The incidence of Barrett’s-related adenocarcinoma of the esophagus continues to increase at an alarming rate. Studies to date show great promise for optical coherence tomography (OCT) in screening, surveillance, and guiding management of Barrett’s esophagus. With continued innovation in rapid, accurate scanning systems, such as volumetric laser endomicroscopy or optical frequency domain imaging, advanced OCT seems likely to have an important impact. The next few years are likely to see the initiation of large clinical studies that will define the extent and significance of this impact.
Key points
- •
The incidence of Barrett’s-related cancer of the esophagus continues to increase at an alarming rate.
- •
Ablative therapies for Barrett’s esophagus and for early neoplasia in Barrett’s esophagus are increasingly being applied for cancer prevention.
- •
Studies to date show great promise for optical coherence tomography (OCT) in screening, surveillance, and guiding management of Barrett’s esophagus.
- •
With continued innovation in rapid, accurate scanning systems, such as volumetric laser endomicroscopy or optical frequency-domain imaging, advanced OCT seems likely to have an important impact.
- •
The next few years are likely to see the initiation of larger clinical studies that will define the extent and significance of this impact.
Introduction
Optical coherence tomography (OCT) is emerging as perhaps the most important advance in imaging Barrett’s esophagus in the modern era. High-resolution white-light endoscopy and digital chromoendoscopy with magnification provide superb imaging of the mucosal surface, but nothing below the surface. Endoscopic ultrasonography (EUS) can image the entire esophageal wall thickness, but at insufficient resolution to satisfactorily detect dysplasia or early cancer. Confocal laser endomicroscopy (CLE), both endoscope-based and probe-based, can obtain dramatic cellular level imaging of the esophageal mucosa, but of small areas and at specific limited depths.
Introduction
Optical coherence tomography (OCT) is emerging as perhaps the most important advance in imaging Barrett’s esophagus in the modern era. High-resolution white-light endoscopy and digital chromoendoscopy with magnification provide superb imaging of the mucosal surface, but nothing below the surface. Endoscopic ultrasonography (EUS) can image the entire esophageal wall thickness, but at insufficient resolution to satisfactorily detect dysplasia or early cancer. Confocal laser endomicroscopy (CLE), both endoscope-based and probe-based, can obtain dramatic cellular level imaging of the esophageal mucosa, but of small areas and at specific limited depths.
Imaging of Barrett’s esophagus
Barrett’s esophagus presents a difficult problem for endoscopic imaging. The presence and extent of short-segment Barrett’s esophagus may be difficult to detect at the esophagogastric junction. The top of the gastric folds, used as a landmark for the esophagogastric junction, change with insufflation of air, and there is often a hiatal hernia causing bends and angles at the distal esophagus, making a complete view difficult. These factors are further compounded by movement associated with swallowing, belching, coughing, peristalsis, respiration, and pulsation.
Endoscopic surveillance of Barrett’s esophagus
In patients with known Barrett’s esophagus, endoscopic surveillance is performed to detect dysplasia as a risk factor for progression to cancer. Low-grade dysplasia (LGD) involves primarily cytologic abnormalities. Though comparable with those in adenoma, in Barrett’s esophagus the changes of LGD are usually flat and changes in mucosal appearance are usually not detectable by endoscopy.
High-grade dysplasia (HGD) involves changes in both cytology and tissue architecture, and may be detected in some cases using high-resolution white-light endoscopy, digital chromoendoscopy with magnification, and CLE, but unless present in visible nodules or ulcers, detection of HGD can be subtle and difficult. In addition, dysplastic changes usually occur in a mosaic-type unpredictable distribution within the Barrett’s metaplasia.
Endoscopic Biopsies
Four-quadrant random biopsies every 1 to 2 cm of Barrett’s length are recommended as the standard approach to dysplasia detection, but are notoriously underperformed, and even when correctly done sampling error is common.
Need for Red-Flag Technology
Using targeted biopsies based on abnormalities seen with high-resolution white-light endoscopy and narrow-band imaging increase the accuracy of dysplasia detection, and CLE further adds to the diagnostic yield, but sampling error persists. The requirement is for a “red-flag” or scanning technology that can accurately locate all the dysplastic areas within the Barrett’s segment for targeted biopsies or endoscopic therapy. Autofluorescence imaging combined with high-resolution white-light endoscopy and narrow-band imaging (trimodal imaging) was tested for this purpose, but false-positive rates were prohibitively high. The use of fluorescent-tagged lectins and peptide probes with specialized endoscopic systems (molecular imaging) are the subject of ongoing research projects, which are designed to localize areas of dysplasia for targeted biopsy or therapy.
Advanced OCT in Barrett’s esophagus
It would seem that advanced OCT may have the ability to meet most of the challenges of imaging in Barrett’s esophagus. The method has the potential to achieve wide-field scanning at a resolution comparable with that of low-power microscopy, detecting changes in tissue architecture that occur in Barrett’s metaplasia, dysplasia, and cancer.
The technology has the potential to:
- 1.
Identify Barrett’s metaplasia
- 2.
Detect HGD and early cancer
- 3.
Locate subsquamous (buried) Barrett’s glands
- 4.
Guide endoscopic therapies.
Analogy of EUS and OCT
Gastrointestinal endoscopists have become increasingly familiar with EUS, which is now widely available in modern endoscopy units. There are some parallels between OCT and EUS imaging. In ultrasonographic imaging, tissue structures are imaged in depth by measuring the time delay between the interrogating sound wave (emitted by a piezoelectric crystal) and the time taken by reflected sound waves to return to the crystal detector. The total depth and resolution of the image depend on the sound frequency. The sound waves require a liquid medium between the ultrasound transducer and tissue, and the best high-frequency EUS axial resolution is in the range of 100 μm.
OCT can be thought of as a technique analogous to ultrasonography; however, instead of producing an image from the scattering of sound waves, it uses infrared light from a laser and optical scattering, based on differences in tissue composition, to form a 2-dimensional image. With OCT, depth intensity can be measured by time-domain measurements, allowing for image construction in all 3 dimensions. OCT is a noncontact method. Air does not produce artifacts as in ultrasonography, and a liquid conducting medium is not required. The great benefit of OCT over ultrasonography is that it is capable of generating cross-sectional images of tissues with an axial resolution in the range of 10 μm or less, which is comparable with that of low-power (4×) bright-field microscopy. This resolution is sufficient to detect microscopic tissue architecture.
Interferometry
The speed of light is so much faster than the speed of sound that no detector is capable of directly determining the time difference between incident and reflected light. OCT uses a method called interferometry to perform this task. OCT measurements are made using low-coherence interferometry typically configured as a Michelson-type interferometer, as illustrated in Fig. 1 . The interferometer functions by splitting the beam into 2 independent paths and then recombining the 2 paths to generate interference. The depth-dependent tissue microstructure information is derived from the interference patterns. Signal-processing algorithms transform interference patterns into images than can be displayed in real time.
Initial medical uses of OCT
The first report of OCT imaging of human tissue was published in 1991 by a research group at the Massachusetts Institute of Technology, who used OCT in the laboratory for in vitro studies of the human retina and atherosclerotic plaques. The first clinical applications were in ophthalmology, where OCT has found a solid role in diagnosing and monitoring diseases such as glaucoma and macular degeneration.
In 1997, a catheter-based OCT probe was used to study the gastrointestinal tract in live animals, and a pilot study in humans was reported using a different OCT catheter-based endoscope system. In 2000, 3 different groups reported OCT imaging of the esophagus and stomach. Imaging depth in the esophagus was achieved to approximately 2 mm, allowing imaging of the submucosa. These studies showed that the normal squamous mucosa was routinely imaged as a layered structure, with layers corresponding to the epithelium, lamina propria, and muscularis mucosae.
OCT in Barrett’s esophagus
Barrett’s metaplasia has a specific appearance on OCT imaging, easily distinguished from the appearance of the gastric cardia and normal squamous mucosa. In 2001, Poneros and colleagues published the first study examining the accuracy of in vivo OCT in diagnosing Barrett’s metaplasia. Studies have found sensitivities ranging from 81% to 97% and specificities from 57% to 92% in comparison with histopathologic evaluation of endoscopic biopsies.
OCT in imaging of dysplasia
Initial studies differentiating nondysplastic Barrett’s mucosa from Barrett’s dysplasia proved to be a more subtle distinction, and somewhat less accurate. In 2005, Isenberg and colleagues published a histologic correlation study examining the accuracy of in vivo OCT in diagnosing dysplasia, and reported sensitivity of 68% and specificity of 82% with high interobserver variability.
In 2006, Evans and colleagues published a study further examining the OCT-image characteristics of HGD and intramucosal adenocarcinoma in Barrett’s esophagus. In this study, a 4-point scoring system was developed based on histopathology characteristics. A score of 2 or more resulted in sensitivity of 83% and specificity of 75%.
Recent improvements in OCT resolution are expected to result in greater OCT accuracy in the diagnosis of HGD and intramucosal carcinoma. With marked improvement in imaging speed, 3-dimensional OCT has become available, allowing a powerful combination of high resolution, large field of view, and rapid data acquisition.
Barrett’s ablation and subsquamous intestinal metaplasia
A variety of endoscopic ablation therapies has been applied to remove the metaplastic mucosal lining that characterizes Barrett’s esophagus. Most ablation procedures have been reported in patients in whom dysplasia has been detected within the Barrett’s segment.
Photodynamic Therapy
Photodynamic therapy (PDT), in which a photosensitive chemical is usually given systemically followed by photoactivation in the Barrett’s segment with a directed laser at a specific wavelength, was effective but was associated with many adverse reactions. PDT using porfimer sodium as the chemical sensitizer resulted in deep injury in some cases, with a resulting high stricture rate. Despite this, on follow-up endoscopic biopsies Barrett’s glands were detected in some patients underneath the neosquamous epithelium.
Buried Barrett’s Glands Before Ablation Therapy
Further analysis has confirmed that such “buried” glands occur even before ablation therapy, at the Z-line (squamocolumnar junction), and are related to squamous islands within the Barrett’s segment. Buried glands that present after ablation are of unclear clinical significance; however, particularly in patients who have been treated for HGD, buried glands after therapy may contain or develop dysplasia or adenocarcinoma.
Argon Plasma Coagulation
Argon plasma coagulation (APC), widely available in modern endoscopy units, has been used for the ablation of Barrett’s mucosa. However, the depth of ablation is variable, and an even ablation depth is difficult to achieve, particularly in larger segments. Buried glands and recurrent dysplasia and cancer have been reported following APC ablation of Barrett’s esophagus.
Radiofrequency ablation in Barrett’s esophagus
The current standard for ablation of Barrett’s esophagus is radiofrequency ablation (RFA). RFA for Barrett’s esophagus consists of a radiofrequency energy waveform delivered on contact with the targeted epithelium resulting in water vaporization, coagulation of proteins, and cell necrosis. The depth of injury is controlled by the electrode pattern and field geometry, as well as standardization of power density and energy density. Devices are available for circumferential and focal RFA.
RFA Results
Shaheen and colleagues reported on a multicenter randomized, sham-controlled trial in the United States of RFA versus surveillance for dysplastic Barrett’s esophagus including separate patient cohorts having HGD or LGD. In the per-protocol analyses complete eradication of HGD was 89.5%, compared with 20% in the control group, and eradication of LGD was reported as 95.0% in the ablation group compared with 26.3% in the control group ( P <.001). The incidence of any neoplastic progression during the first year of the study was 3.6% in the ablation group, compared with 16.3% in the controls ( P <.05).
RFA treatment was designed to remove Barrett’s mucosa (with an emphasis on safety) just to the top of the submucosa. Transient chest pain and odynophagia are common after RFA, but in the 84 patients with dysplasia in this randomized controlled trial there was only 1 case of significant gastrointestinal bleeding and 3 cases of easily dilated esophageal strictures.
Surveillance After RFA for Barrett’s Esophagus
In an ongoing follow-up of the same cohorts including patients in the control group crossed over to treatment after 1 year, more than 85% of patients remained free of dysplasia, and more than 75% were free of intestinal metaplasia at 3 years. More than 90% of patients were free of dysplasia and intestinal metaplasia after a single additional treatment.
In another study, Pouw and colleagues showed a reversion of abnormal molecular markers in dysplastic Barrett’s esophagus to normal in the neosquamous mucosa after RFA. With all this benefit, however, on further surveillance 7% to 32% of patients successfully ablated with RFA will over time be found to have intestinal metaplasia on endoscopic biopsies. The great majority of these will be at the new squamocolumnar junction or within a few centimeters of the junction. Long-segment Barrett’s esophagus and ablation performed in patients with HGD and intramucosal carcinoma have been identified as having a higher risk for recurrence of intestinal metaplasia. Recurrent dysplasia and adenocarcinoma have also been reported, almost all in patients who had dysplasia or intramucosal cancer before ablation. This phenomenon has once again raised the question of the significance of buried Barrett’s glands.
Buried Glands Before and After RFA
In a controlled study of RFA for Barrett’s dysplasia, a systematic review of all biopsy specimens before and after RFA was performed by the central pathology laboratory. Before ablation, buried glands were found to be common, and were a nearly identical (25% and 26%, respectively) in the treatment and control groups. Following the 1-year RFA protocol, the buried glands in the treatment group decreased to 5%, whereas the rate in the control group increased to 40% with the additional biopsies. In this rigorous analysis, clearly RFA was reducing, but not completely eliminating, buried Barrett’s glands.
OCT for Detection of Buried Glands After RFA
In 2009, Adler and colleagues published a case report detailing the use of 3-dimensional OCT in a patient with Barrett’s esophagus following RFA. Buried Barrett’s glands were identified on OCT beneath neosquamous mucosa. Challenged by a Letter to the Editor that histologic confirmation was inconclusive and that OCT might not be relevant in postablation patients, the investigators pointed out the much larger area sampled by OCT compared with the 4-mm 2 area sampled by a biopsy; they pointed out that buried biopsy glands usually occur in a sparse distribution, likely to be missed by biopsy sampling error.
An ex vivo study by Cobb and colleagues in 2009 supported the potential for OCT to detect buried Barrett’s glands after ablation. The investigators conducted 2-dimensional OCT on esophagectomy specimens from 14 patients who underwent surgery for HGD or adenocarcinoma in Barrett’s esophagus. Buried Barrett’s glands in these ablation-naïve patients on OCT corresponded to histology. OCT showed buried glands in 10 of 14 patients, which were not detected by prior endoscopic biopsy.
In 2012, Zhou and colleagues studied patients with 3-dimensional OCT at various stages of RFA treatment, evaluating the presence of buried glands in the area of the esophagogastric junction. A total of 18 patients were examined before they had complete response of intestinal metaplasia (CR-IM), and 16 patients were examined after CR-IM on endoscopy. OCT showed buried glands in 13 of 18 patients in the pre–CE-IM group and 10 of 16 patients in the post–CR-IM group. The number (mean [standard deviation]) of buried glands per patient in the post–CE-IM group (7.1 [9.3]) was significantly lower than that in the pre–CE-IM group (34.4 [44.6]; P = .02). The investigators concluded that while buried glands were markedly reduced by RFA, their continued presence at the esophagogastric junction could be localized with OCT for possible additional therapy.
OCT for Predicting Incomplete RFA
In a second article from this group in 2012, Tsai and colleagues published a report evaluating 33 patients with 3-dimensional OCT. The patients had short-segment Barrett’s esophagus (<3 cm in length). OCT was performed at the esophagogastric junction immediately before and after RFA treatment using the focal RFA device. Using OCT, the investigators measured the thickness of the Barrett’s mucosa before treatment and looked for evidence of residual Barrett’s glands immediately after completion of the ablation. After 6 to 8 weeks the patients were examined again with standard endoscopy, and biopsies were performed if there was no endoscopic evidence of residual Barrett’s esophagus.
Results showed that the Barrett’s mucosa was significantly thinner in patients who achieved complete eradication of intestinal metaplasia (257 ± 60 μm) than in patients who did not achieve complete eradication of intestinal metaplasia (403 ± 86 μm) on biopsy 6 to 8 weeks after an initial focal RFA ( P <.0001). A threshold thickness of 333 μm (derived from receiver-operating characteristic curves) corresponded to sensitivity of 93.3%, specificity of 85%, and accuracy of 87.9% in predicting the presence of Barrett’s glands on follow-up biopsy. The presence of Barrett’s glands on OCT imaging immediately after ablation also correlated with the finding of Barrett’s glands on follow-up (sensitivity 83.3%, specificity 95%, accuracy 90.6%). The investigators postulated that OCT might be able to guide RFA by suggesting more aggressive treatment in selected patients based on the pretreatment thickness of the Barrett’s mucosa or the detection of residual Barrett’s glands immediately after treatment.
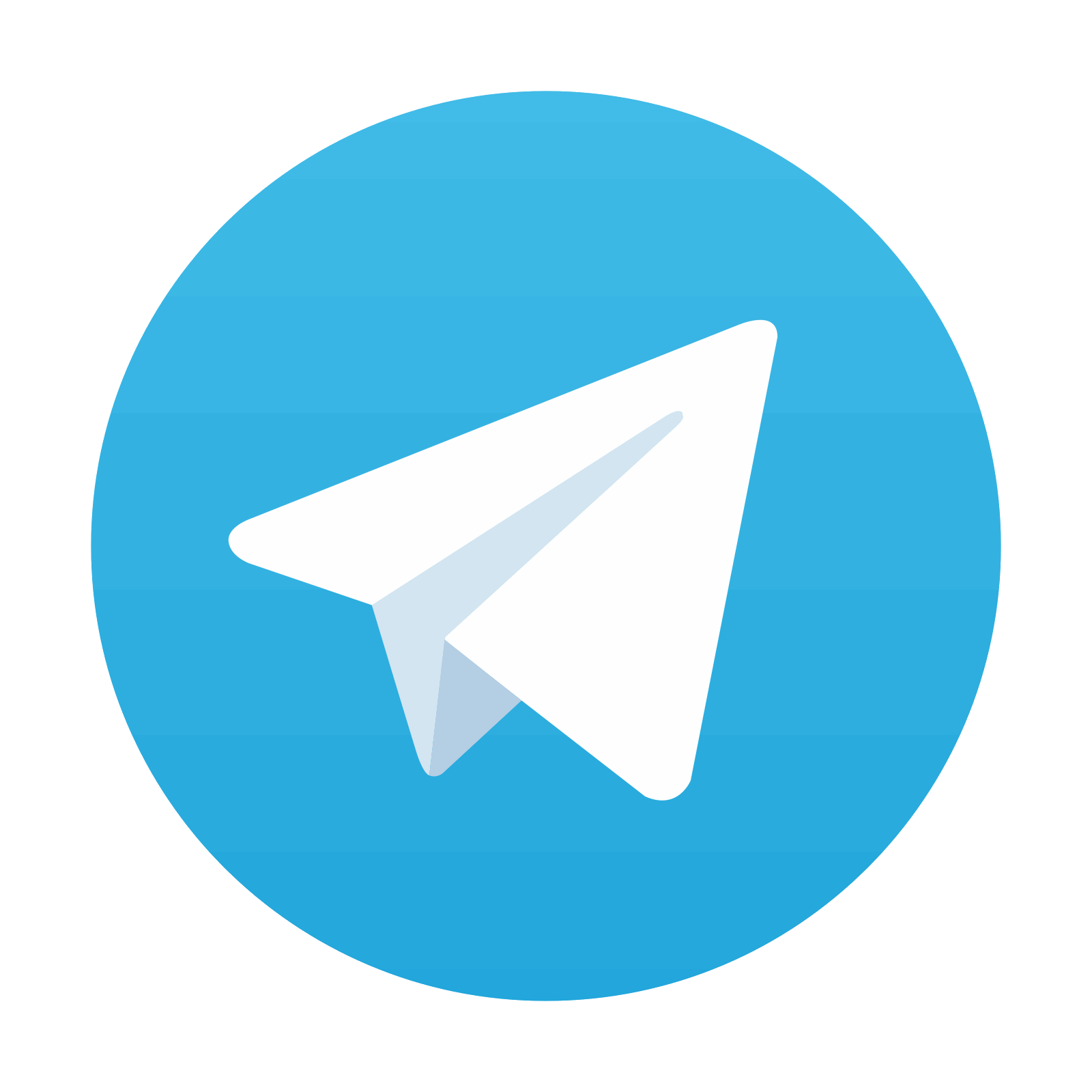
Stay updated, free articles. Join our Telegram channel

Full access? Get Clinical Tree
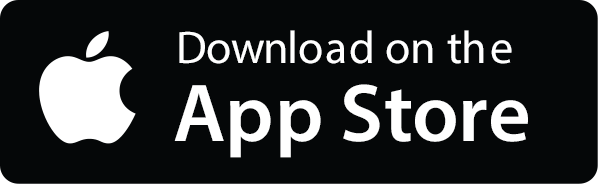
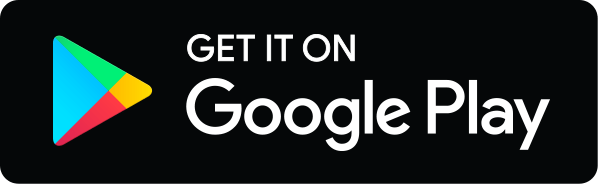