Diagnostic imaging of pediatric urologic disorders is continuously changing as technological advances are made. Although the backbone of pediatric urologic imaging has been ultrasound (US), voiding cystourethrography (VCUG), and radionuclide scintigraphy, newer and advanced modalities are increasingly becoming important. The aim of this review is to discuss the techniques and clinical applications of 3 such imaging modalities as they pertain to pediatric urologic disorders: MR urography, advanced US (harmonic imaging, 3D, voiding urosonography), and CT angiography.
Diagnostic imaging of pediatric urologic disorders is continuously changing as technological advances are made. Although the backbone of pediatric urologic imaging has been ultrasound (US), voiding cystourethrography (VCUG), and radionuclide scintigraphy, newer and advanced modalities are increasingly becoming important. The aim of this review is to discuss the techniques and clinical applications of 3 such imaging modalities as they pertain to pediatric urologic disorders: MR urography (MRU), advanced US (harmonic imaging, 3-dimensional [3D], voiding urosonography [VUS]), and CT angiography (CTA).
Magnetic resonance urography
MRU is a powerful examination that has the distinct advantage of providing both anatomic and functional information in one examination. MRU allows a one-stop-shop evaluation of the renal parenchyma, collecting system, vasculature, bladder, and surrounding structures. MRU has intrinsic high soft tissue contrast resolution and multiplanar 3D reconstruction capabilities, without the use of radiation. Additionally, MRU allows quantification of numerous renal functional parameters including transit times, an index of glomerular filtration rate (GFR), and differential renal functions. To date, MRU may serve as the most comprehensive and definitive study in the evaluation of urinary tract obstruction, complex genitourinary anomalies, and infection. The following sections discuss patient preparation, technique, clinical applications, and limitations of MRU as it pertains to the evaluation of pediatric urologic disorders.
Patient Preparations
Patient preparation is a crucial part of the successful MRU examination. The examination begins with discussion with the family as to the purpose and operations of the study. The patient preparation portion of the MRU examination typically takes approximately 1 hour, which includes sedation, hydration, and catheterization. Patients younger than 7 years are typically sedated to eliminate patient motion artifact. Although sedation protocols must be adapted according to the experience of each center, at our institution, a combination of versed, fentanyl, and phenobarbital is typically used. All children undergoing sedation are under continuous close electrocardiogram (ECG) and pulse oximetry monitoring under the control of an appropriately trained member of the sedation unit. Older nonsedated children are asked to breathe quietly, or if they are able to cooperate, breath hold imaging is performed.
Provided no contraindications (eg, fluid restriction, congestive heart failure) exist, patients are given 20 mL/kg (maximum of 1 L) of normal saline or Ringer’s solution intravenously over the course of 30 to 60 minutes before the start of imaging. To minimize the need for additional manipulations during the scan, the infusion is stopped before entering the MR scanner room. The administration of intravenous (IV) fluid helps to reduce the MR contrast concentration and thus decrease the potential of T2* effect, making a linear relationship of the gadolinium concentration to the signal intensity possible. It also improves the visualization of the pelvicalyceal system and ureter and optimizes the baseline for subsequent furosemide (Lasix) administration.
A bladder catheter without inflatable balloon is placed. In a patient with planned sedation, this is done after sedation. The catheter helps to decompress the bladder. A decompressed bladder is important, as it ensures that the contrast washout is not disturbed by full bladder effect and/or reflux. The catheter may also serve as a urethral marker in cases of possible ureteral ectopy.
The patient is positioned in the MRI scanner supine with the arms above the head. Patients with dilated pelvicalyceal system and without contrast in the ipsilateral ureter need to be turned to the prone position for the acquisition of additional delayed sequences.
Once the patient is appropriately positioned, before the start of imaging, furosemide is administered intravenously with a dose of 1 mg/kg up to a maximum dose of 20 mg. The purpose of the furosemide injection is several-fold. furosemide increases urine flow and ensures the urinary tract is distended without increasing GFR. This increased distension of the urinary tract allows improved visualization of nondilated collecting systems. It serves to reduce the gadolinium concentration for the same reasons as discussed under hydration. Furosemide results in a more uniform distribution of gadolinium-based contrast, which reduces susceptibility artifacts. It is necessary for the evaluation of the excretory function under diuresis. Finally, furosemide administration shortens the examination time. Contraindications to furosemide administration include anuria, electrolyte imbalance, and hypotension. Patients with sulfonamide allergies may also be allergic to furosemide.
The imaging portion of the examination can be expected to take between 30 to 60 minutes, depending on the need of delayed images including after repositioning the patient to the prone position.
Technique
A comprehensive MRU protocol is a 2-part imaging technique composed of precontrast sequences (static MRU) and postcontrast sequences (dynamic or excretory MRU).
Part 1—static MRU (precontrast imaging)
Static MRU uses heavily T2-weighted fluid-sensitive sequences in which urine-containing structures are bright ( Fig. 1 A , B). These T2-weighted images serve to delineate the anatomy of the renal collecting systems and ureters. Unlike the postcontrast excretory MRU images, these T2-weighted images are not dependent on renal function and excretion of contrast material into the collecting systems to outline the anatomy of the urinary tract. Therefore, the T2-weighted sequences prove to be of greatest value in delineating the anatomy of the collecting system of a poorly or nonfunctioning renal moiety or kidney. These sequences become particularly important in identifying ureteral ectopy, for example in the case of the girl with constant wetting. Three-dimensional respiratory triggered T2 sequences can be reconstructed to create maximum intensity projections (MIP) and volume rendered images of the entire collecting system. Additional high-quality axial T2 sequences are also obtained to provide a high-resolution view of the renal parenchyma.
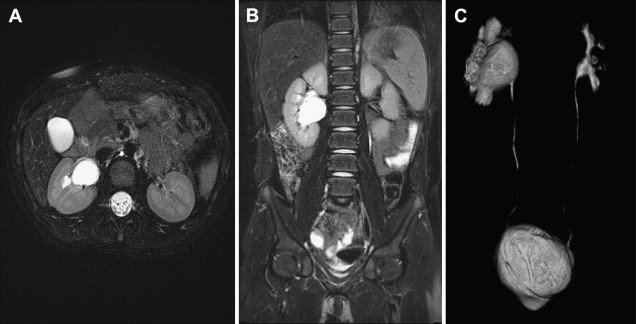
Part 2—dynamic MR urography (postcontrast imaging)
Dynamic MRU not only provides anatomic information including that of the vascular system, but it also offers functional information, which is in many ways analogous to a nuclear medicine study ( Fig. 2 A , B). Intravenous gadolinium is administered and sequential 3D dynamic sequences of the whole urinary tract are acquired. These images can be presented as a MIP and a cine loop. The former provides morphologic information (see Fig. 1 C). The dynamic sequences are the basis of the functional calculation, assess renal perfusion, evaluate renal transit and excretion, and allow generation of signal intensity versus time curves. The images are transferred and postprocessed on an external computer using a freely available custom-made software package ( www.chop-fmru.com ). The software package is used to calculate a number of functional parameters including calyceal and renal transit times, differential renal functions (DRF) based on renal parenchymal volume and Patlak number (an index of GFR), and both parenchymal volume and Patlak number. Moreover, the software package provides time-signal intensity curves of the renal parenchyma and contrasted part of the pelvicalyceal system corresponding to the renal enhancement and excretion (washout) curves, respectively ( Fig. 3 ).
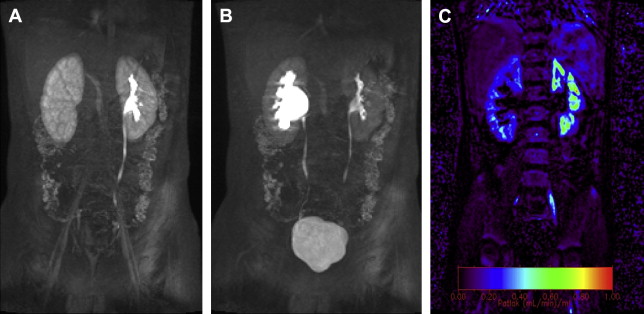
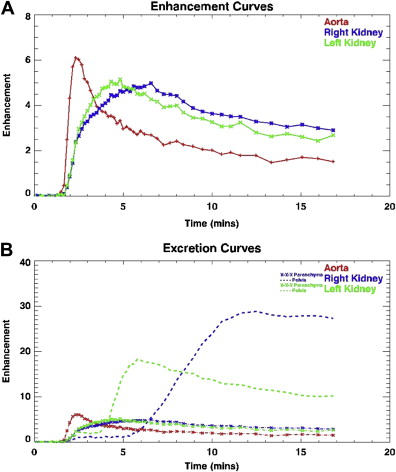
Calyceal transit time (CTT) and renal transit time (RTT) refer to the time period between the appearance of contrast in the aorta and just before its appearance in the calyces and proximal ureter, respectively. CTT is the more reliable parameter, as RTT can vary according to the volume of the renal pelvis and the morphology of uretero-pelvic junction (UPJ); the RTT value alone may not always differentiate between stasis and obstruction. CTT and RTT should be interpreted only in conjunction with both the static and dynamic images. The influence of parenchymal disease on these values should not be underestimated. The cut-off points for normal and abnormal RTT as published in one study do not seem to be universally reliable in classifying UPJ obstruction as compensated and decompensated.
DRF remains the most widely used measure of renal function. With MRU, the differential renal function is calculated using 2 distinct methods. The first method is referred to as the volumetric differential renal function (vDRF) and is based on the volume of enhancing renal parenchyma, which essentially represents functional renal mass. The vDRF is calculated by measuring the volume of enhancing renal parenchyma at the time point of homogeneous renal enhancement before contrast excretion in the calyces as determined by viewing the dynamic series. This method of calculation makes a distinction between functioning enhancing tissue and nonfunctional dysplastic or scarred tissue. This method also allows for the calculation of the individual contributions from upper and lower pole moieties in duplex kidneys. The second method is referred to as the Patlak differential renal function (pDRF), and is an index of GFR. The pDRF is calculated using the Patlak model. The same enhancing parenchyma that determines vDRF is the basis for calculating pDRF, but takes the contrast concentration over time in the aorta into consideration. After the injection of gadolinium, sequential intensity values of the aorta and renal parenchyma are obtained and subsequently entered automatically in the Patlak equation; this generates the Patlak plot and Patlak numbers. The vDRF is a relatively stable number, whereas the pDRF tends to change with acute changes in GFR. This change in pDRF may reflect a measure of renal function recoverability. Comparatively speaking, vDRF is thought to correlate with the DRF measured by dimercaptosuccinic acid (DMSA) scintigraphy, whereas pDRF is thought to correlate with mercaptotriglycylglycine (MAG3) scintigraphy. When the percentage of the renal parenchyma is taken into consideration with the pDRF, a volumetric pDRF (vpDRF) can be calculated.
Comparison with Renal Scintigraphy
MRU has been shown to be superior to renal scintigraphy in many regards. MRU has superior contrast and temporal and spatial resolution, and can provide precise anatomic information, which radionuclide studies cannot. MRU has also been shown to be superior to renal scintigraphy in distinguishing between pyelonephritis and scar. The functional analysis of MRU also provides more comprehensive functional information.
Limitations
Although MRU is a powerful study with numerous advantages, few limitations do exist. MRU can have relatively long imaging times and is sensitive to motion artifact, which necessitates sedation for young children. This may be considered a minor limitation by some, as MRU can provide both anatomic and functional information in one examination.
Although, in an effort to avoid ionizing radiation, MRU may be used to follow children with bigger recurrent stone disease, CT remains the gold standard for the detection of small urinary tract calculi that are not always necessarily resolvable on MR.
The postcontrast dynamic images and interpretation of time-signal intensity curves can be used to assess for the possibility of vesicoureteral reflux. However, MR VCUG is unlikely to replace conventional VCUG because of technical limitations including the inability of sedated patients to completely empty their bladder.
An important limitation of the dynamic postcontrast part of the MRU is that it can be contraindicated in patients with moderate renal insufficiency. As stated, excretory MRU involves the administration of intravenous gadolinium and requires excretion into the collecting systems. Although it was previously thought that gadolinium could be safely administered in patients with renal insufficiency/failure in an effort to avoid iodinated contrast material, relatively recent reports have linked the disorder nephrogenic systemic fibrosis (NSF) to gadolinium administration. New recommendations are to make every effort to avoid administering gadolinium-based contrast material in patients with moderate to severe renal insufficiency (GFR <30 mL/min). However, this issue remains a topic of investigation and recommendations may change in the future.
Clinical Applications
MRU can be used in the evaluation of congenital genitourinary (GU) anomalies, urinary tract obstruction, infection versus scarring, renal transplantation, hematuria, and surgically altered anatomy. The 2 most common indications for MRU in the pediatric population include complex congenital anatomic anomalies of the GU tract and hydronephrosis.
Congenital GU Anomalies
Congenital anomalies of the GU tract are more common than those of any other organ system, with a frequency of between 1:650 and 1:1000. Urinary tract anomalies predispose the child to a variety of complications, including infection, obstruction, wetting, stasis, and stone disease. At present, US is still most often used to provide anatomic information and renal scintigraphy to obtain functional information. MRU has the potential to replace multimodality imaging by providing both the 3D anatomic evaluation of the entire urinary tract and the renal functional information that will aid in management decisions. For example, in the evaluation of the girl who is “wet all the time,” MRU has been shown to be superior to US both in the detection of occult upper pole moieties and in demonstrating ureteral ectopy. In this instance, excretory function of the kidney is not a limiting factor in diagnosing an ectopic ureter because the static-fluid MRU images are sufficient. This is also true for the detection of dysplastic ectopic kidneys that are very difficult to see with other imaging modalities. MRU can also clarify the anatomy and complications related to complex urinary tract anomalies, such as crossed fused renal ectopia and horseshoe kidney.
Hydronephrosis/Obstruction
Hydronephrosis is the most common abnormality seen in the kidney on both prenatal and postnatal imaging. UPJ obstruction is the most common cause of hydronephrosis in childhood. In neonates, UPJ obstruction is most often attributable to intrinsic narrowing of the proximal ureter; however, in older children it is often attributable to a crossing vessel causing extrinsic compression and kinking of the ureter. Prognosis and need for surgical intervention is best predicted on the basis of renal function.
MRU can provide both the anatomic and functional information necessary to guide management. Obstruction is suggested morphologically by dilation of the renal pelvis and narrowing of the ureter (see Fig. 2 A, B). MRU can depict the site of ureteral narrowing, assess for a possible crossing vessel, and evaluate for possible obstructing urothelial mass. Obstruction is suggested functionally by non- or delayed excretion of contrast material.
Dynamic MRU can also be used to assess the success of pyeloplasty. Prepyeloplasty parameters, vDRF, pDRF, and RTT can be compared with those obtained postpyeloplasty to ascertain the effect of surgery.
Pyelonephritis Versus Scar
MRU can demonstrate pyelonephritis but may also distinguish acute pyelonephritis from renal scarring, which is a distinct advantage compared with renal scintigraphy. MRU has also been shown to be more sensitive in detecting renal scarring when compared with US, a finding of clinical importance when deciding if a Deflux procedure should be performed. The normal renal cortex demonstrates homogeneous low signal intensity on T2-weighted images. Acute pyelonephritis will appear as areas of abnormal T2 signal hyperintensity in the renal cortex and will also demonstrate a striated or patchy nephrogram on postcontrast imaging similar to that seen with CT. Unlike acute pyelonephritis, renal scarring will appear as focal area(s) of parenchymal volume loss with deformity of the renal contour, with or without associated deformity of the underlying calyx.
Renal Transplantation
Renal transplant complications can be categorized as prerenal (vascular), renal (intrinsic parenchymal disease), and postrenal (obstruction). MRU has the ability to evaluate and distinguish among these complications in a single comprehensive examination. Because gadolinium is being administered for the dynamic portion of the MRU, MR angiography (MRA) can also be performed during the first pass of contrast material through the aorta and renal arteries. As such, evaluation for possible renal artery stenosis can be made. Additionally, delayed images will allow assessment for patency of the renal veins. Intrinsic parenchymal disease can be suggested on the T2-weighted images if increased cortical T2 signal and loss of corticomedullary differentiation are seen. The T2-weighted sequences can also be used to depict hydronephrosis, ureteral stenosis/kinks, lymphoceles, and urinomas. The dynamic MRU can provide functional information of the transplant kidney such as GFR. Decreased transplant function may provide an earlier clue to acute or chronic rejection and potentially reduce the need for biopsy.
Hematuria
MR urography can evaluate for possible renal parenchymal lesions, vascular lesions, and urothelial abnormalities as an etiology for hematuria. Additionally, MRU can be used to follow children with large recurrent urinary tract calculi in an effort to avoid ionizing radiation. Urinary tract calculi will appear as filling defects in the collecting systems on both the static-fluid urography images and excretory MRU images. Secondary signs of stone disease such as perirenal edema, renal enlargement, and urothelial thickening can be demonstrated.
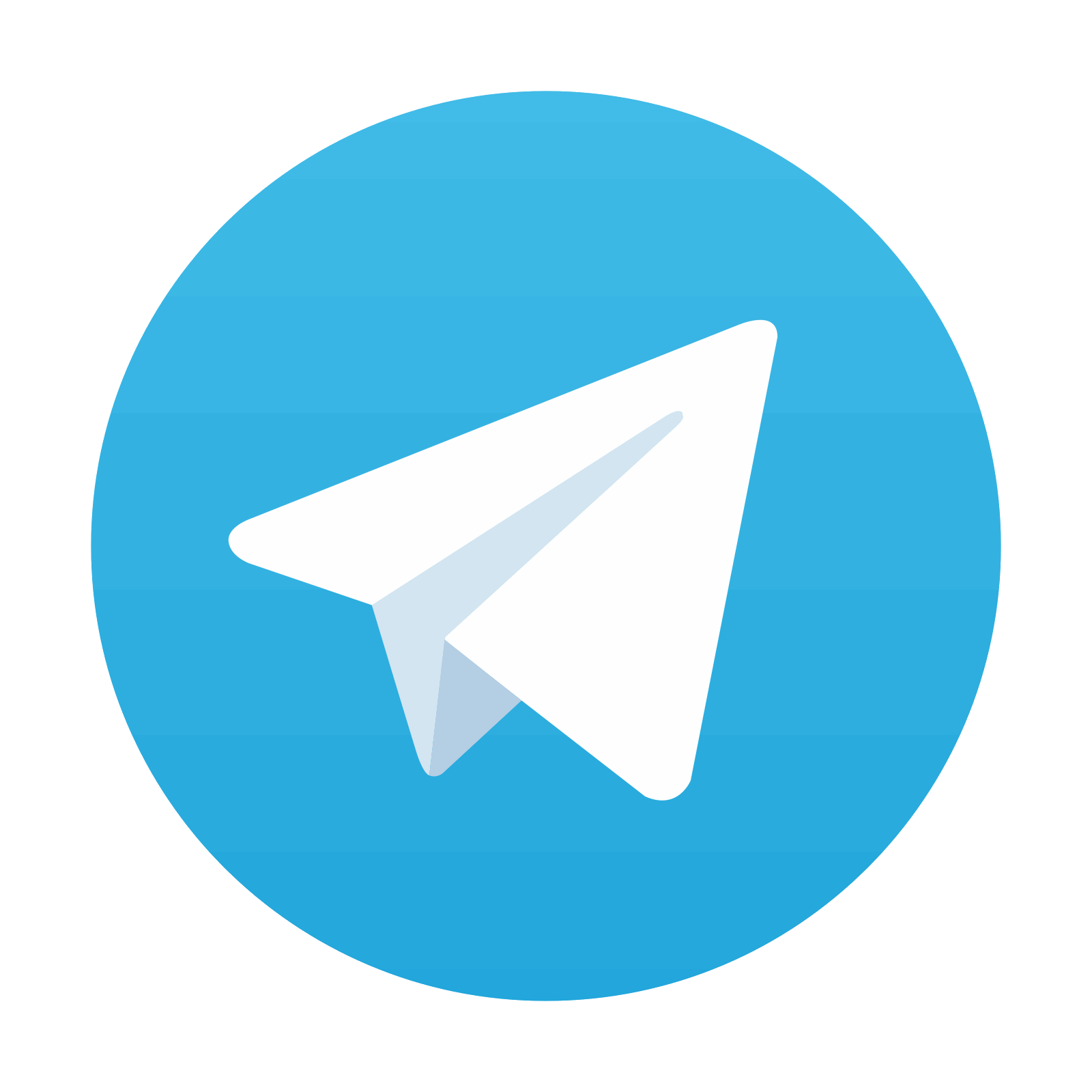
Stay updated, free articles. Join our Telegram channel

Full access? Get Clinical Tree
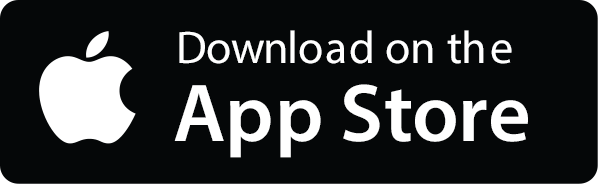
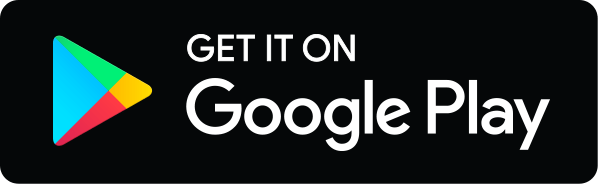