(1)
Division of Vascular Surgery, Stanford University School of Medicine, Stanford, CA, USA
Introduction
Mechanisms of abdominal aortic aneurysm degeneration have been the subject of intense investigation over the last 20 years. As noted in Fig. 4.1, the pathogenesis of abdominal aortic aneurysm disease involves a symphony of interactions between genetic risk, environmental exposures, and interplay between aortic mural inflammation, angiogenesis, smooth muscle cell and elastin depletion, wall strain, and dysfunctional and insufficient regenerative responses of the extracellular matrix [1–10] (Table 4.1).


Fig. 4.1
In the setting of specific at-risk haplotypes (such as variability at chromosome 9, p21) and demographic and environmental risks, the interplay of unfavorable hemodynamic influences on expression of reactive oxygen species, proteolytic enzymes, pro-inflammatory immune responses, and mediator production creates conditions for aneurysmal degeneration of the infrarenal aorta, over the course of years to decades (Reproduced from Tedesco and Dalman [91], with permission from Elsevier)
Table 4.1
Results of multivariable regression analysis for predictors of abdominal aortic aneurysm
Variable | Odds ratio | 95 % confidence interval | P values |
---|---|---|---|
Male (vs. female) | 5.71 | 5.57–5.85 | <.0001 |
Age (vs. <55) | |||
55–59 | 2.76 | 2.55–3 | <.0001 |
60–64 | 5.35 | 4.97–5.76 | <.0001 |
65–69 | 9.41 | 8.76–10.12 | <.0001 |
70–74 | 14.46 | 13.45–15.55 | <.0001 |
75–79 | 20.43 | 18.99–21.99 | <.0001 |
80–84 | 28.37 | 26.31–30.59 | <.0001 |
Race/ethnicity (vs. Caucasian) | |||
Hispanic | 0.69 | 0.62–0.77 | <.0001 |
African American | 0.72 | 0.66–0.78 | <.0001 |
Asian | 0.72 | 0.59–0.75 | <.0001 |
High blood pressure | 1.25 | 1.21–1.28 | <.0001 |
Coronary artery disease | 1.72 | 1.69–1.76 | <.0001 |
Family history of AAA | 3.8 | 3.66–3.95 | <.0001 |
High cholesterol | 1.34 | 1.31–1.37 | <.0001 |
Diabetes | 0.75 | 0.73–0.77 | <.0001 |
Peripheral arterial disease | 1.59 | 1.54–1.65 | <.0001 |
Carotid disease | 1.51 | 1.46–1.56 | <.0001 |
Cerebrovascular history | 1.18 | 1.14–1.21 | <.0001 |
Smoking, packs/day | |||
≤10 years | |||
<0.5 | 2.61 | 2.47–2.74 | <.0001 |
0.5–1 | 3.19 | 2.93–3.46 | <.0001 |
>1 | 3.2 | 2.88–3.56 | <.0001 |
11–20 years | |||
<0.5 | 4.87 | 4.63–5.12 | <.0001 |
0.5–1 | 5.79 | 5.48–6.12 | <.0001 |
>1 | 6 | 5.66–6.35 | <.0001 |
21–35 years | |||
<0.5 | 7.29 | 6.97–7.64 | <.0001 |
0.5–1 | 7.99 | 7.62–8.38 | <.0001 |
>1 | 8.41 | 8.57–9.36 | <.0001 |
>35 years | |||
<0.5 | 8.96 | 8.57–9.36 | <.0001 |
0.5–1 | 11.19 | 10.76–11.64 | <.0001 |
>1 | 12.13 | 11.66–12.61 | <.0001 |
Quit smoking | |||
<5 years ago | 0.87 | 0.84–0.912 | <.0001 |
5–10 years ago | 0.68 | 0.65–0.71 | <.0001 |
>10 years ago | 0.42 | 0.41–0.43 | <.0001 |
Fruit and veg. >3 times/week | 0.91 | 0.88–0.92 | <.0001 |
Nuts >3 times/week | 0.9 | 0.89–0.93 | <.0001 |
Exercise ≥1 time/week | 0.86 | 0.85–0.88 | <.0001 |
BMI ≥ 25 kg/m2 | 1.2 | 1.17–1.22 | <.0001 |
Epidemiologic studies provide an accurate accounting for demographic and environmental risks. Male gender, age, family history, high cholesterol, hypertension and other cardiovascular diseases, increasing years of smoking and number of cigarettes smoked, and excess weight all carried increased risk for abdominal aortic aneurysm (AAA) disease. The presence of diabetes, smoking cessation, modest levels of regular exercise, consumption of nuts, vegetables, and fruits, as well as African American, Hispanic, or Asians descent are all negatively associated with AAA risk [11].
Much less is known, however, regarding the process(es) that promote aneurysmal progression or rupture in existing “atherosclerotic” aneurysms. As noted in Fig. 4.2, 25-year follow-up data from the Chichester screening study in the UK demonstrates that AAA identified at screening followed a bimodal distribution in terms of aortic diameter enlargement in the years following the baseline imaging study. At both the 5–10-year time intervals, a significant percentage of AAA was noted to either enlarge or remain stable over time. The cellular and molecular mechanisms accounting for these different natural histories, subjects of intense investigation over the last few decades, remain obscure.


Fig. 4.2
Distribution of initial abdominal aortic aneurysm (AAA) diameters and final diameters at (a) 3.5 years and (b) 5 years after identification at screening in the Chichester registry. Data shown for the entire cohort and for subjects grouped according to outcome. At both timepoints, only a subset of identified AAAs continue to progress (Reproduced from Thompson et al. [16], with permission from British Journal of Surgery Society)
The term “atherosclerotic” aneurysm is used to distinguish these AAAs from those associated with syndromic (Marfan, Ehlers-Danlos, etc.) or mycotic aortic conditions and recognizes the common risk factors that predispose patients to occlusive or aneurysmal aortic diseases. Despite this shorthand nomenclature, however, the preponderance of available evidence distinguishes aneurysmal and occlusive aortic diseases as distinct entities, each with their own characteristic pathogenic features and natural histories.
Although diameter is the anatomic feature most closely correlated with AAA rupture risk [12], the incidence of rupture varies among series reporting the natural history of large aneurysms not treated due to various circumstances [12–14]. As highlighted by the current Society for Vascular Surgery guidelines regarding AAA disease management, additional circumstances are known or suspected to increase the risk for AAA rupture including saccular vs. fusiform mural contour female gender or rapid enlargement at any given diameter, underscoring the reality that additional, still poorly defined, circumstances actually initiate the process of rupture [15]. Female gender, large initial aneurysm diameter, low forced expiratory volume in one second, current smoking history, and elevated mean blood pressure were all identified as specific covariates predicting risk of AAA rupture in the United Kingdom Small Aneurysm trial [16].
The following sections summarize current hypotheses regarding AAA pathogenesis, with particular emphasis on features associated with rupture. Limitations on the latter necessarily stem from the emergent and unpredictable nature of clinical aneurysm progression, limiting access to human AAA tissue immediately preceding the moment of rupture, and the lack of biomarkers or validated animal models to guide mechanistic investigations [17].
Proteolysis and Disease Progression
Elastin and collagen type I and III are key structural components of the aortic wall, both extensively investigated in the pathogenesis of AAA disease [6–9, 18–23]. A true pioneer in the field, Phil Dobrin, with colleagues reported that elastin degradation leads to vessel dilation and decreased distensibility, whereas collagen degradation produces greater dilation and ultimate vessel rupture [6, 7]. The accelerated elastin degradation in AAA pathogenesis leads to the phenomenon of collagen loading, where progressively attenuated aortic medial collagen fibers bear a greater share of superimposed hemodynamic strain.
Degradation of elastin and collagen is primary mediated by proteases expressed by constitutive and infiltrative aortic cells, including matrix metalloproteinases (MMPs), serine proteases, cathepsins, and related enzymes [8, 9]. MMPs are classified according to their substrate specificity [24] (Fig. 4.3). Of the relevant MMPs, many prior studies have suggested a strong relationship between MMP-9 and AAA rupture [18–23]. MMP-9 is primarily expressed from infiltrating macrophages, highlighting the importance of inflammatory rupture pathogenesis [20]. Expression and activity of MMP-2 are also characteristically elevated in both ruptured and non-ruptured AAA tissue [19, 20, 25]; however, MMP-2 may be related more to expansion rather than rupture [21]. Additional MMPs are also elevated in the setting of rupture but with less frequency than MMP-2 and MMP-9 [22, 23].


Fig. 4.3
Matrix metalloproteinases and their substrates. MMP matrix metalloproteinases, MT membrane type (Reproduced from Chistiakov et al. [24], with permission from Lippincott Williams & Wilkins)
Tissue inhibitors of metalloproteinases, or TIMPs, are regulatory molecules intimately related to ECM homeostasis and renewal. The role of TIMP activity, or the lack of it, in AAA progression remains uncertain. TIMP-1 binds to MMP-9; this interaction is proposed to be central to the pathological processes of AAA progression [26, 27]. Allaire et al. reported that local overexpression of TIMP-1 prevented AAA rupture in a rat model [18]. Other studies have demonstrated significant endogenous upregulation and expression of TIMP-1 in rat AAA rupture models [28], and no difference was observed in TIMP expression in tissues harvested from ruptured and intact human AAAs [21–23].
Tissue-type plasminogen activator (tPA) and urokinase-type plasminogen activator (uPA) are serine proteases that activate plasminogen and have central roles in blood coagulation and fibrinolysis. In terms of AAA development, Reilly et al. reported that tPA is diffusely present both in the intima and media, and uPA is present only in the infiltrative monocellular cells in the adventitia of the AAA wall [29]. Since plasmin is a potential activator of pro-MMPs [28] and both uPA and tPA can upregulate MMP-2 and MMP-9 [30], uPA and tPA have been reported to contribute to AAA progression [31]. Also, uPA and tPA seem to contribute to the AAA progression through induction of cytokines like IFN-γ, TNF-α, and inflammatory chemokines like monocyte chemoattractant protein-1 (MCP-1) and macrophage inflammatory protein-2 (MIP-2), which lead to monocytes recruitment [30].
There seems to be positive relationship between serine proteases and other related plasminogen activators/inhibitors like plasminogen activator inhibitor-1 (PAI-1) with AAA progression [31], but the effect of serine proteases on AAA rupture has not been investigated thoroughly especially using human AAA tissues [32, 33]. Results from aneurysm models have been mixed [18, 28, 34, 35]. For example, Uchida and associates reported augmented AAA rupture in the angiotensin II infusion in apolipoprotein E-deficient mouse model (Ang II/Apo E -/-) by inhibition of uPA activator in bone-marrow-derived cells [35]. Thus further research is warranted.
Cathepsins are class of lysosomal proteases with high proteolytic activity, also recognized as potential effectors of AAA pathogenesis. Human cathepsins can be classified to B, C, D, F, G, H, K, L, O, S, V, W, and X subtypes, most are either cysteine or aspartic proteases [36]. Expressions of cathepsins in AAA wall and serum are significantly increased compared to control samples [37, 38], and known AAA risk factors such as cigarette smoking, hypertension, and atherosclerosis are all known to induce cathepsin secretion through injury of vascular endothelial cells [36]. Furthermore, pharmacologic cathepsin inhibition inhibits AAA formation in experimental AAA models, reinforcing the potential link to AAA disease [39, 40]. Unfortunately, the role of cathepsins in AAA rupture remains uncertain, however, limited by circumstances similar to those previously discussed.
The concept of progressive aortic mural proteolysis as a modifiable pathogenic mechanism in AAA pathogenesis has been questioned recently on the basis of the Dutch PHAST trial. In this controversial multicenter trial, doxycycline was found to be ineffective in preventing progression of early AAA disease. Rupture, however, was not evaluated as a primary end point [41]. A recent multicenter study (AORTA trial) trialing mast cell inhibitor, which inhibits both MMP-9 and cathepsin G, was also unsuccessful [42]. The work of Shen et al. underscored a potential dual role for MMP-2 in extracellular homeostasis in aneurysm pathogenesis, demonstrating a differential effect of MMP-2 ablation in the progression of thoracic and abdominal disease [43].
Together with the recently reported clinical trial data, these data underscore the complexity of aortic mural homeostasis, and the interplay by which concurrent processes of matrix deposition and degradation occurs. Similar to the concept of autophagy, it is likely that constitutive aortic cells maintain matrix integrity by continuous and concurrent processes of both creation and destruction, and the paucity of medial smooth muscle cell cellularity present in advanced aneurysmal disease leads to progressive deterioration and degeneration of the extracellular matrix (ECM) on the basis of cellular neglect rather than disproportionate proteolysis. Regardless, a larger randomized controlled trial is reexamining the ability of doxycycline, an MMP inhibitor, in limiting progression of early AAA disease (NTA3CT trial, clinicaltrials.gov #NCT01756833). This trial will likely settle the “doxycycline” question once and for all, at least for early (<5 cm in diameter) AAA disease. Remaining to be determined will be the role of proteolysis as a distinct pathogenetic process in AAA disease and whether inhibition with doxycycline can prevent rupture of advanced aneurysms, as the latter are specifically excluded from the study.
Inflammatory Mediators
The role of pro-inflammatory chemokines and cytokines expressed by infiltrative inflammatory cells has been extensively investigated in AAA pathogenesis using both human surgical specimens and animal models [1, 2, 4, 44]. For example, the pro-inflammatory cytokine interleukin (IL)-1β is significantly overexpressed in human AAA tissue [45]. Johnston and associates were able to inhibit experimental AAA formation and progression by genetic and pharmacologic inhibition of IL-1β expression [46]. As was the case with MMPs, however, the influence of IL-1β or any specific chemokine/cytokine on AAA rupture remains unknown.
IL-6 is a pro-inflammatory cytokine also known to be overexpressed in explanted human AAA tissue [47]. The importance of IL-6 is underscored by its broad recognition as a systemic marker of increased cardiovascular mortality [48]. Circulating IL-6 levels are increased in ruptured AAA patients compared to patients undergoing elective AAA repair [49, 50]. Similar results have been observed in experimental models of AAA rupture model [28]. Cheuk and associates suggested that since no significant relationship has been recognized between AAA diameter and circulating IL-6 levels, IL-6 may be uniquely related to rupture independent of size [51]. Plasma levels of IL-10, generally recognized as an anti-inflammatory cytokine, have also been demonstrated to be elevated in ruptured AAA patients [50] and are also elevated in animal modeling experiments [28]. Like the MMP-TIMP dyadic, the role of IL- 6 and IL-10 in promoting or preventing aneurysm rupture remains to be defined in higher-fidelity modeling systems yet to be developed.
As to the significance of specific cytokines or chemokines on aneurysm rupture, it remains highly controversial. Wilson and associates reported that tissue (rather than circulating) expression of inflammatory mediators did not differ between intact AAAs and non-ruptured segments of ruptured AAAs, also underscoring the concept that the stimulus for rupture itself, in the advanced but still intact aneurysm, is highly dependent on local (potentially physical) factors. For the record, Wilson et al. also identified lower levels of IL-1β and reduced lymphocyte density at the site of rupture itself vs. intact segments of ruptured AAA. On the basis of their results, these investigators concluded that the biological events leading to AAA rupture may not be dependent on upregulation of the inflammatory process [52]. This observation underscores the growing recognition that processes of aneurysm progression and rupture (as well as initiation) are likely distinct and relatively unique to each respective phase of the disease, and that agents or interventions effective in limiting early or mid-disease progression may not prevent rupture of advanced AAA. A related question remains as to whether the advanced aortic inflammatory response present at the site of rupture precedes the event, or whether inflammation is focally stimulated by the process of rupture itself remains an important area of future investigation.
Infiltration of Inflammatory Cells
Aortic mural inflammatory cell infiltration, especially the localization of macrophages within the adventitia, is a central pathologic feature of AAA development [2]. Adventitial macrophage density is increased at sites of aortic rupture compared to intact AAA tissue in both clinical [53] and experimental [18, 28] specimens. As previously mentioned, infiltrative inflammatory macrophages (M1 phenotype in the classical characterization) are the predominant source for aortic MMP-9 [20, 54]. These activated macrophages are believed to be strongly related with the pathogenesis of rupture. On the other hand, M2 phenotype macrophages, thought to limit the inflammatory response and promote tissue repair through transforming growth factor-β2 (TGF-β2), may inhibit AAA disease progression [55, 56]. Sakalihasan and associates have demonstrated that inflammatory macrophage localization (as determined by 18F-FDG uptake during positive emission tomography) is characteristic of symptomatic aneurysms and have suggested that this modality may hold promise in predicting impending rupture [57] (Fig. 4.4). Other investigators have failed to identify differences in CD-68- and CD-15-positive cell densities between the site of the rupture and intact AAA wall, however, questioning the broad applicability of macrophage-based molecular imaging strategies in predicting rupture. The recognition that CD-45 cells were less prevalent at the site of rupture again raised the possibility that inflammation itself may be less related to rupture than it is to the process of gradual aneurysm enlargement prior to rupture [23], as previously noted above.


Fig. 4.4
Example of positive 18F-FDG PET/CT representing dense infiltration of leukocytes in the adventitia. (a) Transaxial PET (b) CT (c) fused PET/CT images. Red arrow, focus of increased activity; white arrow, absence of uptake by thrombus; arrowhead, mild uptake by intra-aortic blood pool; red circle, intraoperative tissue sampling for positive area; white circle, intraoperative tissue sampling for negative area (Reproduced from Courtois et al. [57], with permission from the Society of Nuclear Medicine and Molecular Imaging)
Even less evidence is available for the role of inflammatory cells other than macrophages in AAA rupture. Granulocytes, or neutrophils, are the first cell type to infiltrate thrombus [58], store and release uPA from granules [59], and express MMP-9 in the experimental models of aortic dissection [60]. Increased mural neutrophil infiltration has been observed in experimental models of AAA rupture as well [28]. In human-ruptured AAA tissue, however, a paucity of neutrophils has been identified, highlighting the challenges previously noted in experimental investigations of aneurysm rupture [23, 61]. In summary, although inflammation is unquestionably related to AAA pathogenesis and progression, aortic mural inflammation may be less relevant to the process of aortic tensile failure and rupture in the setting of end-stage disease. Significantly, accelerated AAA progression and rupture have been previously noted in solid organ transplant recipients on massive anti-inflammatory/antirejection medical regimens [62]. Unquestionably, the specific role that inflammatory cells play in precipitating aortic rupture, if any, remains to be determined.
Intraluminal Thrombus
The role of intraluminal thrombus (ILT) in promoting or preventing aneurysm rupture has been extensively debated for years [63–65]. Although ILT may reduce AAA wall strain [63], paradoxically advanced ILT has also been associated with accelerated aneurysm enlargement [66]. Empirically, laminar ILT has been shown to induce relative hypoxia in adjacent aortic segments, in turn promoting inflammation [64], MMP-9 activation [26, 67], and downregulation of mural protein synthesis [68]. Many investigators have attempted to draw correlations between ILT burden and deposition pattern and aneurysm size and clinical outcomes based on cross-sectional imaging datasets [65, 69]. However, despite demonstrating a positive correlation between AAA diameter and ILT volume, this ratio was not useful in distinguishing ruptured from intact AAAs, either in clinical series [70] or experimental modeling [28]. Also, Golledge and colleagues recently reported that ILT volume was similar between ruptured and intact AAA [71], making the role of ILT in rupture process even more obscure.
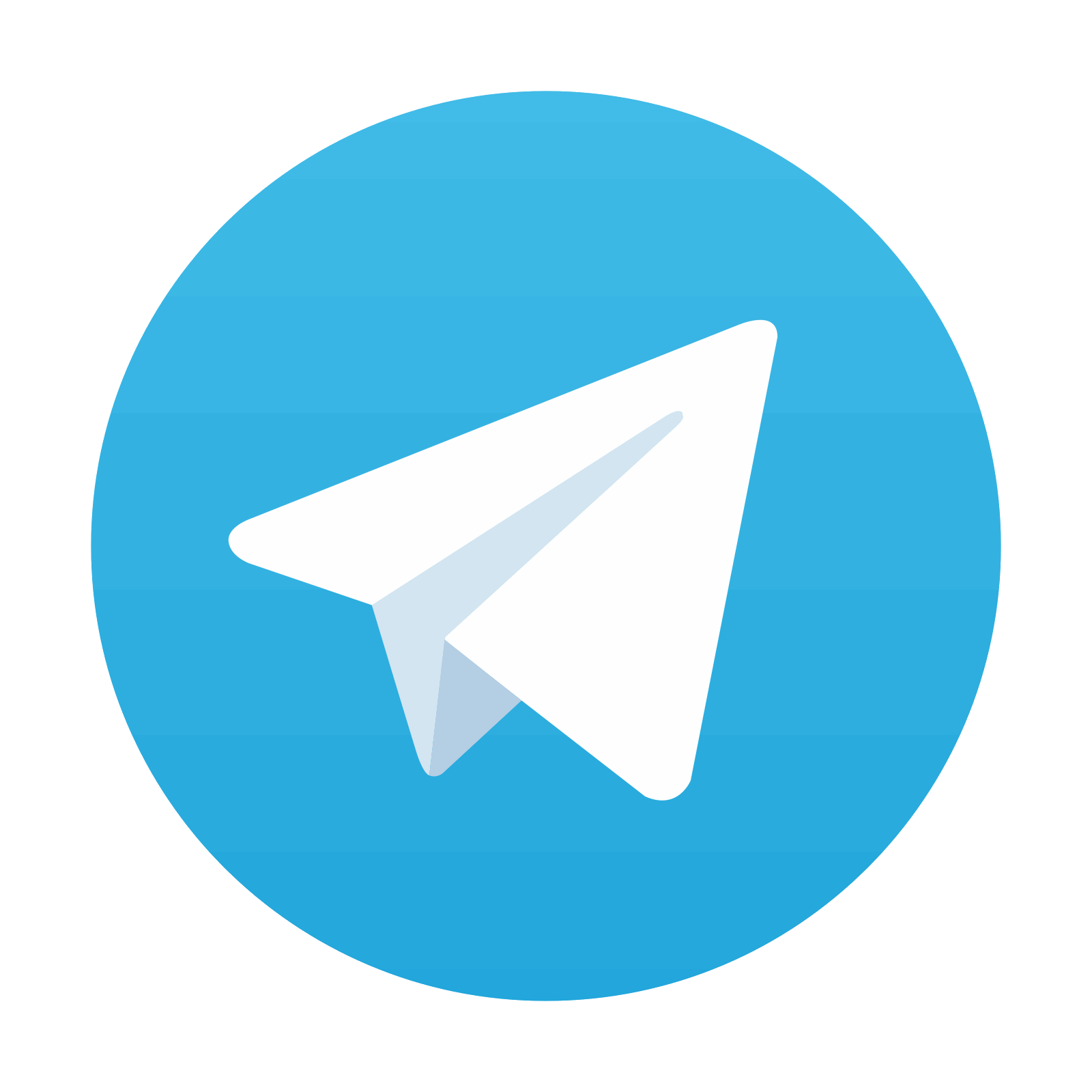
Stay updated, free articles. Join our Telegram channel

Full access? Get Clinical Tree
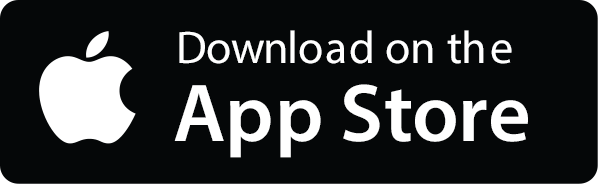
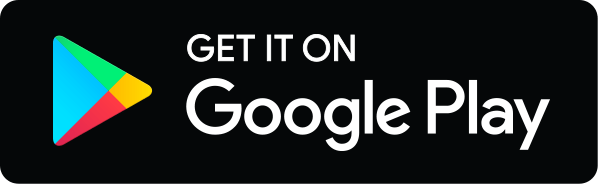