div class=”ChapterContextInformation”>
6. Treatment of Storage LUTS
Keywords
Conservative measuresBehavioural modificationAntimuscarinicsBeat-3 agonistPelvic floor muscle trainingBladder trainingBiofeedbackOnabotulinum neurotoxin-ASacral nerve stimulationTibial nerve stimulationColposuspensionMidurethral tapeAutologous fascial slingMixed LUTSContinence products6.1 Introduction
Conservative treatment strategies are an accepted option in patients with storage LUTS in both men and women. Conservative management is defined as any therapy that does not involve either pharmacological or surgical intervention [1]. It is often viewed as the first-line treatment for men and women with stress or urgency incontinence, beginning initially in a primary care setting and continued in patients awaiting or unsuitable for surgery.
As an alternative to the long-established antimuscarinic drugs for overactive bladder, beta-3 adrenergic receptor (adrenoceptor) agonists were shown effective in several animal models of overactive bladder (OAB). Two have progressed from laboratory testing to clinical trials—mirabegron and solabegron [2]. The only beta-3 agonist available in clinical practice for the treatment of OAB syndrome is mirabegron. It was approved in Europe, in 2013, after it was proven effective in improving symptoms of urgency, increased frequency and urgency urinary incontinence (UUI) [3]. It has become a popular alternative to antimuscarinics, but its place in the treatment of OAB, among other drugs, remains undetermined [4]. Some potential targets have been identified for future drugs. While these include members of the transient receptor potential (TRP) channel family, members of the Degenerin/Epithelial Na+ (Deg/ENaC) channel family and Big Potassium (BK) channels, the reality of converting any of these into an available medication is a long way off.
Once medications have proved insufficient (‘refractory OAB’), sacral nerve stimulation, tibial nerve stimulation and botulinum injections each have their advocates.
If pelvic floor muscle training proves inadequate in people with stress incontinence, the main interventions are surgical, both for men and women. These can be combined with the OAB therapies for people suffering from mixed urinary incontinence.
Reconstructive urological surgery has four major goals: to increase life expectancy, maintain or improve renal function, maintain or obtain urinary continence and preserve or improve sexual function. Maintenance of renal function is thus one of the most important objectives to be weighted against the morbidity of genitourinary reconstructive procedures. In this regard the surgeon should always balance the ability to provide urinary continence with the risk of losing renal function. Whether performed at a young or older age, families must be adequately counselled regarding the daily management responsibilities and potential risks associated with lower urinary tract reconstruction, including bladder augmentation.
Everyone with urinary incontinence (UI) and/or faecal incontinence (FI) would like to be cured, but complete cure is not always possible. Then the challenge is to discover how to manage any unresolved leakage such as to minimize its impact on quality of life, and this usually involves using continence products to prevent or to contain leakage. Even those whose incontinence is ultimately treated successfully may have to live with symptoms for a time (for example, while they wait for surgery, or for pelvic floor muscle training to yield its benefits), and thus they may use continence products temporarily. Others may use them as an adjunct to a treatment that reduces their leakage without eliminating it. There is a bewildering variety of products on the market and very few of them have been subjected to published trials, so that identifying those most appropriate to a particular need can be challenging. However, the plethora of products fall into relatively few major design categories, and findings from a trial of one specific product can often be usefully extrapolated to others in the category; that is, useful general principles may be drafted from limited data.
6.2 Conservative Measures in Men and Women
6.2.1 Lifestyle Changes/Behaviour Modification
- a.
Alter fluid intake (decrease to reduce incontinence and frequency, increase to improve urine concentration)
- b.
Modify the diet to eliminate bladder irritants (reducing caffeine, alcohol, carbonated drinks)
- c.
Regulation of bowel function to avoid constipation
- d.
Cessation of smoking
- e.
Weight control
Adequate fluid intake is required to eliminate irritants from the bladder. Under-hydration decreases the functional capacity of the bladder and may play a role in the development of UTIs, while excessive fluid intake can trigger incontinence and symptoms that could be misinterpreted as OAB (i.e. increased daytime frequency, nocturia and persisting desire to void). Fluid intake should be regulated to 6- to 8-ounce glasses or 30 cc/kg body weight per day, with a 1500 mL/day minimum—unless contraindicated by a prior medical condition [6]. Aging is associated with an increase in nocturia and chronic medical conditions (notably congestive cardiac failure, obstructive sleep apnoea and diuretic use); along with evening/night-time fluid consumption, these are all causes of nocturnal polyuria. Patients who develop oedema of the lower extremities may elevate their legs on a stool during the day to stimulate natural diuresis in the evening and hence reduce the amount of voiding during the night—though in reality, the difference this actually makes tends to be marginal. The patient should be advised to reduce his/her fluid intake after 6 pm (or approximately 4 h before usual bedtime) and shift the intake towards the morning and afternoon, to decrease the nocturia precipitated by fluid intake.
The consumption of caffeine-containing beverages , foods and medications are felt to have an impact, as the caffeine causes a significant bladder response, leading to storage symptoms. Alcohol provides a diuretic effect and its use can increase urinary frequency—if taken during evening hours, this may contribute to nocturia. It is therefore recommended that patients eliminate the possible products on a one-by-one basis to assess if symptoms decrease or resolve. Patients with storage LUTS and incontinence should avoid excessive caffeine intake (e.g. no more than 200 mg/day, equivalent to 2 cups) as it has been shown to reduce urinary symptoms and urine leakage [7].
Studies of women who have suffered severe chronic constipation (defined as less than three stools per week) and straining during defecation may manifest a change in pelvic floor neurological function relevant to storage LUTS, UI and OAB. Self-care practices that promote bowel regularity should be an integral part of a treatment care plan, such as addition of dietary fibre, increased fluid intake (which has to be balanced against the points above), regular exercise and external stimulation [8].
Smoking indirectly increases the incidence of urinary urgency and UI due to its effect on coughing and development of associated co-morbid conditions [9]. Coughing leads to increased intra-abdominal pressure and subsequent downward pressure on the pelvic floor, causing likely repeated stretch injury to the muscle and innervation. In conjunction, the effects of nicotine and tobacco products (potentially having possible anti-oestrogenic hormonal effects) may play a role in the worsening of individual symptoms.
The link between obesity and stress UI (SUI) and mixed UI appears to be multifactorial. Increases in intra-abdominal pressure on the bladder due to central obesity, as well as greater urethral mobility, appear to play a role in SUI. Furthermore, weight gain may impair blood flow and innervation to the bladder. Accordingly, weight loss is advocated in the treatment of UI.
6.2.2 Bladder Retraining
Several techniques are employed to improve symptoms and decrease rates of UI. The Cochrane Collaboration have reviewed publications for toileting programmes including habit training, timed voiding and prompted voiding. Most of the systematic reviews were based on the context of elderly patients within a care-home setting [6]. Bladder retraining has 3 main outcomes: (1) Improved bladder overactivity by controlling urgency and decreasing frequency; (2) Increased bladder capacity and (3) Reduced urgency incontinence episodes. It is felt that retraining improves cortical inhibition of detrusor contractions, facilitates cortical reinforcement of urethral closure during bladder filling, strengthens pelvic striated muscles and alters behaviours that affect continence [10].
The bladder training programme is done mainly on an outpatient basis. The initial voiding interval is determined by a pre-completed bladder diary, normally beginning with only a short deferral of voiding (a few minutes) and only during daytime hours, and steadily increasing the intervals in small realistic increments. The goal is for the patient to learn to inhibit urgency sensations by resisting or relaxing when they first start to get the sensation. Methods to resist or inhibit the urgency sensation include slow deep breathing to consciously relax the bladder, or six deliberate pelvic muscle contractions of 2–3 seconds duration. The final goal is that the patient will develop a normal voiding pattern [11], or (more likely) ability to reach voiding frequency and urgency severity that does not dominate their everyday life.
6.2.3 Pelvic Floor Muscle Training (PFMT)
There are two types of striated skeletal fibres that make up the pelvic floor muscles (PFM) which are under voluntary control and respond to the prescribed exercise regime. Type I (80%) are slow-twitch muscle fibres which improve muscle endurance by generating a slow, sustained but less-intense contraction to maintain a general level of pelvic support and urethral closure pressure. Type II (20%) are fast-twitch fibres which cause strong and forceful muscle contractions, build pelvic floor muscle strength and help in times of sudden increases in intra-abdominal pressure by contributing to urethral closure. The role of PFMT is to develop the technique of producing a voluntary pelvic floor contraction which will help to increase pressure in the urethra, inhibit detrusor contraction and control leakage of urine. The proposed mechanism of action is threefold: (a) increased urethral closure pressure; (b) lifting of the endopelvic fascia towards the pubic symphysis due to mechanical pressure increase and (c) a pelvic muscle ‘reflex’ contraction that precedes increased bladder pressure and may inhibit OAB [12].
- 1.
Strength: the maximum contraction that a muscle can generate
- 2.
Power: the ability of the muscle to contract as strongly as possible.
- 3.
Endurance: the muscle contraction is timed until it fatigues, up to 10 seconds
- 4.
Repetitions: the number of times a muscle can contract at equal force can be repeated. There must be a 5-second muscle relaxation interval between each contraction without permitting excessive rest periods for the strong muscles.
- 5.
Fatigue: failure to maintain the required force of the PFM contraction for more than one or two times in succession.
Clinicians and specialist physiotherapists should assess the tone of the PFM by vaginal examination. Low muscle tone is the impaired ability to isolate and contract the PFM in the presence of atrophic, weak muscles, while high muscle tone refers to hypertonic, spastic PFM with impairment of muscle isolation, contraction and relaxation [14]. They then need to provide specific instructions on the location of the PFM, and how to focus on contracting it specifically [15], checking by physical examination that the patient has comprehended the instruction and can do it. The type of instruction used can be exemplified by: ‘Try to imagine the control of passing gas or pinching off a stool by tightening the ring of muscles around the anus without tensing the additional muscles of the legs, buttocks or abdomen. A closing and lifting sensation should be felt’.

Example instructions for PFMT
The long-term efficacy of PFMT ranges from 48 to 81% improvement rates [11].
A drawback, and perhaps the reason for hesitation on the part of the public, is that PFMT and other behavioural changes take time and effort—as many as 16 weeks for initial improvements and a lifelong commitment to ongoing maintenance. In addition, ineffective self-management can result in lack of improvement and frustration. Therapies and prevention technologies that provide feedback (i.e. biofeedback) can help women understand whether they are doing PFMT correctly.
6.2.4 Biofeedback
Biofeedback is the technique by which information regarding PFM contractions and relaxations is displayed in a format intuitively understandable to the patient, so they can adjust muscle action to gain confident appreciation of the activity. It uses either electromyography (EMG) or manometric pressure measurement. A common error in contraction of the PFM is collateral recruitment, when an additional (non-relevant) muscle group is contracting at the same time, which may in turn mask the strength of the PFM contraction. For example, it is very common for women to contract the gluteal muscles when trying to contract the PFM. Ongoing biofeedback visits are necessary until recruitment no longer happens.
There are different methods of EMG measurement which have been used: vaginal sensor, anal sensor, surface skin electrodes and needle electrodes. The baseline and follow-up EMG recordings measure short/quick contractions and sustained muscle contractions, as well as the relaxation phase. The ability to relax following a PFM contraction is important to gain control and co-ordination of the muscles [16].
- a.
Digital (vaginal or rectal)—PFM assessment of the levator ani muscle is a form of biofeedback. The effect of PFMT is dependent on whether the contraction/relaxation exercises are performed correctly.
- b.
Verbal feedback enhances patient effort and can be palpated in the 4 and 8 o’clock positions, immediately superior to the hymenal ring.
- c.
Manometric/EMG biofeedback allows PFM awareness, a greater feeling of control, and progress monitoring of the treatment plan.
6.3 Antimuscarinics for OAB
The muscarinic receptors found in the human detrusor are of the M2 and M3 subtypes. They appear to receive background stimulation during the storage phase resulting from a very low-level release of acetylcholine, either from nerve terminals or from the urothelium. This seems to have an effect on smooth muscle or nerve endings that can increase sensory nerve activity or smooth muscle micromotions. The clinical medications counteract the low levels of ACh present in the storage phase, and do not generally affect voiding because the detrusor contraction is driven by a substantial release of ACh direct at the muscle location, easily exceeding the antagonistic effect of a clinical dose of OAB therapy. There are several such drugs, which have been widely tested in randomized controlled trials and shown to be effective in the treatment of urgency incontinence [17]. They generally cause a 50% reduction in incontinence episodes compared to placebo [18]. When initiating the treatment, it is important to check the patient has complied with first-line measures (fluid advice and bladder training), since the antimuscarinics cannot counteract behavioural factors like high caffeine intake. Use of extended-release formulations is preferable in terms of the balance between efficacy and adverse events, cost-effectiveness, adherence and persistence with treatment [19]. Response tends to be rather individualized, so it may be necessary to work on dose and agent selection in order to find one which suits the patient in balancing efficacy against side effects. The side effects mean that antimuscarinics may be poorly tolerated [20]. This evidently translates into low levels of long-term use [21]. They may also cause concern due to a potential impact on M1 receptors, which are important in cognition. The overall anticholinergic burden of prescribed medications has led to awareness of impact on dementia [22] and often results in reluctance of patients to take them for OAB treatment. There is also a decline in antimuscarinic use now that beta-3 agonist is available, as mirabegron 50 mg appears to be at least as effective as most antimuscarinics, but with an incidence of dry mouth similar to placebo [23].
Common side effects result from effects on muscarinic receptors elsewhere, resulting in reduced salivation, dry skin, constipation, blurred vision and dry eyes. Cardiovascular effects include palpitations. Gastrointestinal disturbances can include constipation, flatulence and taste disturbances. Thus, they are contraindicated in ulcerative colitis, toxic megacolon and in gastrointestinal obstruction. They also are contraindicated in poorly controlled angle-closure glaucoma, severe gastro-oesophageal reflux and myasthenia gravis. In angle-closure glaucoma they cause papillary dilatation, hence obstructing outflow of aqueous humour. They can safely be used in open-angle glaucoma or angle-closure glaucoma that has been treated with iridotomy. Myasthenia gravis is an autoimmune neuromuscular disease caused by circulating antibodies that block acetylcholine receptors at the post-synaptic neuromuscular junction. Myasthenia is treated medically with cholinesterase inhibitors or immunosuppression. Antimuscarinics for OAB are contraindicated because they compete with ACh for a common binding site on the receptor, hence further reducing receptor activation and muscle contraction.
6.4 Beta-3 Adrenergic Agonist for OAB
Mirabegron is a potent, selective beta-3 agonist [24]. After oral administration, mirabegron’s time to maximum plasma concentration is approximately 3–5 h, and its plasma half-life is roughly 40 h [25], reaching a steady state after 7 days of treatment [26]. Its oral bioavailability ranges between 24 and 53% and is not significantly altered by food intake [27]. Mirabegron is cleared by several mechanisms, including renal and biliary excretion, mostly as the unchanged form, and also as metabolites arising from the action of cytochrome P450 and other enzymes, in the liver [28]. Therefore, it is not recommended to use mirabegron in individuals with severe kidney or liver disease, nor in those who are on potent cytochrome P450 inhibitors (mostly 3A4 and 2D6 isoforms). Furthermore, daily doses of 25 mg should not be exceeded in individuals whose glomerular filtration rate lies between 15 and 30 mL/min or in those with chronic liver disease (Child-Pugh B or worse). The patient’s age does not seem to affect mirabegron’s pharmacokinetics [25], but women reach higher plasma concentration than men due to different body composition [29].
The clinical efficacy of mirabegron on OAB symptoms has been assessed in several clinical trials. It significantly reduces urgency urinary incontinence (UUI) episodes, urgency, increased frequency and nocturia [3, 30, 31]. In Europe, the licenced starting dose is 50 mg, with a 25-mg preparation available for patients with moderate chronic liver or kidney disease. In the USA, the required starting dose is 25 mg, with the possibility to increase it to 50 mg. In a large meta-analysis with over 10,000 patients, mirabegron 50 mg led to an adjusted mean reduction from baseline of UUI episodes per 24 h of 1.25, and of urgency episodes per 24 h of 1.9; similarly, there was a reduction in the number of micturitions per 24 h of 1.7, including a reduction of nocturia episodes of 0.5, and an increment of voided volume by 20.8 mL per micturition; these variations are significantly more pronounced than placebo-induced changes in OAB symptoms [32].
The clinical benefit of mirabegron appears to be greater for individuals with more severe symptoms [33]. Furthermore, these improvements seem to be sustained and long-lasting, regardless of previous antimuscarinic treatment [34]. It is also important to notice that there is a direct correlation between objective and patient-reported outcome measures [35], meaning that improvements in objective measures translate into real benefits in patients’ quality of life. Antimuscarinics and mirabegron have shown similar efficacy in reducing OAB symptoms, with full continence (“dry rates”) of up to 50% [23, 36]. In a large meta-analysis, comparing mirabegron to placebo and several antimuscarinics, the efficacy of mirabegron 50 mg was significantly higher than placebo for all endpoints [37]; concerning increased frequency, it did not contrast significantly with other active treatments other than solifenacin 10 mg; regarding UUI, mirabegron 50 mg was outperformed by fesoterodine 8 mg; as for dry rates, trospium 60 mg, solifenacin 10 mg and fesoterodine 8 mg were more efficacious than mirabegron 50 mg; moreover, solifenacin 5 mg was better at achieving a 50% reduction in UUI episodes. However, mirabegron 50 mg may provide a better efficacy/tolerability relation compared to antimuscarinics, which could explain the higher adherence of mirabegron over antimuscarinics [38]. In fact, after one year of treatment, the adherence rate for mirabegron is about 2/5 versus about 1/5 for antimuscarinics, and median time to discontinuation is around 5.6 and 1.9 months, respectively [39, 40]. Furthermore, recent data suggests that mirabegron might be more cost-effective than tolterodine in first-line therapy, excluding the most severe OAB cases [41].
Adding mirabegron 50 mg in patients with insufficient clinical response to solifenacin 5 mg leads to a higher probability of meaningful symptomatic improvement without decreased tolerability, as compared to either monotherapy and dose escalation of antimuscarinics [37, 42–45]. Combined therapy produces consistent symptomatic improvements compared to monotherapy by week 4, in an additive rather than synergistic manner [46]. This may be an appropriate option for patients unwilling or unsuitable for more invasive treatments.
The most common adverse effects of mirabegron are hypertension (7%), nasopharyngitis (3%) and urinary tract infections (3%) [30]. There is no evidence of a dose-dependent incidence of adverse effects [32]. The tolerability of mirabegron seems to be higher in females, the elderly, and those without UUI—an explanation for these findings is still lacking [38].
The cardiovascular profile of mirabegron is comparable with that of antimuscarinics [47], despite the expression of beta-3 adrenoceptors in cardiovascular tissues. Moreover, there is no significant interaction of mirabegron with beta-blockers in clinical practice, regarding their efficacy and adverse effects [47]. In healthy individuals, mirabegron does not cause QTc prolongation, and neither does it interfere with blood pressure and heart rate in a clinically significant manner [32]. Nevertheless, mirabegron is contraindicated in patients with severe uncontrolled systemic hypertension (systolic blood pressure >180 mmHg or diastolic blood pressure >110 mmHg).
Compared to antimuscarinics, the incidence of urinary retention, xerostomia and other gastrointestinal disorders is significantly lower during treatment with mirabegron [34, 38]. Additionally, there is no concern regarding possible cognitive impairment as for antimuscarinics. Overall, these factors can translate into better quality of life for patients and consequently higher treatment adherence and persistence. Mirabegron does not worsen voiding urodynamic parameters in men with combined OAB and bladder outlet obstruction compared to placebo [48]. Therefore, it does not seem to impair bladder contractility during voiding, despite its relaxing effect during filling.
Current evidence shows that, comparing to antimuscarinics, mirabegron 50 mg is equally effective in reducing OAB symptoms with more tolerable side effects. Further investigation should help tailor the pharmacological treatment of different OAB subsets and assess the efficacy and safety of combination regimens. Future development of other beta-3 agonists will expectedly broaden the armamentarium of OAB management.
6.5 Novel Treatment Targets for Storage LUTS
6.5.1 TRP Channels
Cation channels of the TRP (Transient Receptor Potential) superfamily can be considered as universal cellular sensors, due to their ability to respond to a wide range of local signals, including physical stimuli (temperature, stretch) and a variety of endogenous and exogenous chemical ligands [2, 3]. In recent years, research using TRP channel-deficient mice and specific TRP channel (ant)agonists has revealed that several members of the TRP superfamily can act as sensors in the urothelium and bladder wall [2, 4, 17–19]. For example, TRPV1 (vanilloid 1) and TRPV4 (vanilloid 4) have been implicated in sensing bladder stretch [4, 17, 20], TRPV1 and TRPA1 (ankyrin 1) may act as sensors of chemical irritants and TRPM8 (melastatin 8), known as a cold sensor in the skin, is implicated in acute cold-induced urinary urgency [21]. Moreover, altered TRP channel expression and function have been linked to bladder dysfunction in various animal models of bladder disease [22]. These findings raise the possibility that TRP channels may be targeted pharmacologically to modulate the sensory function of urothelium and bladder wall, and could thereby alleviate storage LUTS in some patients. However, confining the drug effects to the lower urinary tract is a challenge that considerably limits their development for this indication.
The only clinical application of TRP modulation used in patients with LUTS so far is the well-characterized intravesical TRPV1 desensitization using capsaicin or the 1000-fold more potent TRPV1-agonist resiniferatoxin [23, 24]. Intravesical resiniferatoxin instillation inactivates bladder afferent C fibres, resulting in reduced bladder pain in patients with bladder pain syndrome and increased maximum cystometric capacity in patients with detrusor overactivity [24]. These are not used in current practice but provide a valuable point of principle.
Other potential therapeutic targets for treating storage LUTS include TRPV4 and TRPM8, which are both expressed in the urothelium, and TRPV4 also is present in detrusor smooth muscle. Mice lacking TRPV4 exhibit a decrease in voiding frequency, with increased non-voiding contractions, and systemic administration of the TRPV4 antagonist HC-067047 also results in decreased voiding frequency with larger bladder capacities [4, 25]. The latter effect is seen both in healthy mice and in mice with cyclophosphamide-induced cystitis [4]. This makes TRPV4 antagonist a putative clinical candidate, but side effects would limit the dosing due to a widespread expression of TRPV4 in other organs (notably the nervous system, vascular endothelium and airway epithelium) [26].
Patients with idiopathic detrusor overactivity have an increase in density of suburothelial TRPM8-immunoreactive nerve fibres, and this density correlates with frequency scores [27]. Administration of a TRPM8-antagonist increases bladder capacity in rats [28]. Use of TRPM8 antagonist to treat increased frequency and (cold-exacerbated) urgency should be investigated further [18].
6.5.2 Degenerin/Epithelial Na+ Channels
The Degenerin/Epithelial sodium channels (Deg/ENaC) are mechanosensitive ion channels, and previous research has shown that they play a key role in the mechanosensory signalling of the bladder [29]. This cationic channel family, which was discovered in the 1990s, is characterized by amiloride sensitivity. They can be activated by either ligands and/or mechanical stimuli, or they may be constitutively active. The Deg/ENaC channels are divided into subfamilies and can be found in both vertebrates and non-vertebrates. In mammals, the following three groups are described: Epithelial Na channel (ENaC), acid-sensing ion channels 5-(ASIC) and brain–liver–intestine sodium channel/human intestine sodium channel (BLINaC/hINaC) [30]. One can notice some similarities when comparing the general characteristics of the DEG/ENaC family to the TRP channel family.
The ENaCs were first described to play a crucial role in sodium absorption in a wide variety of epithelia, such as secretory glands, respiratory airways, kidney, distal colon and peripheral nervous system [29]. They are expressed in the mammalian urinary tract epithelia and suburothelial nerve fibres; in the bladder epithelium, they are implicated in mechanosensory transduction by controlling stretch-evoked ATP release. Therefore, the Degenerin/Epithelial sodium channels are a potential pharmacological target for storage LUTS.
Proving this hypothesis appeared rather challenging. The in vivo research was conducted mostly on animals. However, it has been shown that there is a marked difference in expression level of ENaCs in human bladder under normal conditions versus the rodent bladder. Furthermore, these very low expression levels in humans under normal conditions seemed to be subject to changes in pathological situations. For example, a marked upregulation of ENaC expression was shown when comparing tissue from bladder epithelium of patients with detrusor overactivity caused by bladder outlet obstruction versus healthy controls. The research group concluded that ENaCs might be implicated in the mechanosensory transduction in the bladder afferent pathways [31]. Another group found that the expression of ENaCs and ASICs was highly upregulated in spinal cord injury patients with detrusor overactivity. They even conclude that this expression level could correlate significantly with their storage urinary symptoms [32]. Needless to say, these are very strong conclusions based on small populations, and this variance in expression complicates the implementation of a generalized disease model.
Another path that has been investigated are the ASICs, a subgroup of neuronal ENaCs. They were reported to be highly expressed in the urothelium and suburothelial nerve plexus. Upregulation of these channels or an increase in intra-bladder pressure during bladder filling may trigger afferent signalling [33]. They may be involved in nociception in various pathological conditions such as bladder pain syndrome and bladder inflammation [34]. Consequently, multiple research groups have defined ENaC/ASIC ion channels to be possible novel targets for the pharmacological treatment of inflammatory and overactive bladder conditions [29, 35].
To our knowledge, to this date, no large clinical trials have been reported in humans testing experimental drugs targeting members of the Deg/ENaC family. Some research teams are focusing on the effect of amiloride, an antagonist of ENaCs. One group has found that the induction of voiding frequency caused by fludrocortisone could be reversed by giving an intravesical infusion of amiloride. They conclude that upregulation of ENaC could play a role in storage dysfunction [36]. Furthermore, the antagonist amiloride was also shown to attenuate pain, induced by an intradermal acid infusion, but did not inhibit pain caused by capsaicin (TRPV1 agonist). This was investigated more extensively than the effect on bladder dysfunction, but due to some conflicting results and an incomplete physiological knowledge of ASICs, until now no drugs are developed and clinically tested [37].
6.5.3 Big Potassium Channels
Relaxation and contraction of the detrusor muscle allows the bladder to store urine or empty the bladder at appropriate times. In detrusor smooth muscle cells, three major subtypes of potassium channels are represented. These channels play a key role in maintaining the resting membrane potential and action potentials of the detrusor muscle. One of the most pathologically and physiologically important potassium channels are the large-conductance voltage- and Ca2+-activated K+ (BK) channels [38]. These so-called Big Potassium channels are widely expressed in mammalian tissues and cell types such as neurons, smooth and skeletal muscle and exocrine cells. They are positioned on membrane surfaces such as the plasma membrane, mitochondrial membranes and the nuclear membrane [39]. As these channels can be activated by both Ca2+ and membrane depolarization, they have the ability to integrate changes in intracellular calcium and membrane potential. This makes the BK channels an important negative feedback system to limit membrane depolarization and intracellular Ca2+ concentrations. Therefore, they are essential for the control of detrusor smooth muscle cell tone and detrusor contraction [40, 41]. For this reason, BK channels and their regulatory mechanisms could serve as novel targets for pharmacotherapeutic interventions or genetic therapies for OAB [42]. Thus far, three approaches to modulate BK channels have been explored. These include the use of BK channel openers, modulation of BK channel regulatory mechanisms and BK channel gene therapy.
Extensive in vitro and in vivo research in both animals and humans shows that positive modulation of BK channels by small molecules, the so-called channel openers, can reduce detrusor contractility without compromising normal voiding functions [41]. These BK channel openers have a certain selectivity for smooth muscle cell BK channels. As these BK channels, however, are also expressed in other smooth muscle cells, selective BK channel openers may, for example, also have vascular effects. Consequently, further investigation concerning new BK openers with improved selectivity is needed [38]. BK channel regulatory mechanisms provide another approach to targeting BK channels. One example of this approach is the selective β3-agonist mirabegron, which was successfully introduced into clinical practice in 2012. Other potential BK channel regulatory mechanism targets are PDE1 and PDE4. These enzymes have an essential part in regulation of cellular cAMP levels in detrusor smooth muscle cells. Modulation of these regulatory mechanisms, instead of direct activation of BK channels by selective BK channel openers, could be a better option for treatment of storage LUTS with possibly lower risk of adverse effects [38]. BK channel gene therapy is another potential therapeutic approach for LUTS treatment. According to the FDA, human gene therapy seeks to modify or manipulate the expression of a gene or to alter the biological properties of living cells for therapeutic use. A phase I multicentre study reported that the recombinant BK channel α-subunit gene can be directly and locally instilled in the human bladder to create a detrusor muscle cell-specific, long-lasting effect without significant transfer-related side effects. This transfer, however, needs to be repeated every 6 months, as the DNA does not integrate into the chromosome of the cells [38]. Another perspective is that BK channels may play an important role in bladder afferent nerve activity and urothelial function [41]. Unlike the role of BK channels in the detrusor smooth muscle cells, the physiological function of BK channels in the urothelium is still being investigated. A recent study, however, confirmed the presence of BK channels on mouse urothelial umbrella cells [43].
We can conclude that the BK channel embodies a promising opportunity for pharmacotherapeutic treatment of storage LUTS. The pathophysiological role of these channels in regulation of detrusor smooth muscle cells, however, needs to be understood better to better correlate basic research with clinical practice.
6.6 Botulinum Toxin A in Idiopathic OAB
Botulinum Toxin type A (BoNT/A) is, among the seven serotypes produced by Clostridium botulinum, the most relevant for clinical application. BoNT/A consists of a heavy and a light chain linked by a disulphide bond. The heavy chain is responsible for the recognition of specific neuronal regions. The light chain is the one that has enzymatic properties. Once internalized into the cytosol, it inactivates the membrane proteins involved in fusion of synaptic vesicles with the cytoplasmatic membrane, thereby impairing neurotransmitter release [49].
There are at least four different commercial forms of BoNT/A: Botox, Dysport, Xeomin and Prosigne. Although the toxin effect is the same, it is available as a protein complex wrapped by different proteins, which change both the molecular weight and the relative potency of each brand. This issue was the basis for the introduction of non-proprietary names by the FDA: onabotulinum toxin A (onabotA), abobotulinum toxin A (abobotA) and incobotulinum toxin A (incobotA) for Botox®, Dysport® and Xeomin®, respectively. Prosigne is the proprietary name of a BoNT/A produced in China, for which a non-proprietary name is awaited. Although potency of each toxin brand is expressed in apparently similar units (U), the doses are not inter-changeable and conversion ratios between the different brands do not exist. Estimates from studies carried out in skeletal muscle suggest that onabotulinum toxin type A is roughly three times more potent than abobotulinum toxin A and equivalent to incobotulinum toxin A. Nevertheless, these equivalences should be approached with caution, since in the bladder wall of the mice the conversion ratio between onabotulinum toxin and abobotulinum was calculated around 1:1.6 [50].
In the human bladder, parasympathetic, sympathetic and sensory transmission are impaired after onabotulinum toxin A injection [51]. The blockade of acetylcholine (ACh) release from parasympathetic fibres is a key mechanism of the therapeutic benefit, and also the reduced detrusor contractility that follows the toxin injection in the bladder. However, bladder sensory impairment is also likely to play a relevant role in the final effect of onabotulinum toxin A, and the biological mechanism causing this is less certain. There is no evidence that repeated injections of onabotulinum toxin A into the bladder causes inflammation, fibrotic activity or apoptosis of bladder cells [52].
Onabotulinum toxin A intravesical injection was approved for the treatment of neurogenic detrusor overactivity (NDO) and idiopathic overactive bladder (OAB) by both the European Medicines Agency (EMEA) and FDA. The dose of onabotulinum toxin A licenced for the treatment of OAB is 100 U, based on a dose escalating placebo-controlled study and two large phase III placebo-controlled trials [53–55]. The treatment paradigm consists of 20 injections of 0.5 mL each above the trigone, containing 5 U per injection, making a total of 100 U [53, 54]. The two phase III studies enrolled mostly female patients with idiopathic OAB, who had not had sufficient clinical response to anticholinergic medications. Both studies showed a significant decrease in incontinence episodes per day, frequency, urgency episodes and nocturia. Also, clinically meaningful improvements from baseline in all symptom score (I-QOL and KHQ) multi-item domains indicated positive impact on quality of life.
A pooled analysis of the two pivotal phase 3 studies [56] included 1105 patients randomized to onabotulinum toxin A 100 U (n = 557) or placebo (n = 548). In patients submitted to onabotulinum toxin A, the dry rate at week 12 was 27.1%. Furthermore, 60.5% of onabotulinum toxin A-treated patients reported a reduction of ≥50% from baseline in urinary incontinence episodes compared to 31% in the placebo group (p < 0.001). The decrease from baseline in the number of episodes of urinary urgency per day was 3.30 and 1.23 in the onabotulinum toxin A and placebo groups, respectively. The median time to request retreatment was 24 weeks following treatment with onabotulinum toxin A compared to 13 weeks with placebo. The number of previous anticholinergic drugs used does not influence the outcome of onabotulinum toxin A treatment. The effect remains stable across subsequent treatments during a period of 3.5 years [57]. The median duration of effect, derived from the time to retreatment, was 7.6 months.
The most frequent complications of Onabotulinum toxin A injection in the bladder are urinary retention, leading to the necessity of clean intermittent catheterization (CIC), and UTIs. The overall risk of CIC was slightly above 5%. However, it is lower in younger patients and worse in patients above the age of 70. The proportion of patients that required CIC in the first treatment cycle did not show an increased risk in subsequent treatments.
Real-life studies offer contradictory results concerning persistence on an onabotulinum toxin A programme. In a group of 100 patients with refractory OAB, 90% would return to the programme in the future, and 93% would recommend the treatment to a friend; 81% accepted the treatment as lifelong [58].
6.6.1 Comparisons of Onabotulinum Toxin A with Other Treatments
The Anticholinergic Versus Botulinum Toxin A Comparison Trial (ABC trial) [59] randomized 249 women with OAB who had 5 or more episodes of urgency urinary incontinence in a 3-day bladder diary. For a 6-month period, participants were randomly assigned to intradetrusor injection of 100 U of onabotulinum toxin A plus daily oral placebo, or daily oral anticholinergic medication (solifenacin 5 mg initially, with possible escalation to 10 mg and, if necessary, subsequent switch to trospium XR 60 mg) plus intradetrusor injection of saline. Complete continence was reported more commonly in the onabotulinum toxin A than in the anticholinergic group, at 27% vs 13% (p = 0.003), respectively. The toxin group had higher rates of catheter use at 2 months (5% vs. 0%) and UTI (33% vs. 13%) than the anticholinergic group. The latter on the other hand had a higher rate of dry mouth (46% vs. 31%) [59]. In a similar study, researchers randomized patients with OAB and urinary incontinence to receive onabotulinum toxin A 100 U injection plus oral placebo (n = 145), or oral solifenacin plus placebo injection (n = 151) or double placebo (n = 60), for 12 weeks. At week 12, mean reduction from baseline in urinary incontinence episodes/day was significantly greater with either onabotulinum toxin A (−3.2) or solifenacin (−2.6) versus placebo (−1.3; P < 0.001 for both). The proportion of patients with 100% reduction in urinary incontinence episodes at week 12 was 33% for onabotulinum toxin A and 24% for solifenacin [60]. Overall, a metanalysis confirmed that onabotulinum toxin A injections are more effective than any oral therapy (antimuscarinics, beta 3 agonists) to cure urgency incontinence [61].
The Rosetta study compared onabotulinum toxin A 200 U bladder injections against SNM (interStim) in refractory OAB patients [62]. A significantly greater number of patients experienced a complete resolution (20% vs 4%) of UUI episodes with onabotulinum toxin A 200 U treatment than with SNM InterStim at 6 months. However, these differences had faded away at 2 years follow-up. The cure rates were identical, 5% in both groups, although the satisfaction rate remained higher among patients treated with onabotulinum toxin A injections. In the onabotulinum A arm 72% asked for a second injection, while in the SNM 58% required reprogramming of the device. UTI and CIC were more common in the Onabotulinum toxin A arm [62].
6.6.2 Economic Aspects and Patient Preferences
Economic aspects of onabotulinum toxin A are a concern, due to the price of the drug and the need for repeated cystoscopies. For OAB/idiopathic detrusor overactivity, an assessment of costs from a US payer perspective extending up to 3 years was made for 3 interventions; sacral neuromodulation, onabotulinum toxin A and augmentation cystoplasty in patients refractory to antimuscarinics. The initial treatment cost was $22,226, $1,313, and $10,252 for sacral neuromodulation, onabotulinum toxin A and augmentation cystoplasty, respectively. Three years after initiating treatment, the cumulative cost was $26,269, $7651 and $14,337, respectively. Sensitivity analyses revealed that sacral neuromodulation persisted as the costliest intervention [63].
In a survey of 50 OAB patients, with a mean age of 61 years, 74% preferred onabotulinum toxin A and 26% chose SNM as first treatment option. In those who preferred onabotulinum toxin A, 54% disliked the thought of a foreign body in the back, 46% chose the option due to a shorter waiting list and 43% made the choice based on the quicker onset of benefit. In the SNM group 62% were averse to the potential need for repeated injections and 46% chose SNM to avoid the risk of urinary retention associated with the toxin [64].
6.7 Sacral Nerve Stimulation for OAB

CT scan of a patient with a sacral neuromodulation implant, showing the subcutaneous battery pack (blue arrow) under the skin of the right buttock, and the electrode (orange arrow) next to the right S3 nerve root
Device-related adverse events arise in around 16% of people during test stimulation and 30% of patients after definitive implant placement [68]. The subcutaneous location is essential to minimize risk of infection. Sometimes people are aware of the battery pack, especially if they are slim and the battery is unwisely placed where the patient’s clothing might press on it. Physical activity has to be planned to avoid abrupt movements with a rapid change in direction (e.g. bungee jumping), as this can displace the electrode and cause loss of function. This is less likely with modern electrodes, due to the presence of retaining wings referred to as ‘tines’ [70]. An SNS implant should not be used in patients likely to need an MRI scan for any indication subsequently, as the implant and electrode may have to be removed. The implant is costly, and the expense is ongoing as the battery pack is replaced once it has discharged—though technology advances may soon achieve the ability to recharge percutaneously [71]. The cost in the longer term may be comparable to the expense of other treatments [72].
SNS is an option for patients experiencing insufficient response to botulinum toxin-A treatment, as some of these may benefit sufficiently with SNS [73]. SNS should be chosen in preference to botulinum injections if self catheterisation is not possible for an individual patient.
6.8 Tibial Nerve Stimulation for OAB

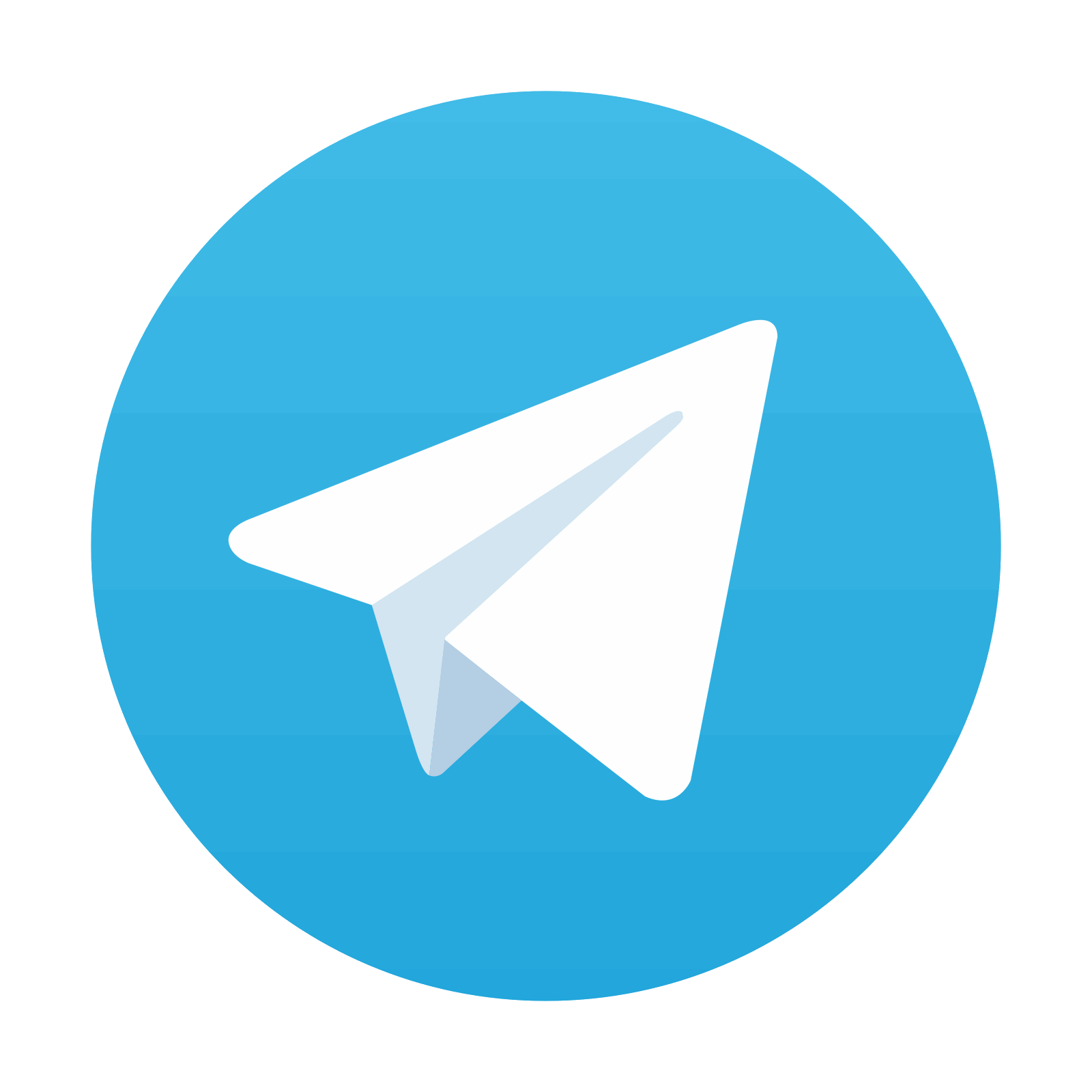
Stay updated, free articles. Join our Telegram channel

Full access? Get Clinical Tree
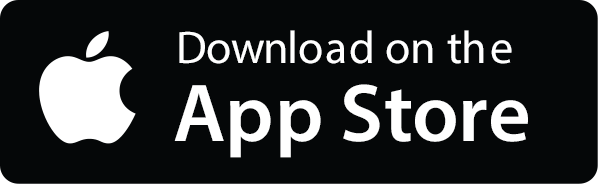
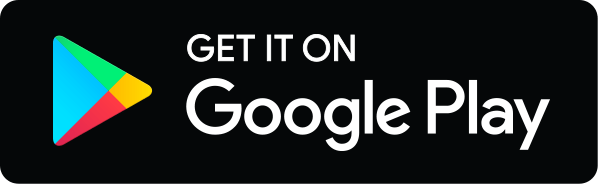