Abstract
Obstruction of the urinary tract is a simple event that initiates a complex series of reactions at the cellular level. A 3-mm ureteral stone has the ability to alter the function of the NA + /K + transport on the basil membrane of a nephron’s tubular cell. Medical treatments continue to focus on reducing the damage prior to the relief of the obstruction. The elevation of hydrostatic pressure in Bowman’s space is followed within minutes by the appearance of PGE 2 and NO to counterbalance the pressure. As these compensatory effects are overwhelmed, GFR and tubular functions deteriorate. Na + reabsorption, acidification, and urinary concentration gradients throughout the nephron are compromised. The fibrosis of prolonged obstruction involves ischemia, apoptosis, and macrophage migration and production. The release of cytokines and growth factors (TGF-β, PAI-1, IL-2, IL-6) is widespread. The classic understanding of fibrosis formation as a loss of the homeostasis of collagen deposition/breakdown within the ECM continues to be true, but the actors have changed with some even reversing their roles. As the older fibrotic pathways are better understood and newer ones discovered, the search for medical treatment to manipulate the pathways continues to be explored. At present, relief of the obstruction remains the only dependable treatment for preventing permanent renal damage. Surgical intervention from PCN to laparoscopic reconstruction of the actual lesion continues to improve with less invasive and more successful techniques. The complexity of obstructive uropathy is clearly more than just a shift in hydrostatic pressure. We must continue to search for a better understanding of the molecular and cellular processes of obstructive uropathy to reduce the lifelong consequences that can occur from a simple 3-mm ureteral stone.
Keywords
obstruction, uropathy, nephron, fibrosis, cytokines, TGF-β, PAI-1 surgery
Obstruction of the urinary tract can occur anywhere between the collecting duct and the urethral meatus. Microcrystals in the collecting duct, urinary calculi, tumors, and luminal strictures may block the normal flow of urine. Regardless of the cause, the ultimate effect is the same: an increase in the hydrostatic pressure of the collecting system, which is transmitted into Bowman space. This reduces the glomerular filtration rate (GFR) and initiates a cascade of events that, if not reversed, will result in kidney scarring and loss of overall kidney function. The extent of kidney injury and the damage to the physical structures of the collecting system vary depending on the duration and completeness of the obstruction. The reduction of kidney function is determined by two components: loss of GFR and the loss of tubular function. Although both are critical, GFR is the dominant contributor. In an unobstructed kidney, tubular function collapses when glomerular filtration is disrupted. The glomerular filtrate is necessary to provide the substrate (i.e., Na + ions) for tubular function. In cases of prolonged obstruction to the kidney, both glomerular and tubular functions are compromised.
Unilateral Ureteral Obstruction
Historically, unilateral and bilateral ureteral obstructions (BUOs) are discussed separately because of distinct changes in kidney physiology. In the first hours after obstruction, differences between the two types of obstructions occur in glomerular blood flow and ureteral pressure profiles. In unilateral ureteral obstruction (UUO), a triphasic event of vascular blood flow and ureteral pressure is seen. Only two phases are seen in BUO. In UUO ( Fig. 45.1 ), there is an initial elevation in the luminal hydrostatic pressure. GFR is maintained by a simultaneous increase in the glomerular capillary pressure induced by afferent arteriolar dilation. Prostaglandin E 2 (PGE 2 ) and nitrous oxide (NO) are considered the initial mediators. Studies using inhibitors of PGE 2 and NO attenuate this increase in renal blood flow and GFR. The exact triggering mechanisms for the production of PGE 2 and NO are less well understood but may be due to the decreased presentation of Na + and Cl − to the macula densa. These increases in GFR and luminal pressure define the first phase.

The second phase of UUO begins with the decrease of glomerular blood flow. Between 12 and 24 hours after the initial obstruction, afferent arteriolar vasodilation transitions to vasoconstriction. Activation of the renin-angiotensin system occurs during the first phase and becomes the dominant process affecting GFR, whereupon efferent and partial afferent arteriole vasoconstriction overwhelms PGE 2 – and NO-mediated vasodilation. Experimental data show that the administration of angiotensin-converting enzyme (ACE) inhibitors blunts the vasoconstriction and GFR reduction. Thromboxane A2 and endothelin reduce glomerular blood flow during UUO. This second phase of UUO is defined by a persistent elevation of hydrostatic pressure from the obstructed lumen, even with the reduction of GFR.
The third and last phase of UUO is marked by decreased luminal hydrostatic pressure and renal blood flow. Glomerular capillary blood flow and luminal pressure remain below baseline until the obstruction is relieved. It is during this last phase that the majority of permanent damage is done to the kidney. The return to baseline function is dependent on the overall duration and severity of the initial obstruction. A partial obstruction may be present for 14 days or more with complete return of function. A total obstruction will leave permanent fibrosis within a week.
Bilateral Ureteral Obstruction
The primary difference between UUO and BUO is persistent vasoconstriction of the efferent artery, which maintains GFR in BUO. Luminal pressure remains elevated for longer than 24 hours in BUO, whereas it begins to decrease by 6 hours with UUO. To account for the persistent afferent arteriolar dilation and efferent arteriolar vasoconstriction, it is likely that additional vasoactive substances accumulate or are produced in BUO but not in UUO. One substance, atrial natriuretic peptide (ANP), is produced in the setting of volume overload and increases diuresis. Vasodilators PGE 2 and NO are likely present because blockade of their production magnifies the already blunted increase in GFR seen in BUO. This suggests that although ANP produces afferent arteriolar dilation, PGE 2 and NO enhance this process.
Tubular Dysfunction
Loss of tubular function during obstruction occurs primarily from a decrease in GFR rather than direct hydrostatic pressure injury to the tubular cells. Sodium and potassium homeostasis, water handling, and acidification are altered. The decline in GFR initiates a series of compensatory yet maladaptive events that are mediated by vasoactive substances, cytokines, and ischemia. These maladaptive events alter the amount of filtrate, the composition of the filtrate, tubular transport proteins, and tubular blood flow ( Table 45.1 ).
|
Sodium Reabsorption
Obstruction of the kidney impairs Na + reabsorption throughout the nephron. The luminal Na + /H + exchanger (NHE3), the luminal Na + /K + /2Cl − cotransporter (NKCC2), and the basolateral Na + /K + -ATPase pump are downregulated in obstructed kidneys. Cell suspension studies of distinct nephron segments support this conclusion. In the proximal tubule, reduction of NHE3 activity is seen. In the loop of Henle, decreased activity of NKCC2 occurs. Diminished activity of the NKCC2 cotransporter is noted in cells studied from the thick ascending limb. Na + reabsorption also requires luminal movement from the lumen into the cell. This process is affected by furosemide, supporting disruption of the NKCC2 transporter. In the medullary portion of the collecting system, NHE3 has reduced energy consumption and decreased expression of NHE3. Many of these transport processes are energy dependent and require ATP. Although a reduction in the amount of available ATP has been hypothesized because of ischemia, it is the downregulation of the receptors and enzymes that appears to be the rate-limiting step in Na + transport.
The actual triggers for the loss of receptor and enzyme activity are still an area of research. Possible signals include decreased filtrate substrates, natriuretic substances, and direct tubular hydrostatic pressure. Decreased GFR reduces filtrate and Na + ion presentation to the cells. Depletion of the Na + ion could downregulate its receptor and transport proteins. Additionally, loss of luminal Na + reduces the electrochemical gradient, whereas blockade of Na + movement into obstructed medullary thick ascending limb cells results in a loss of ouabain-sensitive ATPase. Taken together, decreased Na + presentation to cells could downregulate Na + /K + -ATPase at the translational or posttranslational level.
PGE 2 levels change as a result of obstruction and eventually begin to affect Na + reabsorption. PGE 2 is released during obstruction from increased COX-2 production. COX-2 inhibition reduces the loss of NKCC2 and Na + /K + -ATPase activity, implying an effect of PGE 2 to impair Na + reabsorption.
Na + reabsorption in BUO differs from UUO because of the presence of volume expansion. The addition of ANP and the loss of aldosterone reduce tubular reabsorption of Na + . Much of the impaired Na + handling in UUO is amplified by the effect of ANP, which blocks release of renin and reduces the ultimate creation of angiotensin II. ANP also directly reduces Na + reabsorption in the collecting duct and blocks angiotensin II’s effect on Na + reabsorption, the net effects being diuresis and natriuresis.
Urinary Concentration
Obstruction disrupts normal urinary concentration. With a reduced GFR, less Na + is available to create the osmolar gradient in the medullary interstitium. As with the defects in Na + reabsorption, there is loss of the luminal and basolateral membrane proteins, NKCC2 and Na + /K + -ATPase. This prevents Na + transport from the tubular lumen into the medullary interstitium, which is critical to the countercurrent multiplier that creates the medullary gradient. Without the ability to reabsorb Na + in the ascending limb and dilute the filtrate as it enters the distal convoluted tubule, the solutes required to maintain the gradient are excreted. In the collecting duct of an obstructed kidney, action of antidiuretic hormone (ADH) to increase water permeability is blunted because of decreased AQP-2 in the luminal membrane. Several studies have shown this to be a post-cAMP defect. Reduced transcription of AQP-2 mRNA and a decrease in the phosphorylation necessary to incorporate AQP-2 vesicles into the luminal membrane explain this effect. Finally, a decrease in basolateral membrane AQP-3 and AQP-4 is also noted. After obstruction is relieved, overall urinary concentration returns, paralleling the return of the AQP-2 channel to the luminal membrane.
Urea recycling is another process used by the nephron to increase the gradient for urinary concentration. Urea within the filtrate passively exits the collecting duct at its inner medullary segment and enters the interstitium. The vasa recta and the thin portion of the loop of Henle reabsorb it. A maximum medullary interstitial osmotic gradient is created with the recycling of urea. Urea permeability in the collecting duct tubules is controlled by urea transporters UT-A1 and UT-A3. ADH enhances the permeability of urea, allowing it to flow into the interstitium. Urea reabsorption by the vasa recta is stimulated by UT-B receptors under ADH control. In the obstructed kidney, expression of urea transporters UT-A1, UT-A3, and UT-B is reduced. Urea transporter defects reduce the maximal concentrating effect of the gradient by disrupting urea recycling and allowing urea to be excreted.
Potassium
K + handling in the nephron is not affected directly by obstruction. The initial disturbance in K + homeostasis is explained by defects in Na + handling, H + handling, and reduced GFR. With obstruction, there is a decrease in both Na + reabsorption (reduced Na + channels) and Na + presentation to the distal tubule (reduced GFR). Additionally, decreased K + secretion occurs as a result of increased intraluminal K + . In the low-flow state of obstruction, high urinary potassium concentrations in the collecting duct blunt the gradient between the lumen and the tubular cells, resulting in a reduction in K + movement into the lumen and ultimately hyperkalemia. The effect of obstruction on the renal outer medullary K + channel (ROMK), a K + secretory channel, is presently unknown.
Acidification
Urinary obstruction produces a metabolic acidosis best understood as a form of distal (type 1) renal tubular acidosis (RTA) with hyperkalemia, or “voltage-dependent” RTA. It is characterized by a failure of distal H + and K + secretion. The Na + channel defects play a central role in this acidosis. Loss of Na + reabsorption from the distal tubule results in impaired urinary acidification in the obstructed kidney. Na + /K + -ATPase dysfunction on the basolateral surface of the cell ultimately disrupts Na + removal from the lumen of the collecting duct. This decrease in cation reabsorption reduces the passive H + excretion into the collecting duct lumen down the electrochemical gradient, and a “voltage-dependent acidosis” occurs. Simultaneous hyperkalemia occurs from failure of Na + /K + -ATPase and Na + reabsorption. Decreased expression of the H + -ATPase in the collecting duct adds to the metabolic acidosis. However, a defect of the H + -ATPase transporter cannot account completely for the acidosis seen in hyperkalemic distal RTA due to urinary obstruction. Urinary acidification occurs in the early phases of obstruction, suggesting an intact proton pump. Similarities are noted between voltage-dependent RTA and type 4 RTA. In neither of these two processes is H + secretion felt to be the primary defect.
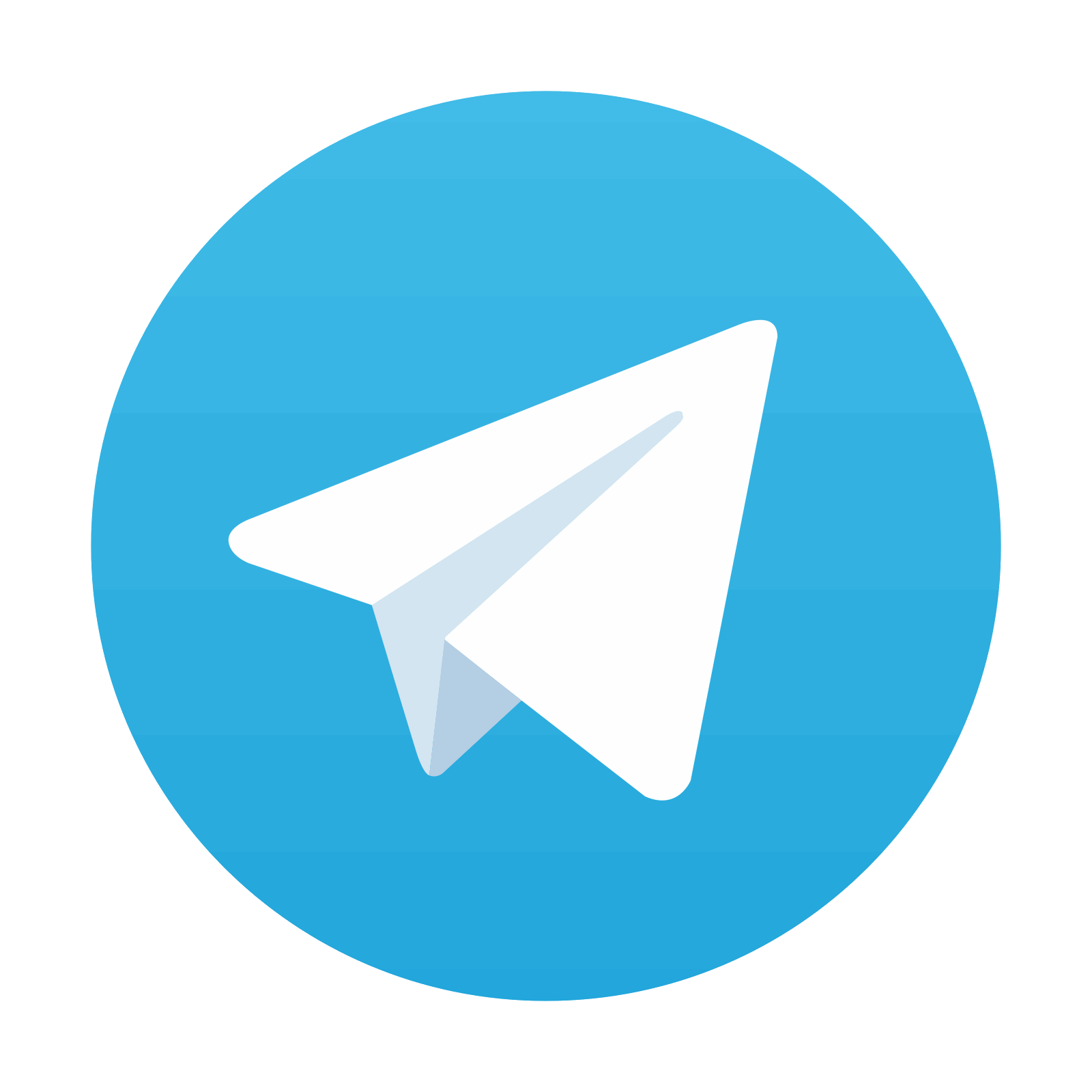
Stay updated, free articles. Join our Telegram channel

Full access? Get Clinical Tree
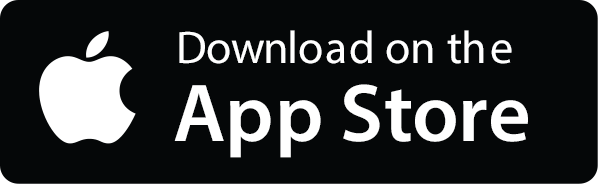
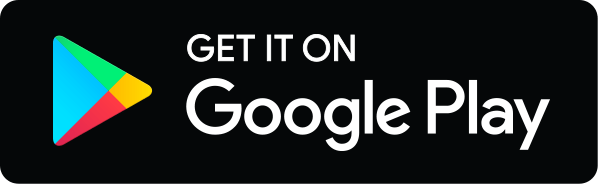