Fig. 5.1
HBV carcinogenesis . HBV contributes to HCC by (a) insertional mutagenesis due to integration of the viral DNA into host chromosomes; (b) increased genomic instability caused by both viral integration and the activity of the viral protein HBx; (c) modifications of the epigenome promoted by HBx and HBc; (d) modulation of cell death and proliferation pathways by the prolonged expression of viral proteins (wild-type and mutant HBx, LHB envelope proteins, truncated MHB proteins, HBc)
HBx Protein
HBx regulatory protein is both required for HBV cccDNA transcription/viral replication [29, 127], and thought to contribute to HBV oncogenicity . Although the mechanisms underlying these pleiotropic activities of HBx have not been fully elucidated, regulation of transcription, through direct nuclear (transcription and chromatin) and/or indirect cytoplasmic (cell signaling) mechanisms , is thought to play an important role (Fig. 5.2). In the following subsections we provide a rather comprehensive description of the vast scientific literature reporting on HBx activities over almost two decades. We have underlined wherever possible the relevance of those results that have been generated in the context of HBV replication/infection systems and/or conformed in either in vivo animal models or through the ex vivo evaluation of CHB- and HBV-related HCC patients samples.


Fig. 5.2
Multiple cellular targets of the regulatory protein HBx. The regulatory protein HBx, in addition to be required for viral replication, contributes to hepatocytes transformation by multiple mechanisms, mediated by its interaction with a large number of cellular targets. HBx physically interacts with several cellular proteins that modulate cell proliferation, cell death, transcription, and DNA repair
HBx, Chromatin and Viral/Cellular Transcriptional Control
HBx is recruited to the cccDNA minichromosome in HBV-replicating cells to increase transcription of the nuclear cccDNA minichromosome [29, 127]. In the absence of HBx, cccDNA-bound histones are hypoacetylated, and the cccDNA transcribes significantly less pgRNA [29]. HBx also binds and blocks the inhibitory activity on HBV transcription exterted by the PRMT1 methyl-transferase [30] and the Tudor-domain protein Spindlin-1 [128]. Additional mechanisms by which HBx can potentiate HBV replication include the down-regulation of DNMT3A expression through the induction of miR-101 [129], induction of endocytosis and autophagy that are required for viral replication [130–132], binding to the UV-DDB1 protein [133], and elevation of cytosolic calcium levels [134].
ChIP-Seq genome-wide analysis of HBx chromatin recruitment in HBV-replicating cells revealed a specific binding of HBx to a large number of new and known HBx target sequences [135], including protein-coding genes and ncRNAs [16 lncRNA promoters and 32 lncRNA intragenic regions, 44 snoRNA, 3 snRNA, and 75 microRNA promoter regions]. 39 out of the 75 HBx-targeted miRNAs are classified as intragenic and 15 of them display HBx peaks in the promoter region of their target genes. Multiple transcription factors seem to mediate the recruitment of HBx on the target chromatin (i.e., E2F1, CREB, β-catenin, NFkB) [135]. Pathway analysis of the protein-coding genes and miRNAs potentially regulated by HBx showed an enrichment in genes/ncRNAs involved in cell metabolism, chromatin dynamics and cancer but also in genes/ncRNAs that modulate HBV replication. (Ras, calcium transport, endocytosis, MAPK/WNT pathways, Src, the EGF/HGF family). Functional experiments identified new mechanisms by which HBx, in addition to its activity on the viral cccDNA, boosts HBV replication, mediated by direct transcriptional activation of genes and miRNAs that potentiate endocytosis (RAB family) and autophagy (ATGs, beclin-1, miR-33a) and the transcriptional repression of miRNAs (miR-138, miR-224, miR-596) that directly target the HBV pgRNA and would inhibit HBV replication [135].
Mechanistically, the activity of HBx on transcription of both cellular genes and the viral genome rely on the interaction with transcription factors and chromatin modifying enzymes and the modulation gene expression through epigenetic modifications (Fig. 5.3). Indeed, HBx binds several nuclear proteins involved in the regulation of transcription including component of the basal transcriptional machinery (RPB5, TFIIB, TBP, TFIIH), coactivators (CBP, p300, and PCAF) and transcription factors (ATF/CREB, ATF3, c/EBP, NF-IL-6, Ets, Egr, SMAD4, Oct1, RXR receptor, p53) [4]. HBx binds in vivo to the promoters of a number of CREB-regulated genes and increases the recruitment of CBP/p300 to these promoters leading to increased gene expression [136]. More recently, the same group has also shown that HBx prevents the inactivation of CREB by a PP1 (protein phosphatase 1)/HDAC1 complex [31].


Fig. 5.3
HBx and chromatin dynamics. HBx binds several nuclear proteins involved in chromatin dynamics and the regulation of transcription: (a) HBx binds in vivo and increases the recruitment of CBP/p300 to the promoters of CREB-regulated genes (upper left panel); (b) HBx induces the PLK1- and proteasomal-dependent degradation of Suz12 [a component of the polycomb repressive complex 2—PRC2, that directs the (tri)methylation of lysine 27 on histone 3 (H3K27Me3) and gene silencing], leading to the overexpression of Suz12/PRC2 direct target genes, including the hepatic cancer stem cell markers BAMBI and EpCAM (lower left panels); (c) in Suz12-depleted cells expressing HBx a regional DNA de-methylation mediated by a complex containing Ezh2, TET2 and DNMT3L around half-NFkB sites releases the expression of EpCam and DLK1 expression (lower right panel) (d) HBx downregulates gene and ncRNAs transcription by (1) promoting the re-location of the DNMT3a DNA methyl-transferase to facilitate the regional hypermethylation of the promoters of certain tumor-suppressor genes, such as p16/INK4A and (2) by converting positive into negative transcription factors complexes (upper right panels)
HBx also increases total DNA methyltransferase (DNMT) activity by the upregulation of DNMT1, DNMT3A1, and DNMT3A2 [137] and, by relocating DNMT3a [138], selectively facilitates the regional hypermethylation of the promoters of certain tumor-suppressor genes, such as p16/INK4A (Fig. 5.3).
Animal models of HBx- and HBV-mediated tumorigenesis downregulate the chromatin-modifying proteins Suz12 [a component of the polycomb repressive complex 2—PRC2, that directs the (tri)methylation of lysine 27 on histone 3 9H3K27Me3) and gene silencing] and ZnF198 [that stabilizes the LSD-Co-RESR-HDAC1 repressor complex] in liver tumors [110]. SUZ12 and ZNF198 are targeted to poly-ubiquitibnation and proteasomal degradation by a Plk1-dependent phosphorylation that is enhanced by the long noncoding RNA HOTAIR that serves as a bridge between the PRC2 and the LSD-Co-RESR-HDAC1 repressor complexes [139]. Suz12/Znf198 downregulation is accompanied, both in animal models and human HBV-related HCCs, by the overexpression of a number of Suz12/PRC2 direct target genes, including the hepatic cancer stem cell markers BAMBI and EpCAM [110] (Fig. 5.3). EpCAM over-expression in hepatic cells that have lost Suz12 is mediated by HBx and involves an active demethylation of CpG dinucleotides flanking NF-κB-binding sequences and the formation of a multi-protein complex containing the transcription factor RelA, the methyltransferase EZH2, the TET2 enzyme catalyzing the conversion of 5-methylcytosine to 5-hydroxymethylcytosine, and the catalytically inactive DNMT3L [140] (Fig. 5.3).
HBx, Oxidative Stress, and Apoptosis
HBx has been shown to have both pro-apoptotic and anti-apoptotic properties, depending on its levels, the cell context (i.e., quiescent hepatocytes, neoplastic or preneoplastic liver cells with defective growth control, liver progenitor cells) and the experimental system used. In HBV replicating cells HBx promotes cytosolic calcium signaling, resulting in Ca2+ accumulation in mitochondria, increased levels of ROS [134] and the activation of the PYK2 and SRC kinases, which also promote HBV replication [32]. HBx also binds to mitochondrial voltage-dependent anion-selective channel protein 3 (VDAC3) [141], leading to membrane depolarization, reactive oxygen species (ROS) production [141] and eventually apoptosis [142]. Ca2+ signaling and increase ROS levels trigger ER stress and the unfolded protein response (UPR) [143]. On the other hand, high levels of HBx have been reported to block tumor necrosis factor-α (TNFα)- and FAS-mediated apoptosis by activation of NFκB [144], suggesting that infected hepatocytes may survive immune-mediated damage whereas uninfected hepatocytes undergo apoptosis in CLD.
HBx, Epithelial–Mesenchymal Transition, and Fibrogenesis
Epithelial–mesenchymal transition (EMT) plays multiple roles in the pathogenesis of CLD by promoting fibrogenesis, tumor progression, and metastasis. TGFβ is central to EMT by inducing collagen synthesis and promoting transcription factors that suppress epithelial markers [145–147]. HBx upregulates TGFβ1 [148] by SMAD-dependent (via stabilization of the SMAD4 complex) [149] and non-SMAD-dependent pathways (via activation of RAS–ERK and PI3K–AKT) [150]. HBx, similar to HCV core [151, 152], also seems to convert TGFβ1 signaling from negative to positive growth regulation and shift TGFβ responses from tumor suppression to EMT. Liver fibrogenesis (type 2 EMT) and HCC metastasis (type 3 EMT) are also mediated by miR 21, which is upregulated by NFκB in HBV-associated HCC [153]. As miR 21 activation usually occurs early during HBV CLD progression, when ROS and HBx stimulates NF-κB and AP1, these observations link HBx, chronic inflammation, and hepatocytes transformation. HBx-stimulated SRC signaling promotes EMT [154] by destabilization of adherens junctions [155]. HBx also suppresses E cadherin by promoter DNA methylation and by upregulating SNAIL [156].
HBx, Hypoxia, and Angiogenesis
Cirrhotic nodules have a “relative” defect of vasculature that may generate local reductions in oxygen tension and hypoxia, upregulate HIF1α expression, and promote angiogenesis. HBx binds to and stabilizes HIF1α and stimulates HIF1α transcription [157], thus promoting angiogenesis and cell “stemness.” HBx also promotes angiogenesis by upregulating the pro-angiogenic growth factor angiopoietin 2 (ANG2) [158]. HIF1α is also stabilized by increased insulin-like growth factor receptor 1 (IGFR1), epidermal growth factor receptor (EGFR) and PI3K–AKT signaling that are all activated by HBx [159].
HBx and Hepatic Stem/Progenitor cells (HSCs/HPCs)
Stemness markers [such as NANOG, OCT4, SOX2, and Krüppel-like factor 4 (KLF 4)] are reactivated and expressed in HCC [160]. About 20–40 % of HCCs display phenotypic markers of hepatic progenitor cells (HPCs) [161, 162] and share a common transcriptional signature with normal HPCs in cDNA microarray-based analysis [163]. HCCs expressing progenitor cell features have a worse prognosis and higher recurrence after treatment compared to HCCs, which are negative for these markers analysis [163]. Although a clinicopathological analysis of surgically resected HCC specimens suggested that EpCAM+ CSCs were more frequently detected in HBV-related HCCs than in HCV-related HCCs [162] a validation on larger independent cohorts including HCCs from multiple etiologies is still lacking. HPCs (also called oval cells in rodent models of carcinogenesis) are small epithelial cells that can differentiate towards both hepatocytes and cholangiocytes and are located in the smallest branches of the biliary tree (canal of Herring and/or the ductular compartment). In animal models, liver cancers can originate from hepatocytes as well as from immature progenitor cells [164]. HBx promotes the expression of NANOG, KLF4, OCT4, and MYC as well as EpCAM (epithelial cell adhesion molecule) and β-catenin [160]. Stabilization of β-catenin transcriptionally upregulates EpCAM [160] and promotes the transcription of stemness genes in association with TCF/LEF1, OCT4, and NANOG. EpCAM+ cells display CSC-like properties and generate invasive tumours in HCC xenograft experiments [162]. HBx also promotes the expression of miR 181 family members, which upregulate EpCAM [165] and are highly expressed in embryonic livers, in HSC , and in patients with α-fetoprotein (AFP)-positive tumours [165].
HBx, Senescence, and Telomeres
Inflammation, oxidative, and oncogenic stress accelerate cellular senescence in chronic HBV (and HCV) infections. In cirrhotic livers, hepatocytes display decreased proliferation rates with a dominant replicative senescence phenotype, critically shortened telomeres and reduced regenerative potential [1]. Indeed, the length of telomeres progressively shortens from normal liver to chronic liver disease, and reaches the shortest levels in HCC [166, 167]. Senescence limits the proliferation of damaged cells and reduces the risk of malignancy by triggering the expression of tumor suppressors [168]. Transformed hepatocytes must bypass senescence and can survive despite critically shortened telomeres. Many studies have indeed showed that 80–90 % of HCCs display a high telomerase activity [169]. TERT promoter mutations activating telomerase expression represent the single most frequent genetic alteration in HCC [170, 171] but are less represented in HBV-related HCCs that re-activate TERT by other mechanisms including the integration of HBV DNA sequences into the TERT gene [111, 113, 121, 122] and the upregulation of TERT expression by HBx and PreS2 proteins [172]. Despite TERT activation telomers remain very short in HCC cells, predisposing to occasional telomere instability, chromosomal instability and polyploidy [172]. Indeed, the majority of HCC cells display a high incidence of chromosome instability that, similar to the accumulation of senescent cells [1], is already evident in cirrhotic liver tissues and increases during the hepato-carcinogenesis process [173]. LOH rate is higher in HBV-related HCCs [173] and HBx directly induces chromosomal instability by affecting the mitotic checkpoints [174]. HBx also binds and inactivates p53 and interacts with the DNA repair protein DDB1, which in turn affect repair functions and allow the accumulation of genetic changes [32]. RAS signaling and the AKT–ARF–p53–p21 and RAS–MEK–ERK–INK4A/p16–RB pathways, linked to oncogene-induced senescence (OIS) [175], are both active in HCC and are activated by HBx [176–178]. At the same time, HBx contributes to overcoming senescence by: a) upregulating DNA methyltransferases (DNMTs) [89]; b) inhibiting the p53 nucleotide excision repair and transcription-coupled repair functions [179]; and c) decreasing the expression of the p53 activators ASPP1 and ASPP2 [92]. HBx also suppresses the cyclin-dependent kinase (CDK) inhibitors INK4A and p21 via promoter methylation, resulting in the inactivation of the RB tumor suppressor [180]. miR 221, which is upregulated in HBV- (and HCV)-related HCCs, blocks the expression of the CDK inhibitor p27 and promotes tumor growth and progression by activation of the PI3K–AKT–mTOR pathway [181]. HBx also interacts with the peptidyl-prolyl cis/trans isomerase Pin1 and this interaction leads to HBx stabilization, enhanced HBx-mediated transactivation of target genes, and increased cellular proliferation [182].
HBx, Tumor Promotion, and Tumor Progression
Despite the large number of published studies, we still lack a unifying picture of HBx role in liver carcinogenesis that reconcile all HBx reported activities. Both wild-type HBx and truncated HBx proteins could demonstrate oncogenic functions and promote tumorigenesis [183–186]. However, it is not yet clear whether mutated HBx proteins “gain” oncogenic functions or rather “lose” activities that would restrain the oncogenic potential of wild-type HBx or that would not be no longer required for tumor progression. The recent demonstration in a large series of HBV-related HCCs that premature stop codon and large deletions leading to a complete inactivation of the HBx gene are selected and accumulate in the tumors in contrast to the surrounding non-tumor liver tissues in more than 70 % of the tumors suggests that HBx inactivation could have a role in liver carcinogenesis or tumor progression. The reported correlation between HBx inactivating mutations, the presence of TP53 mutations, a G1–G3 transcriptomic profile [83], an abnormal expression of onco-fetal genes (EPCAM, AFP and KRT19), and poorer prognosis [84] adds a further layer of complexity to the understanding of HBx contribution to HCC development.
HBc Protein
PreS/S Proteins
The potential pro-oncogenic role of mutated envelope proteins has been confirmed in many studies in transgenic mice and cell cultures [48, 61, 190–193]. PreS2 mutants may induce cyclin A and cyclooxygenase-2 overexpression leading to cell proliferation and anchorage-independent growth [65, 66]. PreS2 mutated proteins also directly interact with the Jun activation domain-binding protein 1 (JAB1), thus triggering cyclin-dependent kinase (Cdk) inhibitor p27 degradation, Retinoblastoma hyper-phosphorylation and cell cycle progression [64]. Cyclin A is located in the cytoplasm rather than in the nucleus in preS2 mutant-transgenic mice where favors centrosome over-duplication and consequently chromosome instability [61, 66]. Finally, the ER stress response induced by preS-mutated proteins increases vascular endothelial growth factor-A (VEGF-A) expression [193]. PreS/S sequences deleted at the 3′-end and producing functionally active MHBst are found in many viral integrates from HBV-associated HCCs [50, 190, 194–196]. MHBst proteins retained in the ER trigger a PKC dependent activation of c-Raf-1/MEK/Erk2 signal transduction cascade, induction of AP-1 and NF-kB transcription factors, and an enhanced proliferative activity of hepatocytes [191, 197]. MHBst directly interact with a preS2-responsive DNA region in the hTERT promoter, resulting in the upregulation of telomerase activity and in the promotion of HCC development [192]. On the other hand, the inappropriate expression and accumulation of wild-type large envelope protein in ER membranes can be directly cytotoxic to the hepatocyte and initiate a cascade of events that ultimately progress to malignant transformation [59, 198].
Conclusions
HBV is a major risk factor worldwide for developing HCC. HBV contributes to hepatocellular carcinoma (HCC) development through direct and indirect mechanisms. Productive HBV infections triggers inflammation, continuous necrosis mediated by the immune response against infected hepatocytes, and cell regeneration favoring the accumulation of genetic and epigenetic lesions. HBV DNA integration into the host genome occurs at early steps of clonal tumor expansion and induces genomic instability and eventually direct insertional mutagenesis. Prolonged expression of the viral regulatory protein HBx and the large envelope protein deregulate the cellular transcription program and proliferation control and sensitize liver cells to carcinogenic factors. Epigenetic changes targeting the expression of tumor suppressor genes occur early in the development of HCC. A major role is played by HBx that is recruited on cellular chromatin and modulates chromatin dynamics at specific gene loci. Genome wide approaches begin to identify homogeneous subgroups of HBV-related tumors with defined genotypes and signaling pathways alterations.
References
1.
El-Serag HB, Rudolph KL. Hepatocellular carcinoma: epidemiology and molecular carcinogenesis. Gastroenterology. 2007;132:2557–76.PubMed
2.
Venook AP, Papandreou C, Furuse J, de Guevara LL. The incidence and epidemiology of hepatocellular carcinoma: a global and regional perspective. Oncologist. 2010;15 Suppl 4:5–13.PubMed
3.
Cancer IARC. Globocan. Estimated cancer incidence, mortality and prevalence worldwide in 2012. World Health Organization; 2012: 9, http://globocan iarc fr/Pages/fact_sheets_cancer aspx Accessed 2015.
4.
Guerrieri F, Belloni L, Pediconi N, Levrero M. Molecular mechanisms of HBV-associated hepatocarcinogenesis. Semin Liver Dis. 2013;33:147–56.PubMed
5.
McGivern DR, Lemon SM. Virus-specific mechanisms of carcinogenesis in hepatitis C virus associated liver cancer. Oncogene. 2011;30:1969.PubMedCentralPubMed
6.
Moeini A, Cornellà H, Villanueva A. Emerging signaling pathways in hepatocellular carcinoma. Liver Cancer. 2012;1:83–93.PubMedCentralPubMed
7.
Nault JC, Zucman-Rossi J. Building a bridge between obesity, inflammation and liver carcinogenesis. J Hepatol. 2010;53:777–9.PubMed
8.
Nault JC, Zucman-Rossi J. Genetics of hepatocellular carcinoma: the next generation. J Hepatol. 2014;60:224–6.PubMed
9.
Villanueva A, Minguez B, Forner A, et al. Hepatocellular carcinoma: novel molecular approaches for diagnosis, prognosis, and therapy. Annu Rev Med. 2010;61:317–28.PubMedCentralPubMed
10.
Villanueva A, Llovet JM. Targeted therapies for hepatocellular carcinoma. Gastroenterology. 2011;140:1410–26.PubMedCentralPubMed
11.
Fattovich G, Stroffolini T, Zagni I, Donato F. Hepatocellular carcinoma in cirrhosis: incidence and risk factors. Gastroenterology. 2004;127(5 Suppl 1):S35–50.PubMed
12.
Raimondo G, Allain JP, Brunetto MR, et al. Statements from the Taormina expert meeting on occult hepatitis B virus infection. J Hepatol. 2008;49:652–7.PubMed
13.
Ahn SH, Park YN, Park JY, et al. Long-term clinical and histological outcomes in patients with spontaneous hepatitis B surface antigen seroclearance. J Hepatol. 2005;42:188–94.PubMed
14.
Chen CJ, Yang HI, Su J, REVEAL-HBV Study Group, et al. Risk of hepatocellular carcinoma across a biological gradient of serum hepatitis B virus DNA level. JAMA. 2006;295:65–73.PubMed
15.
Di Bisceglie AM. Hepatitis B and hepatocellular carcinoma. Hepatology. 2009;49:S56–60.PubMedCentralPubMed
16.
Pollicino T, Squadrito G, Cerenzia G, et al. Hepatitis B virus maintains its pro-oncogenic properties in the case of occult HBV infection. Gastroenterology. 2004;126(1):102–10.PubMed
17.
Raimondo G, Pollicino T, Squadrito G. What is the clinical impact of occult hepatitis B virus infection. Lancet. 2005;365:638–40.PubMed
18.
Sherman M. Risk of hepatocellular carcinoma in hepatitis B and prevention through treatment. Cleve Clin J Med. 2009;76:S6–9.PubMed
19.
Wang SH, Yeh SH, Lin WH, et al. Estrogen receptor a represses transcription of HBV genes via interaction with hepatocyte nuclear factor 4a. Gastroenterology. 2012;142(4):989–98.PubMed
20.
Naugler WE, Sakurai T, Kim S, Maeda S, Kim K, Elsharkawy AM, Karin M. Gender disparity in liver cancer due to sex differences in MyD88-dependent IL-6 production. Science. 2007;317(5834):121–4.PubMed
21.
Donato F, Tagger A, Gelatti U, et al. Alcohol and hepatocellular carcinoma: the effect of lifetime intake and hepatitis virus infections in men and women. Am J Epidemiol. 2002;155:323–31.PubMed
22.
Ming L, Thorgeirsson S, Gail MH, et al. Dominant role of hepatitis B virus and cofactor role of aflatoxin in hepatocarcinogenesis in Qidong, China. Hepatology. 2002;36:1214–20.PubMed
23.
Pollicino T, Vegetti A, Saitta C, et al. Hepatitis B virus DNA integration in tumour tissue of a non-cirrhotic HFE-haemochromatosis patient with hepatocellular carcinoma. J Hepatol. 2013;58:190–3.PubMed
24.
Seeger C, Mason WS. Molecular biology of hepatitis B virus infection. Virology. 2015;479-480C:672–86.
25.
Bock CT, Schwinn S, Locarnini S, Fyfe J, Manns MP, Trautwein C, Zentgraf H. Structural organization of the hepatitis B virus minichromosome. J Mol Biol. 2001;307:183–96.PubMed
26.
Levrero M, Pollicino T, Petersen J, Belloni L, Raimondo G, Dandri M. Control of cccDNA function in hepatitis B virus infection. J Hepatol. 2009;51:581–92.PubMed
27.
Pollicino T, Belloni L, Raffa G, et al. Hepatitis B virus replication is regulated by the acetylation status of hepatitis B virus cccDNA-bound H3 and H4 histones. Gastroenterology. 2006;130:823–37.PubMed
28.
Nassal M. Hepatitis B viruses: reverse transcription a different way. Virus Res. 2008;13:235–49.
29.
Belloni L, Pollicino T, De Nicola F, et al. Nuclear HBx binds the HBV minichromosome and modifies the epigenetic regulation of cccDNA function. Proc Natl Acad Sci U S A. 2009;106:19975–9.PubMedCentralPubMed
30.
Benhenda S, Ducroux A, Rivière L, Sobhian B, Ward MD, Dion S, Hantz O, Protzer U, Michel ML, Benkirane M, et al. Methyltransferase PRMT1 is a binding partner of HBx and a negative regulator of hepatitis B virus transcription. J Virol. 2013;87(8):4360–71.PubMedCentralPubMed
31.
Cougot D, Allemand E, Rivière L, et al. Inhibition of PP1 phosphatase activity by HBx: a mechanism for the activation of hepatitis B virus transcription. Sci Signal. 2012;5(205):ra1.PubMed
32.
Bouchard MJ, Navas-Martin S. Hepatitis B and C virus hepatocarcinogenesis: lessons learned and future challenges. Cancer Lett. 2011;305.
33.
EASL clinical practice guidelines: management of CHB. J Hepatol 2009; 50: 227–42.
34.
Papatheodoridis GV, Lampertico P, Manolakopoulos S, Lok A. Incidence of hepatocellular carcinoma in chronic hepatitis B patients receiving nucleos(t)ide therapy: a systematic review. J Hepatol. 2010;53:348–56.PubMed
35.
Chan HL, Hui AY, Wong ML, Tse AM, Hung LC, Wong VW, et al. Genotype C hepatitis B virus infection is associated with an increased risk of hepatocellular carcinoma. Gut. 2004;53:1494–8.PubMedCentralPubMed
36.
Zoulim F, Locarnini S. Hepatitis B virus resistance to nucleos(t)ide analogues. Gastroenterology. 2009;137:1593–608.PubMed
37.
Locarnini S, McMillan J, Bartholomeusz A. The hepatitis B virus and common mutants. Semin Liver Dis. 2003;23:5–20.PubMed
38.
Kuang SY, Jackson PE, Wang JB, et al. Specific mutations of hepatitis B virus in plasma predict liver cancer development. Proc Natl Acad Sci U S A. 2004;101:3575–80.PubMedCentralPubMed
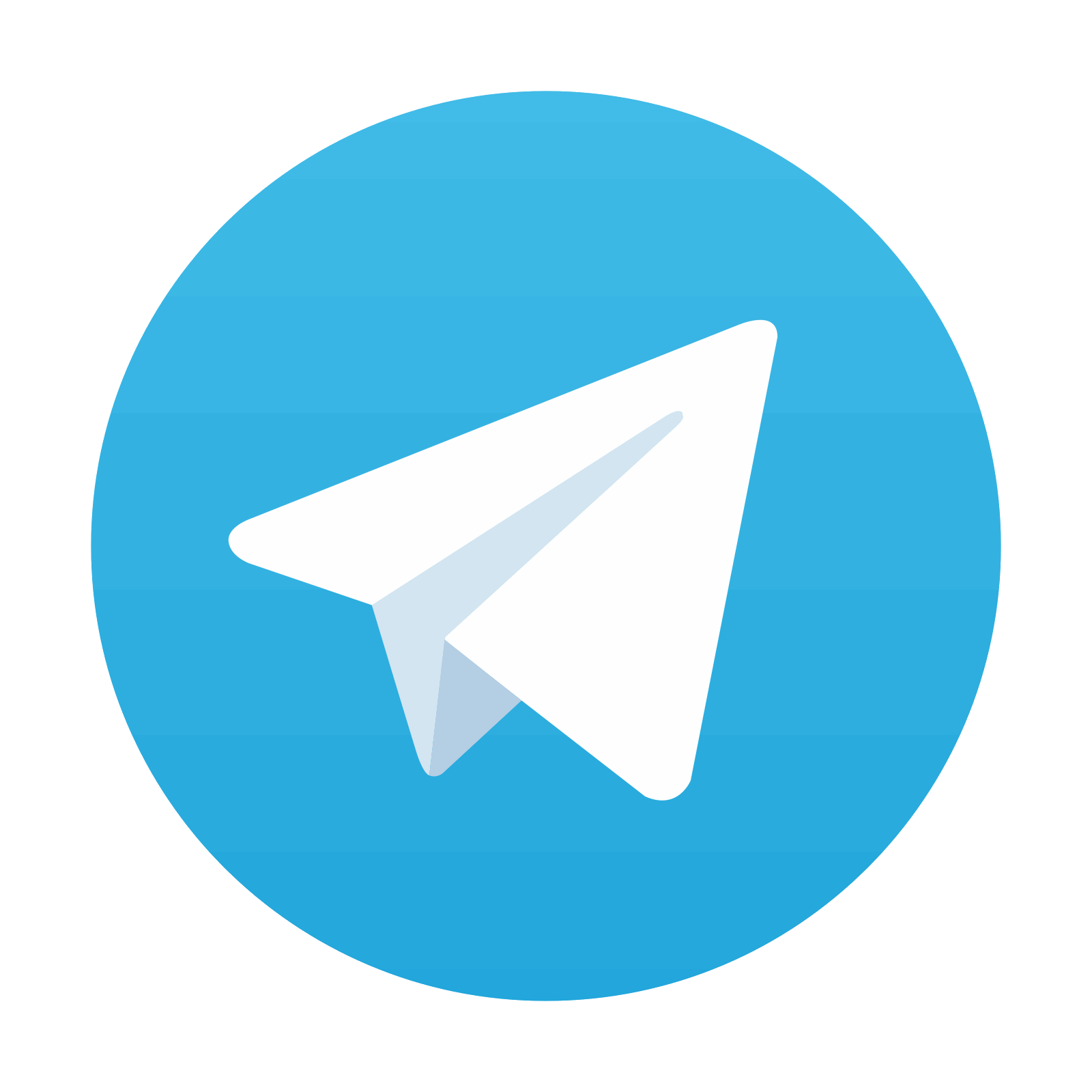
Stay updated, free articles. Join our Telegram channel

Full access? Get Clinical Tree
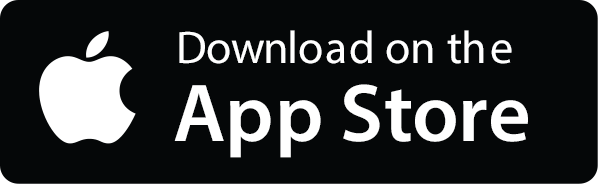
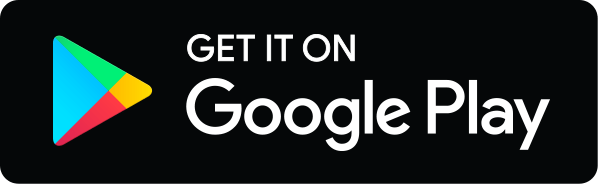