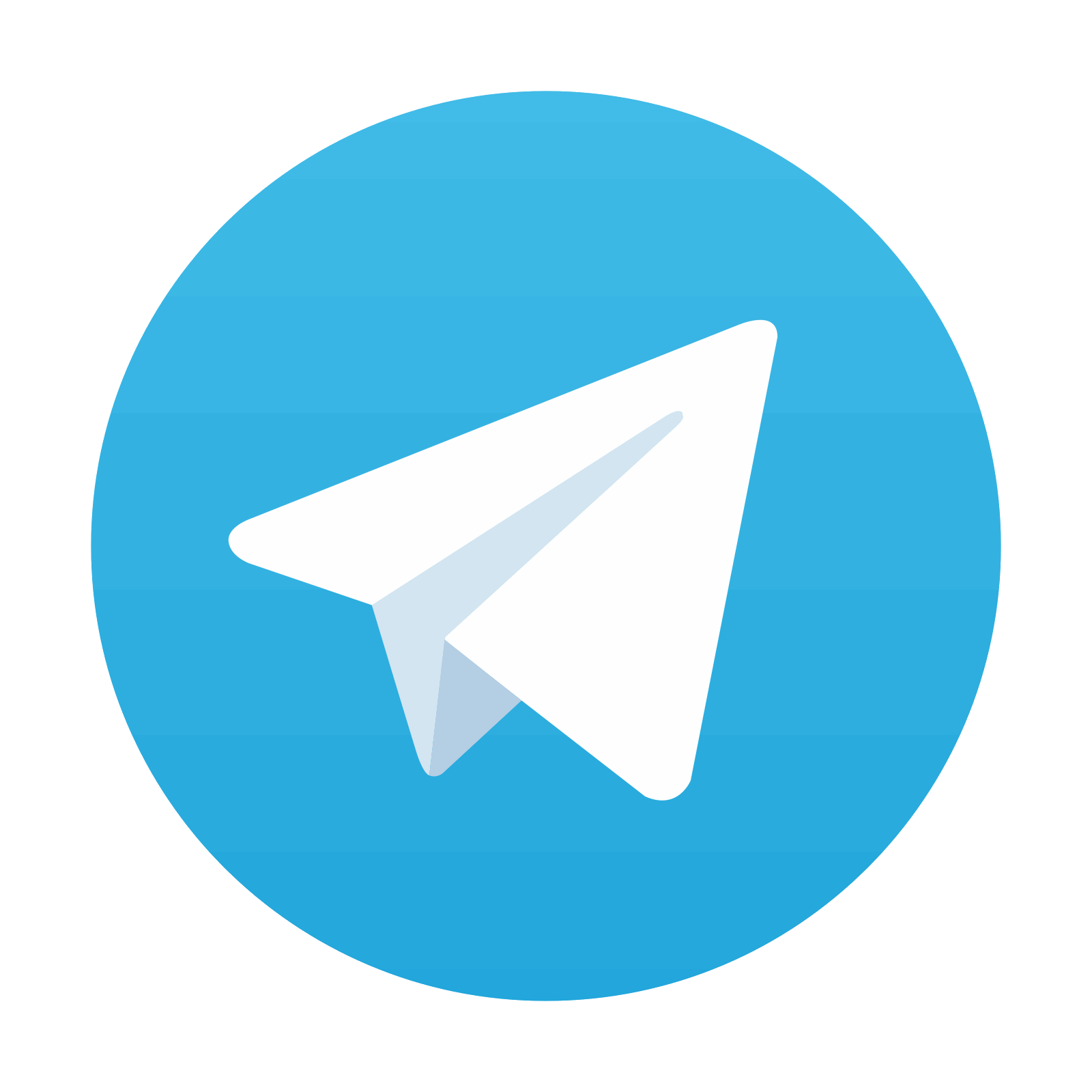
Stay updated, free articles. Join our Telegram channel

Full access? Get Clinical Tree
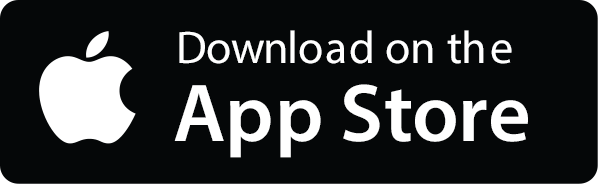
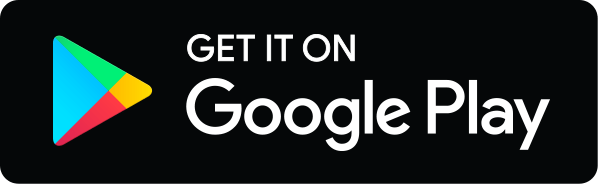