Cytokine
Source
Role in fibrogenesis
TGF-β1
Main source is HSCs (autocrine loop). Other source: KCs, Liver sinusoidal endothelial cells, and hepatocytes [32]
Smad3 signaling to activate HSCs [33]
Expression of the matrix-producing genes
Promoting TIMP
Downregulates MMPs
Induces apoptosis of hepatocytes [34]
TNF-α
Main source is macrophage and monocyte; other sources HSCs, and KCs [35]
Induces synthesis of extracellular matrix by HSCs
Inhibits HSCs apoptosis (downregulation of p53) [36]
PDGF (mostly PDGF-B and PDGF-D)
KCs [6]
Proliferation of HSCs (through mitogen-activated protein kinase (MAPK) and protein kinase (PK)B/Akt pathways [37]
Interferon
Leukocytes [6]
IFN-α: antiapoptotic effect on activated HSCs [38]
Inhibiting HSC activation through TGFβ1 pathways
Proapoptotic effect on HSCs by downregulating heat-shock protein 70
Decreases production of α-smooth muscle actin and collagen
Profibrogenic IL
T lymphocytes, KC, in addition to endothelial cells
IL1: HSCs activation and stimulate production of MMP and TIMP-1 [40]
IL-17: particularly in hepatitis B, upregulation of TNF-α, TGF-β1, and collagen 1α [41]
Leptin
Adipose cells
Upregulates collagen expression in HSCs [42]
In addition to cytokines, there has been a growing interest in the role of micro RNA (miRNA), which are noncoding RNA segments that act as posttranscriptional regulators of many other genes. The miRNA can be divided into profibrogenic and antifibrogenic [6] (Table 1.2). Both animal and human studies show a significant role in hepatic fibrogenesis and a potential target for therapy.
Table 1.2
Profibrogenic and antifibrogenic miRNAs
Profibrogenic miRNA | Antifibrogenic miRNAs |
---|---|
miR-214-5p: increase expression of fibrosis-related genes (such as MMP-2, MMP-9, α-SMA, and TGF-β1) [43] miR-181b and miR-221/222 : promote HSC proliferation by regulating p27 gene and the cell cycle [44, 45] miR-155: increase TNF-α production in response to gut-derived lipopolysaccharide in alcoholic hepatitis [46, 47] In addition to others that are upregulated in response to TGF-β as miR-571 [48] | miRNA-150 and miRNA-194: inhibit HSC activation through downregulation of c-myb [49] miR-21: inhibits HSC activation through downregulation of TGF-β expression [50] miR- 133a: decreases expression of collagens and is inhibited by TGF-β in the setting of chronic hepatitis [51] |
As the deposition of excess collagen occurs, matrix degradation ensues through MMP. However, in the case of chronic liver injury, matrix production occurs at a higher rate than degradation resulting in turn over, matrix remodeling, and formation of the acellular dense collagen rich matrix [52]. Moreover, there is a process of matrix stabilization, leading to accumulation of elastic fibers and covalent cross-linking of collagen. This progression enables the matrix to be more resistant to enzymatic degradation and is driven by enzymes such as lysyl oxidase, a potential target for inhibition of fibrogenesis [53].
Outcome of Activated Stellate Cells
Changes in activated HSCs, for example, increase the expression of nerve growth factor receptor (NGFR) and make them more susceptible to apoptosis [54]. However, even with such changes, there are other factors associated with ongoing liver tissue injury that inhibit HSC apoptosis and the net result is prolonged life of activated cells. This is mediated by factors, such as the antiapoptotic activity of TIMP-1; which was shown to inhibit MMP-2 activity, and blocks apoptosis [55]. Other agents as TNF-α and IGF-I inhibit HSC apoptosis through interaction with NF-κB and phosphatidylinositol-3 kinase (PI3-K) pathways, respectively [54, 56]. Also as mentioned earlier, some components of the fibrotic matrix (i.e., collagen VI) can stimulate activated HSC survival [57]. Resolution of injury is associated with stimulation of apoptosis that was inhibited by the abovementioned mechanisms. Reversal of HSCs to quiescent form occurs after resolution of inflammation and contributes to decreased number of activated cells [58]. In the case of unresolved liver injury, for example, with untreated chronic hepatitis C infection, HSC activation continues, along with active fibrogenesis, eventually resulting in liver cirrhosis.
Macrophages play a critical regulatory role in wound healing and in the resolution of fibrogenesis. They can stimulate liver regeneration and scar resolution through production of fibrinolytic agents such as MMP 13 [59]. Found within areas of fibrosis, scar-associated macrophages (SAMs) have been identified as potential targets of therapy to reverse fibrosis [60, 61].
Definition and Classification of Portal Hypertension
Portal hypertension is defined as the rise in portal pressure above the normal hepatic venous pressure of 1–5 mmHg. The key physiologic feature of portal hypertension is an increase in resistance to portal blood flow. Portal hypertension can be broadly classified into three types: (1) prehepatic, (2) intrahepatic, (3) posthepatic depending on the site of where the resistance develops. Prehepatic portal hypertension involves any obstruction along the portal vein. Intrahepatic portal hypertension in turn can be further subclassified into three subcategories, including (i) presinusoidal (e.g., schistosomiasis, granuloma, congenital fibrosis), (ii) sinusoidal (e.g., cirrhosis), and (iii) postsinusoidal (e.g., sinusoidal obstruction syndrome). In turn, posthepatic portal hypertension involves inferior vena cava obstruction (e.g., Budd–Chiari syndrome) or heart disease (e.g., constrictive pericarditis) [62]. The underlying pathophysiology and the consequences of portal hypertension vary according to the etiology and the location of increased resistance. The focus of this section is portal hypertension at the level of the hepatic sinusoids in the setting of end-stage liver disease [63].
Intrahepatic Changes
Increased Intrahepatic Resistance
Multiple factors contribute to the increase in vascular resistance within the liver. Decreased intrahepatic vasodilators (nitric oxide, NO) and an increase in vasoconstrictors (cyclooxygenase-1, COX-1) appear to be the main drivers of intrahepatic vasoconstriction. Decrease in NO results from a combination of lower concentrations of the NO producing enzyme, endothelial synthase, as well as, NO depletion via formation of peroxynitrite in the setting of chronic bacterial endotoxemia [64]. This increase in vasoconstriction occurs as a result of increased activity of COX-1, which in turn causes an upregulation of thromboxane A2 production, leading to intrahepatic vasoconstriction. Other additional upregulated intrahepatic vasoconstrictors, include endothelin-1, angiotensin-II, and norepinephrine [65]. To a lesser extent, activated stellate cells, which are present in the perisinusoidal space, also play an important role in intrahepatic resistance, as a result of endothelin-1 stimulation [66].
Intrahepatic Angiogenesis
Increased numbers of blood vessels are observed in the cirrhotic fibrotic septa and in the surrounding regenerative nodules. Activated stellate cells stimulate endothelial cells through the release of certain factors, such as, vascular endothelial growth factor (VEGF) and angiopoietin [67, 68]. In theory, the formation of these new blood vessels is expected to decrease hepatic vascular resistance. However, the opposite occurs because these new vessels are abnormal, leading to irregular flow patterns, known as splitting (or intussusceptive) angiogenesis [69].
Intrahepatic Microthrombosis
Part of the hypercoagulable state associated with cirrhosis is the formation of microthrombi in intrahepatic vasculature. These microthrombi propagate in the progression of fibrosis and the increase in intrahepatic vascular resistance contributing to portal hypertension [70]. This can be seen when examining the histology of liver explants. Obliterative lesions and intimal fibrosis – suggestive of healed microthrombi – were present in hepatic and portal venules and associated with regions of confluent fibrosis and cirrhotic nodules (focal parenchymal extinction theory) [71]. This may have clinical application shown through significantly delayed decompensation in cirrhotic patients treated with a 12-month course of enoxaparin [72]. In summary, increased intrahepatic resistance occurs secondary to both structural and dynamic changes. Structural changes in the cirrhotic nodule can be seen by the presence of impaired blood flow, fibrous septa, angiogenesis, and microthrombi. Dynamic changes occur secondary to intrahepatic cytokine-induced vasoconstriction and stellate cell contraction.
Extrahepatic Changes
Extrahepatic Arteriolar Vasodilation
In the setting of cirrhosis, vasodilation is noted in both splanchnic and systemic circulations that significantly contribute to the development of portal hypertension. Similar to the intrahepatic environment, NO is the most potent vasodilator molecule. An increase in portal pressure when sensed by endothelial cells leads to stimulation of endothelial NO synthase, resulting in an increase NO production [73]. Other vasodilator molecules that have been identified to participate in extrahepatic arteriolar vasodilation, include carbon monoxide, prostacyclin, and endocannabinoids [65]. In addition to increased levels of potent vasodilator molecules, there is also a decreased production of potent vasoconstrictor molecules, such as bradykinin [65].
Aside from the disequilibrium between said vasodilators and vasoconstrictors, structural changes such as (1) decreased vascular sympathetic tone as a result of atrophy of sympathetic nerve [74] and (2) thinning of mesenteric arteries may also contribute to splanchnic vasodilation [75].
The net result of splanchnic and systemic vasodilation is the relative decrease in the effective blood volume and systemic vascular filling. This eventually causes stimulation of compensatory mechanism, such as release of antidiuretic hormone and activation of the renin–angiotensin–aldosterone system. This, in turn, leads to both sodium and water retention and increase in blood volume. In accordance to Ohm’s law, such a compensatory mechanism leads to an increase in portal pressure that is proportionate to both flow resistance and portal inflow [76]. The latter is increased by both splanchnic vasodilation and the increased blood volume seen mentioned earlier (Fig. 1.1).


Fig. 1.1
Pathogenesis of portal hypertension (NO nitric oxide, RAS renin–angiotensin system, ADH antidiuretic hormone)
Splenomegaly and Increased Spleen Stiffness
Splenomegaly is a common sign of portal hypertension. It initially occurs as a result of congestion of the spleen’s red pulp in the setting of decreased low venous drainage from the spleen into the now higher portal pressure system. However, as the spleen enlarges its elasticity decreases and stiffness increases, thus creating higher resistance in the portal system [79]. Additionally, the sheer blood volume acquired reservoirs in the now larger spleen, leading to an overall increase in portal blood flow. Given that the portal system is a fixed circuit, the increasing amount of volume overwhelms the system leading to drainage through alternate routes (e.g., varices) [80].
Role of Bacterial Translocation
In cirrhosis there is an increase in bacterial translocation and lipopolysaccharides endotoxemia, as a result of increased (1) intestinal bacterial overgrowth, (2) increased intestinal permeability [81, 82], (3) decreased endotoxin clearance by malfunctioning Kupffer cells and hepatocytes, as well as, low circulating albumin, which has an endotoxin-binding effect [83]. Endotoxins also cause increases in NO production, hepatocyte inflammation via activation of tumor necrosis factor-α (TNF-α) [84], and production of intrahepatic vasoconstrictors, endothelin-1, and thromboxane A2 [85].
Role of Portal Hypertension in Development of Common Complications of Cirrhosis (Varices, Ascites, and HRS)
Esophageal Varices
The presence of esophageal varices represent an elevated portal pressure gradient. Dilated submucosal esophageal veins enlarge when the periesophageal tributary drain through become incompetent communicating veins. As the portal pressure increases, more blood is diverted into the submucosa in an attempt to decompress the portal circulation. The loose submucosal connective tissue and the negative intra thoracic pressure allow further expansion and increase in size of the varices [86, 87]. Increased neovascularization in splanchnic organs plays a role in formation of portal collaterals including varices, shown in animal studies to be mediated by VEGF. When VGEF and PDGF are inhibited, there is a reduction of collateral vein formation [88, 89].
One of the most serious complications of cirrhosis is when esophageal wall tension is exceeded and rupture of the varices occurs leading to bleeding. This increased tension is best described by Laplace’s law [Q x (nl/ ∏ r 4)] x r/w, where Q refers to blood flow per unit of time, r and l are radius and length of the blood vessel, and w is wall thickness. The longer and larger the varices with higher flow rate within a thin wall the more likely for spontaneous rupture and bleeding to occur [90].
Gastric and Ectopic Varices
Gastric varices develop via the same principle and are identified based on their anatomical relationship to esophageal varices and location in the stomach. Gastroesophageal varices when in continuity with the esophagus are classified into two types: type 1 (GOV 1) found along the lesser curvature, whereas type 2 (GOV 2) run along the greater curvature toward the fundus of the stomach. Larger varices are commonly found in GOV 1 than in GOV 2. This is likely due to their relationship to the left gastric vein [91].
Isolated gastric varices do not communicate with the esophagus and are also classified into two types: type 1 (IGV 1) are seen as a cluster of isolated varices in the fundus where type 2 (IGV 2) are isolated varices seen in other parts of the stomach. Splenic vein thrombosis is often associated with presence of IGV1 where portal vein thrombosis is associated with IGV2 [92].
Other ectopic varices can be seen at sites of previous surgery where there is a cross relationship between portal circulation (gastrointestinal tract) and systemic circulation (abdominal wall). Patients with portal hypertension undergoing bowel surgery and stoma formation are susceptible to peristomal varice formation [93].
Portal Hypertensive Gastropathy
The presence of portal hypertensive gastropathy (PHG) is a highly prevalent complication of portal hypertension which manifests as chronic versus overt blood loss. The severity correlates with the progression of liver disease and presence of other signs of portal hypertension such as large esophageal varices [94]. How PHG develops is not fully understood; however, available evidence suggests that portal hypertension increases congestion of capillaries and venules in the gastric submucosa [95], leading to mucosal microcirculation abnormalities causing hypoxia and dysregulation of local cytokines which impair healing and increase risk of bleeding [96]. Patients who suffer from PHG-associated chronic blood loss may requiring repeat blood transfusion and thus considered for decompression with shunt surgery or transjugular intrahepatic portosystemic shunt [97].
It is clinically important to understand how the pathogenesis of PHG differs from gastric antral vascular ectasia (GAVE-watermelon stomach). GAVE is not directly related to the degree of portal hypertension and may be seen in other disease processes like chronic gastritis. Patients with GAVE have an increased concentration of locally acting vasodilator substances (mainly gastrin and prostaglandin E) along with altered antral motility, hence, the histologic appearance of mucosal capillary dilation along with fibrin thrombi and fibromuscular hyperplasia [98]. As the pathophysiology implies, GAVE does not respond to reduction of portal pressure with shunting or beta-blocker therapy [99].
Ascites, Refractory Ascites and Hepatorenal Syndrome
The most acceptable theory currently on how cirrhotic ascites develops is the forward theory [100]. The presence of sinusoidal portal hypertension results in splanchnic arterial vasodilation leading to a forward increase in filtration across splanchnic capillaries and lymphatics. As decompensation worsens, filtration increases beyond the capability of the lymphatic system to complement return to the circulation [101] This effective intravascular reduction activates the renin–angiotensin–aldosterone system stimulating sodium and water retention in an attempt to compensate for underfilling by increasing blood volume. However, because of the increased filtration and the low plasma oncotic pressure, continuous leakage into the peritoneal cavity occurs leading to more ascites [102]. In more advanced cirrhosis, renal vasoconstriction develops along with enhanced sodium reabsorption in the renal tubule. This leads to very low urinary excretion of sodium and development of refractory ascites and eventually hepatorenal syndrome [103].
Diagnosis and Measurement of Portal Hypertension
The diagnosis of portal hypertension is portrayed in patients who have a history of cirrhosis with clinical signs of ascites, varices, or low platelet count. Imaging findings suggestive of portal hypertension are not sensitive or specific to the diagnosis [104]. The presence of splenomegaly, dilated portal vein with reversed flow, recanulized umbilical vein or other intra-abdominal collaterals is relative markers for but do not quantify the degree of portal hypertension.
The measuring of portal pressure is indicated to confirm diagnosis and help determine etiology and/or stratify the risk of complications. Hepatic venous pressure gradient (HVPG) is the method preferred to measure portal hypertension. Performed by interventional radiology, a 6 or 7 French balloon catheter is inserted through the right internal jugular vein and then placed into the hepatic vein to measure the free hepatic pressure. HVPG is an approximate measure of the gradient between the systemic circulation (represented by the free hepatic pressure-FHVP) and portal pressure (represented by the wedge hepatic pressure-WHVP), HVPG = WHVP − FHVP. The wedge hepatic pressure is measured after inflating the balloon. A difference of 6 mmHg or more is consistent with portal hypertension with a gradient > 10–12 mmHg required for clinically significant portal hypertension and development of complications such as ascites and varices [105].
It is important to note that although there is direct measurement of hepatic vein pressure, the portal vein pressure is an indirect method, as wedge hepatic venous pressure is performed with the catheter still in the end tributary of the hepatic vein. This is a limitation of this technique in cases of postsinusoidal induced portal hypertension, as the gradient will remain normal and will not reflect the actual portal hypertension. An alternative method that is more accurate but more invasive is to directly pass the catheter to the portal venous system through a transhepatic catheter. A new technique using the endoscopic guidance of ultrasound (EUS) has been described in animal models with adequate correlation with the conventional interventional radiology method [106].
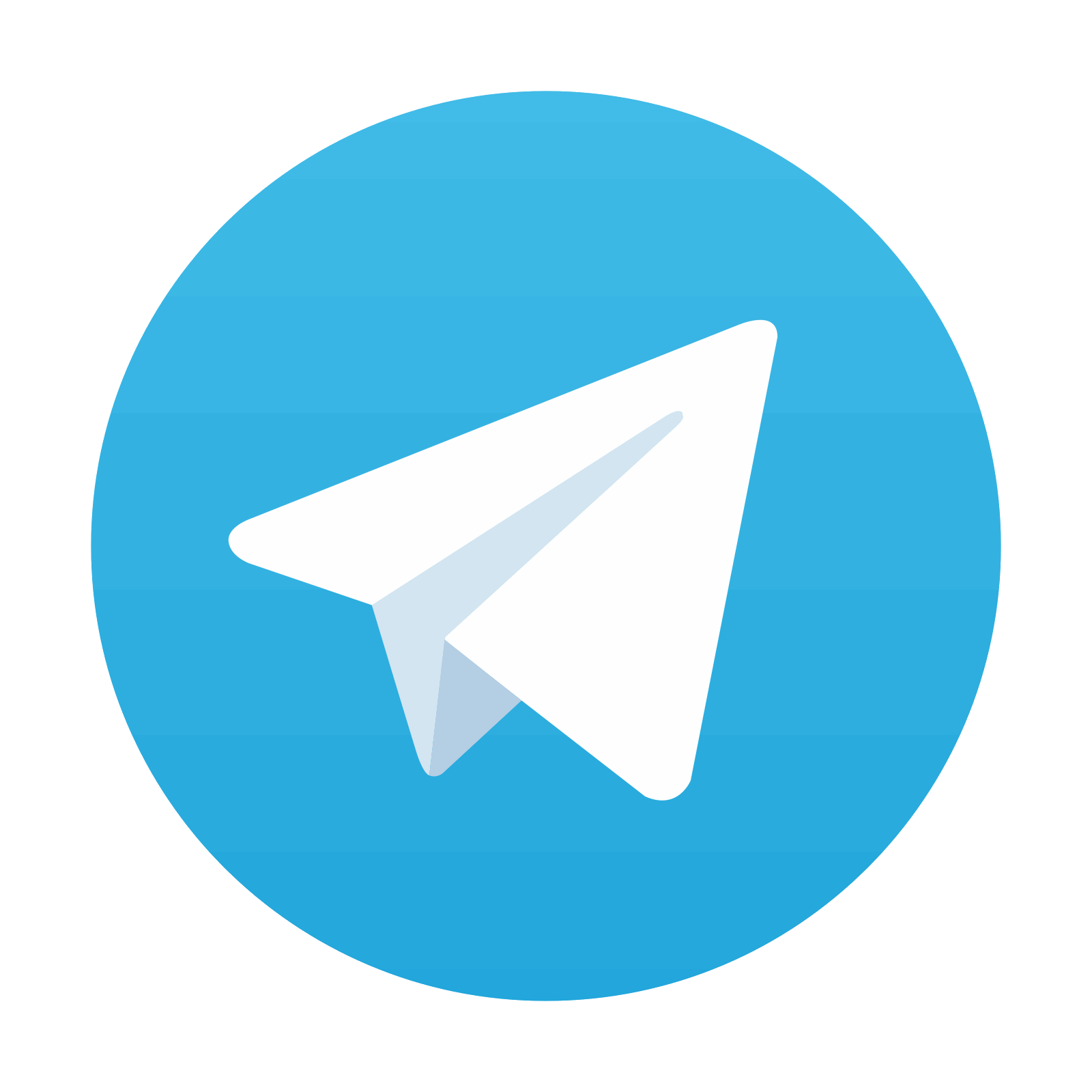
Stay updated, free articles. Join our Telegram channel

Full access? Get Clinical Tree
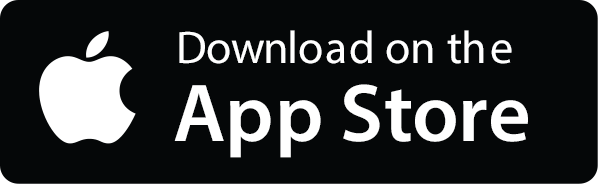
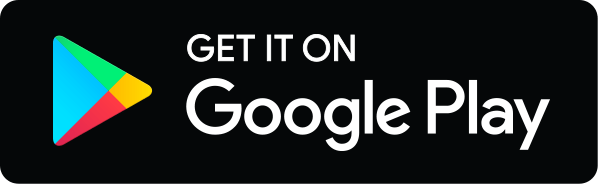