This article describes nucleic acid-based methods to assess the composition and function of the bowel microbiota. The methods range from the relatively simple (polymerase chain reaction) to the technically sophisticated (metatranscriptomics). Not all are accessible to the majority of laboratories, but a core of validated (used in more than 1 study) tools is readily available to most researchers. Reliance on a single methodology per human study is not recommended. Generally, a study could commence with a screening of samples to determine whether it will be worthwhile expending further time and money on an in-depth analysis.
Key Points
- •
Over evolutionary time, people have developed an equilibrium with the microbial world, which consists of cloaking the body inside and out with microorganisms that are likelier to be friends than enemies.
- •
Nucleic acid-based methods of analysis are widely used to determine and monitor the composition of this metaphorical cloak of microbes (microbiota). This was originally because many of the members of microbiota had not yet been cultivated in the laboratory.
- •
Nucleic acid-based methods also facilitate logistical planning and execution of microbiota analysis for probiotic, clinical, and nutritional trials using human subjects.
Is there a need to know about microbiota composition in probiotic studies?
Roy Fuller, a pioneer in the probiotic field, defined a probiotic as a “live microbial feed supplement which beneficially affects the host animal by improving its intestinal microbial balance.” This definition infers that consumption of the probiotic preparation will alter the proportions of the various populations that comprise the microbiota. There is little evidence that this happens, apart from small increases in the abundance of the taxonomic group to which the probiotic belongs. Moreover, the effect is transient, because the probiotic bacteria are only detected in feces as long as the probiotic is consumed. In other words, it is a temporary addition to the microbiota of the large bowel without displacement of resident populations. There is recent evidence that the biochemistry of the microbiota may change during probiotic consumption, but an impact on the immune system by consumption of probiotic bacteria seems to be the main outcome predicted. It can be argued, therefore, that probiotic stimuli are directed at the mucosal immune system of the small bowel as the bolus of bacteria progresses from stomach to colon after consumption.
Until the situation is clarified, and the mechanisms that mediate probiotic activity are discovered, it seems wise to include analysis of the fecal or other microbiota as part of human probiotic trials. The more complete the picture that is generated, the more likely that mechanistic details will be revealed.
While probiotic bacteria are allochthonous to the bowel biome, they should have the capacity to at least survive transit through the human gut after consumption to have some efficacy. Thus detection and quantification of the probiotic strain among the myriad of autochthonous commensals of the large bowel should be accomplished (see section on Why Detect Probiotic Bacteria Using Bulk DNA?).
Human probiotic trials of appropriate statistical power are difficult and expensive to set up and run. The main outcome is usually a clinical read-out such as prevalence of eczema in a test group relative to a placebo control group. Recruits to clinical trials are not usually reluctant to provide fecal samples, and at the least, these can be archived in small aliquots for later microbiota analysis when initial results make this desirable, or additional funding becomes available.
It is recommended that temporal studies of the microbiota be performed, necessitating the collection of specimens for microbiota analysis at several time points during the study. This will provide much needed information about the stability/variability of microbiota compositions over time. A major criticism of gut microbiota research is that human studies, and most experimental animal studies, are one-off; they are never repeated with another group of people or animals. Therefore the consistency of probiotic effects and microbiota composition are not known. This is in contrast to human drug trials, in which repetition of trials in more than one country is required before the drug is approved for general use.
In summary, analysis of the microbiota should be performed because
- •
Probiotics may yet be shown to alter the microbial component of the bowel or other biome in a subtle yet significant way.
- •
Efficacy of probiotic consumption is presumably related to its presence in the bowel or other body site, and this should be demonstrated.
- •
More complete pictures of bowel ecology are required; human trials with probiotics provide possibilities to acquire this knowledge.
Is there a need to know about microbiota composition in probiotic studies?
Roy Fuller, a pioneer in the probiotic field, defined a probiotic as a “live microbial feed supplement which beneficially affects the host animal by improving its intestinal microbial balance.” This definition infers that consumption of the probiotic preparation will alter the proportions of the various populations that comprise the microbiota. There is little evidence that this happens, apart from small increases in the abundance of the taxonomic group to which the probiotic belongs. Moreover, the effect is transient, because the probiotic bacteria are only detected in feces as long as the probiotic is consumed. In other words, it is a temporary addition to the microbiota of the large bowel without displacement of resident populations. There is recent evidence that the biochemistry of the microbiota may change during probiotic consumption, but an impact on the immune system by consumption of probiotic bacteria seems to be the main outcome predicted. It can be argued, therefore, that probiotic stimuli are directed at the mucosal immune system of the small bowel as the bolus of bacteria progresses from stomach to colon after consumption.
Until the situation is clarified, and the mechanisms that mediate probiotic activity are discovered, it seems wise to include analysis of the fecal or other microbiota as part of human probiotic trials. The more complete the picture that is generated, the more likely that mechanistic details will be revealed.
While probiotic bacteria are allochthonous to the bowel biome, they should have the capacity to at least survive transit through the human gut after consumption to have some efficacy. Thus detection and quantification of the probiotic strain among the myriad of autochthonous commensals of the large bowel should be accomplished (see section on Why Detect Probiotic Bacteria Using Bulk DNA?).
Human probiotic trials of appropriate statistical power are difficult and expensive to set up and run. The main outcome is usually a clinical read-out such as prevalence of eczema in a test group relative to a placebo control group. Recruits to clinical trials are not usually reluctant to provide fecal samples, and at the least, these can be archived in small aliquots for later microbiota analysis when initial results make this desirable, or additional funding becomes available.
It is recommended that temporal studies of the microbiota be performed, necessitating the collection of specimens for microbiota analysis at several time points during the study. This will provide much needed information about the stability/variability of microbiota compositions over time. A major criticism of gut microbiota research is that human studies, and most experimental animal studies, are one-off; they are never repeated with another group of people or animals. Therefore the consistency of probiotic effects and microbiota composition are not known. This is in contrast to human drug trials, in which repetition of trials in more than one country is required before the drug is approved for general use.
In summary, analysis of the microbiota should be performed because
- •
Probiotics may yet be shown to alter the microbial component of the bowel or other biome in a subtle yet significant way.
- •
Efficacy of probiotic consumption is presumably related to its presence in the bowel or other body site, and this should be demonstrated.
- •
More complete pictures of bowel ecology are required; human trials with probiotics provide possibilities to acquire this knowledge.
Why use nucleic acid-based tools in microbiota studies?
Much of the bacteriologic information about the bowel community has been generated by the use of nucleic acid-based methodologies. The phylogenetic analysis (determining the phyla, families, genera, and species through molecular sequencing data and construction of data matrices) has mostly relied on comparisons of sequences of the small ribosomal subunit RNA genes (16S rRNA gene in the case of bacteria) present in DNA extracted from feces or other samples. A large database of about 2 million 16S rRNA gene sequences is currently available and provides a cornerstone of bacterial detection and identification by molecular methods.
Nucleic acid-based methods of detection have indicated that most (about 90%) bacterial cells seen microscopically in terrestrial and aquatic samples, even accounting for the possibility that some are dead, have not yet been cultured in the laboratory. This observation was totally unexpected in relation to traditional experiences in medical bacteriology and has been called the great plate count anomaly. Operational taxonomic units (OTU, molecular species) never encountered in culture-based bacteriology but detectable by nucleic acid-based methods have revealed a vast, new bacterial world for investigation.
The situation with human feces is not dire, since at least 50% of the cells seen in samples can be cultivated. Additionally, representatives of most of the metabolic types of bacteria in the human colon have been cultured. It is still convenient, however, to use nucleic acid-based methods to determine the composition and potential or real-time functioning of bowel communities. This is because of the diversity of bacterial types present in the bowel of people and the somewhat idiosyncratic nature of individual microbiota. Extraction of nucleic acids from feces, bowel digesta, or mucosal biopsies is now a standard procedure and is logistically much simpler than the preparation of a spectrum of selective media and anaerobic protocols that are required for culture-based investigations. Moreover, culture-based analysis requires the processing of the samples soon after collection, whereas materials for nucleic acid-based analysis can be frozen after collection and stored at low temperature (dry ice) during transport to the laboratory and while awaiting analysis. DNA, when stored at very low temperature (-80°C), provides a useful archive of microbial genomes that might be used in the future when advanced methodologies may increase the amount of information that can be gleaned from bulk DNA.
The starting point for nucleic acid-based analysis is the extraction of DNA or RNA directly from the fecal or other sample of interest, avoiding the need to cultivate any members of the community. A critical step in the procedure is the lysis of microbial cells to release the nucleic acids, because accurate representation of all of the microbial types in the sample is required. Although chemical methods have been used, mechanical disruption of the cells (bead-beating) is considered preferential, because even gram-positive bacterial cells, often resilient to other methods, will be lysed.
The 16S rRNA gene sequence of bacteria has become the basis of bacterial phylogeny, because it contains regions of nucleotide base sequence that are highly conserved across the bacterial world. These conserved regions are interspersed with variable regions (V regions) that contain the signatures of phylogenetic groups even to species level. Therefore sequences of V regions of 16S rRNA genes are the basis of phylogenetic methods, as will be reinforced in subsequent sections of this article.
It is important to remember, however, that bulk DNA has come from any cells that were present in the sample at the time of collection. The nucleic acids may have come from resident, living, metabolizing cells, or resident, living but relatively quiescent cells or spores, from dead bacteria, or living bacteria adventitiously present at the time of collection. Detection of a particular DNA sequence in a single sample does not equate to evidence of residence of the organism in the ecosystem. Collection and analysis of samples over time are necessary to provide this evidence.
The capacity to sequence and assemble genomic information from fragments of DNA (metagenomics) or mRNA (transcriptomics) has extended the use of nucleic acids in researching the potential and actual metabolic capacity of the microbiota. These exciting approaches will be outlined in other sections of this article.
In summary, nucleic acid-based analysis of fecal or other samples from people is useful because
- •
It enables descriptions to be made of the phylogenetic composition of the microbiota including its yet to be cultivated members.
- •
It has logistical advantages with respect to transport and storage of samples.
- •
It enables the genetic potential of the microbiota to be determined (metagenomics).
- •
It enables the expression of genes at a point in time to be revealed (transcriptomics).
Why detect probiotic bacteria using bulk DNA?
By current definition, probiotics are live microorganisms, which when administered in adequate amounts confer a health benefit on the host. Most probiotics are administered as dietary supplements either in milk-based foods or as tablets or capsules. The detection of the probiotic bacteria in fecal or other samples is not routine in efficacy trials with people. However, inclusion of an assay to detect the presence or absence of the probiotic during the progress of the trial seems a wise step, since any absence of efficacy could be due to the inability of the probiotic to survive transit through the gastrointestinal tract. Even when probiotic efficacy has been established, a mechanistic explanation is often lacking. Hence the more detailed microbiological data available that can be interrogated statistically, the better.
A study demonstrating the differential effect of 2 probiotics in the prevention of eczema and atopy provides a case in point. The aim of the study was to determine whether probiotic administration in early life could prevent the development of eczema and atopy at 2 years of age. A double-blind, randomized placebo-controlled trial of infants at risk of allergic disease was performed. Pregnant women were randomized to take Lactobacillus rhamnosus HN001, Bifidobacterium animalis subspecies lactis HN019, or placebo, daily from 35 weeks gestation until 6 months if breast-feeding, and their infants were randomized to receive the same treatment from birth to 2 years. Four hundred and seventy-four infants were included in the study. The infants’ cumulative prevalence of eczema and point prevalence of atopy (skin prick tests to common allergens) were assessed at 2 years. Infants receiving L. rhamnosus probiotic had a significantly reduced risk of eczema (hazard ratio [HR] 0.51, 95% confidence interval [CI] 0.30–0.85, P = .01) compared with placebo, but this was not the case for the B. animalis subspecies lactis group (HR 0.90, CI 0.58–1.41).
Why was one probiotic effective but the other not? One possible explanation concerned the markedly different rates of detection of the probiotics in the stools of the infants during the trial. A DNA-based technique (polymerase chain reaction [PCR] coupled with an electrophoretic detection method; see section on Electrophoretic Methods to Screen Microbiota Compositions) showed that B. animalis subspecies lactis prevalence in fecal samples increased progressively over the course of the study, from 22.6% of infants at 3 months to 53.1% of infants at 24 months. In contrast, detection of L. rhamnosus was greatest at 3 months, at 71.5% of babies, and slightly lower at 24 months, at 62.3% among infants administered this probiotic. Therefore, the efficacious probiotic ( L. rhamnosus ) was commonly present in the bowel of the infants from an early age, whereas the ineffective probiotic did not seem to transit the gut in about half of the infants.
An indication of the numbers of probiotic bacteria in fecal samples could give additional interpretative data in trials with people. However, it must be kept in mind that absolute guarantees of specificity (quantifying only a specific strain—the probiotic—in samples collected from nature) require a cautionary interpretation. Limited numbers of strains of the probiotic species are usually available, either in laboratory collections or in the form of genome sequences. Therefore, validation of specificity of the nucleic acid-based method is subject to relatively limited knowledge when the study was designed and performed.
In summary, detection of probiotic strains in feces by DNA-based assays
- •
Demonstrates that the probiotic bacteria have at least survived transit through the gut (although viability cannot be demonstrated)
- •
May aid in the interpretation of efficacy outcomes in trials of probiotics with people
Electrophoretic methods to screen microbiota compositions
A relatively simple, semiquantitative screening method to compare the bacterial compositions of multiple samples is provided by PCR coupled with gradient gel electrophoresis. Either a chemical gradient (denaturing gradient gel electrophoresis, DGGE) or temperature gradient (temporal temperature gel electrophoresis, TTGE) is used. A V region of bacterial 16S rRNA genes is amplified from bulk DNA from samples using PCR. The PCR primers anneal with conserved sequences that span the selected V region. One of the primers has a GC-rich 5′ end (GC clamp) to prevent complete denaturation of the amplified DNA fragments during gel electrophoresis. The amplified DNA theoretically contains 16S rRNA gene sequences from all of the members of the microbiota in the sample. All of the amplified fragments are of the same size. A polyacrylamide gel is used to separate the fragments of 16S rRNA gene sequences that originated in different kinds of bacteria. The double-stranded fragments of DNA migrate in an electrical current through the polyacrylamide gel until they reach chemical or thermal conditions that represent their melting point (separation of polynucleotide strands). The migration rate of the partially denatured fragments (remember the GC clamp) is slowed. Because of the variation in the 16S rRNA gene sequences between phylogenetic groups, the DNA fragments from different kinds of bacteria have different melting points and hence show different migrations in the gel. Profiles of microbiota composition can be generated once the electrophoretic gel has been stained to reveal bands of DNA. Images of these profiles can be compared using computer software.
For example, differential clustering of bowel biopsy-associated bacteria of specimens collected in Mexico and Canada was demonstrated using PCR-TTGE. Bacterial collections associated with bowel biopsies, aspirates of residual fluid after pre-endoscopy cleansing, and feces from inflammatory bowel diseases (IBD) patients and healthy subjects in Edmonton, Canada, and Mexico City, Mexico, were investigated. PCR-TTGE produced profiles of the bacterial collections whose similarities were compared. Similarity analysis showed that the profiles did not cluster according to disease status, but the Canadian and Mexican profiles could be differentiated by this method. Comparison of biopsy, aspirate, and fecal samples obtained from the same subject showed that, on average, the profiles were highly similar. Therefore, biopsy-associated bacteria are likely to represent, at least in part, contaminants from the fluid, which resembles a fecal solution, that pools in the bowel after cleansing before endoscopy.
Individual fragments of DNA can be cut from electrophoretic gels, further amplified and cloned in a surrogate host ( Escherichia coli ), then sequenced. The sequence can be compared with those in 16S rRNA gene databanks to obtain identification of the bacterium from which the sequence originated. PCR primers specific for particular groups of bacteria (for example lactic acid bacteria), rather than universal primers used to detect all bacteria, can be designed. These primers produce simpler profiles that can be useful in the detection of bacterial species commonly used as probiotics.
In summary, electrophoretic methods such as DGGE and TTGE
- •
Provide comparative snapshots of microbiota
- •
Can detect, using appropriate PCR primer design, specific bacterial groups within the microbiota
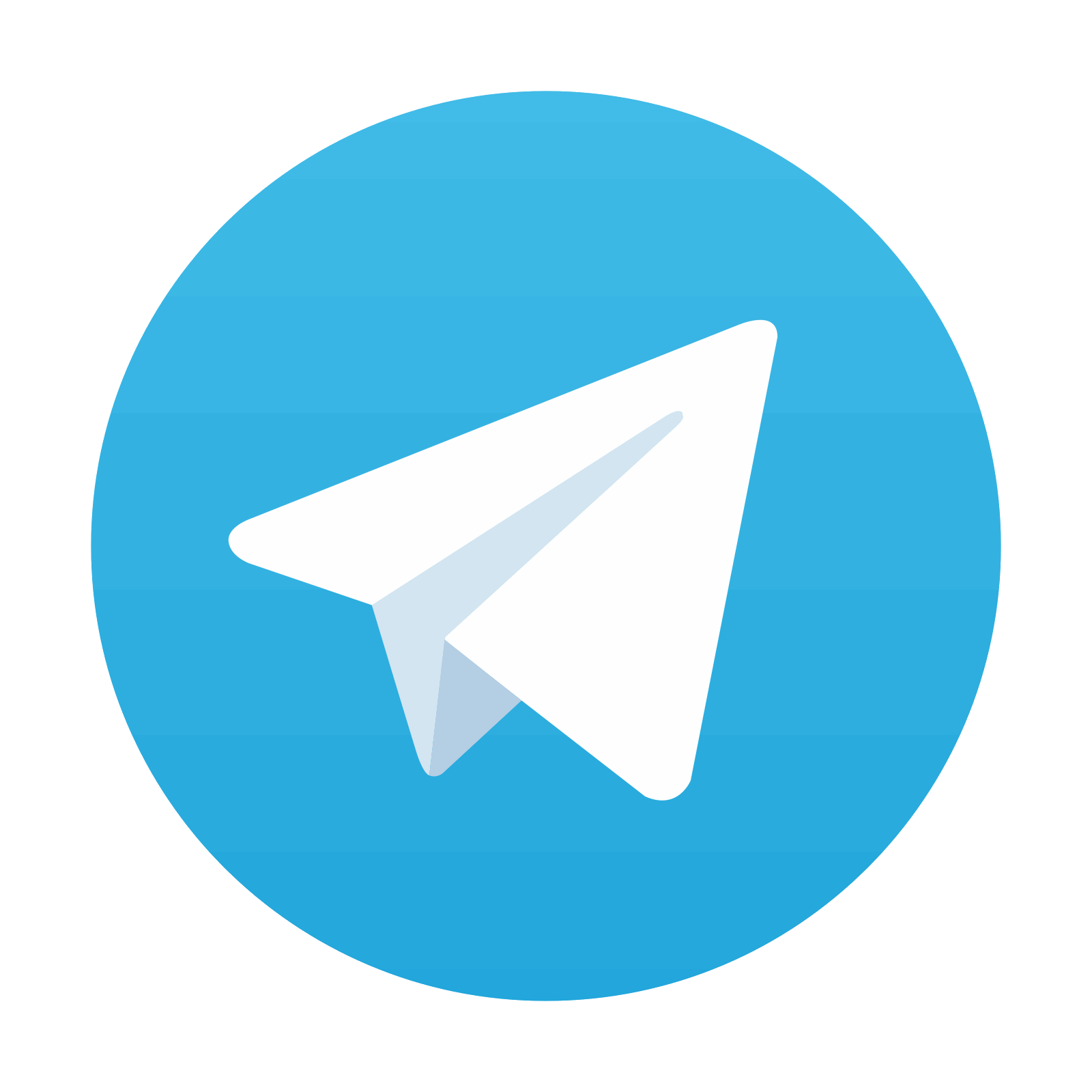
Stay updated, free articles. Join our Telegram channel

Full access? Get Clinical Tree
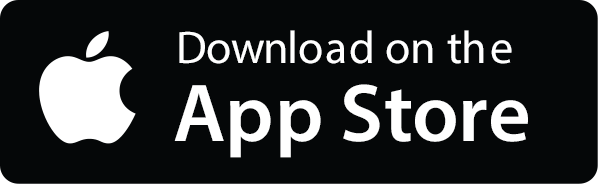
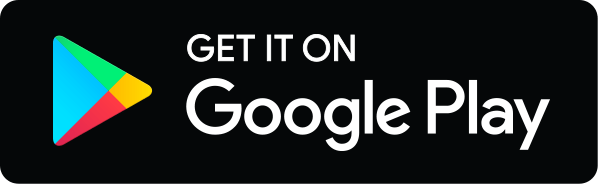