Currently, the only available therapy for celiac disease is strict lifelong adherence to a gluten-free diet (GFD). Although safe and effective, the GFD is not ideal. It is frequently expensive, of limited nutritional value, and not readily available in many countries. Consequently, a need exists for novel, nondietary therapies for celiac disease. Based on the current understanding of celiac disease pathogenesis, several potential targets of therapeutic intervention exist. These novel strategies provide promise of alternative, adjunctive treatment options but also raise important questions regarding safety, efficacy, and monitoring of long-term treatment effect.
Key Points
- •
Currently, the only available therapy for celiac disease is strict lifelong adherence to a gluten-free diet.
- •
Based on the current understanding of celiac disease pathogenesis, several potential targets of therapeutic intervention exist.
- •
These novel strategies provide promise of alternative, adjunctive treatment options but also raise important questions regarding safety, efficacy, and monitoring of long-term treatment effect.
- •
The development of surrogate markers for celiac disease activity should be a concomitant priority along with ongoing drug development.
Introduction
Celiac disease is a common cause of chronic small intestinal inflammation and is one of the most common autoimmune disorders in the developed world. The prevalence of this disease ranges from 1:70 to 1:200 based on antibody screening and confirmatory intestinal biopsy data from North and South America and Western European and Middle Eastern countries. Factors critical in precipitating celiac disease have been well established, including a defined trigger, specifically gluten proteins from wheat and related cereal proteins; the presence of HLA class II genes DQ2 or DQ8; and the generation of autoantibodies to tissue transglutaminase 2 (TG2). TG2 plays a pivotal role in immune activation by deamidating specific gliadin peptides, which increases their affinity for HLA-DQ2 or HLA-DQ8. The deamidated gliadin peptide–TG2 complexes presented by antigen-presenting cells can elicit a vigorous adaptive immune response characterized by activation of CD4+ T-helper 1 (Th1) cells. Apart from this adaptive immune response, wheat protein also triggers innate immunity, which is not restricted to patients with celiac disease. The dual activation of both the adaptive and innate immune responses enhances intestinal mucosal inflammation, which is manifested clinically with a variable spectrum of disease presentations, ranging from asymptomatic disease to severe malabsorption.
Introduction
Celiac disease is a common cause of chronic small intestinal inflammation and is one of the most common autoimmune disorders in the developed world. The prevalence of this disease ranges from 1:70 to 1:200 based on antibody screening and confirmatory intestinal biopsy data from North and South America and Western European and Middle Eastern countries. Factors critical in precipitating celiac disease have been well established, including a defined trigger, specifically gluten proteins from wheat and related cereal proteins; the presence of HLA class II genes DQ2 or DQ8; and the generation of autoantibodies to tissue transglutaminase 2 (TG2). TG2 plays a pivotal role in immune activation by deamidating specific gliadin peptides, which increases their affinity for HLA-DQ2 or HLA-DQ8. The deamidated gliadin peptide–TG2 complexes presented by antigen-presenting cells can elicit a vigorous adaptive immune response characterized by activation of CD4+ T-helper 1 (Th1) cells. Apart from this adaptive immune response, wheat protein also triggers innate immunity, which is not restricted to patients with celiac disease. The dual activation of both the adaptive and innate immune responses enhances intestinal mucosal inflammation, which is manifested clinically with a variable spectrum of disease presentations, ranging from asymptomatic disease to severe malabsorption.
Pathogenesis of celiac disease
Genetic and Environmental Factors
The interplay of genetic and environmental factors contributes to the enteropathy of celiac disease. The central role of HLA class II genes and to a lesser extent non-HLA genes as predisposing hereditary factors has been validated. Most patients with celiac disease carry a variant of HLA-DQ2 (DQ2.5; DQA1*05/DQB1*02), whereas the remaining patients carry HLA-DQ8 (DQA1*03/DQB1*0302). These class II molecules are expressed on antigen-presenting cells, predominantly macrophages, dendritic cells, and B cells. The HLA genes play a critical role in disease pathogenesis by preferentially presenting gluten peptides to CD4+ Th1 cells in the lamina propria. The T cells become activated on recognition of the gluten peptides, and subsequently function as central effector cells of inflammation by releasing different cytokines, notably, interferon (IFN)-γ, a key marker of the inflammatory Th1 response. The release of these cytokines precipitates a cascade of immune, mesenchymal, and epithelial cell activation in the small intestine, resulting in the hallmark lesions of crypt hyperplasia, villous atrophy, and increased intraepithelial lymphocytes. Recent genome-wide association studies and linkage analyses have identified several non-HLA loci implicated in celiac disease susceptibility, putatively through their effects on T-cell regulation and inflammation. However, the overall increased genetic risk conveyed by these polymorphisms is likely modest, estimated at 3% to 4% compared with a 30% to 35% contribution by HLA-DQ2 and/or HLA-DQ8.
In addition to genetics, environmental factors play an important role in celiac disease pathogenesis. Gluten is the most significant identified factor. Dietary gluten contains several distinct T-cell epitopes rich in proline and glutamine residues. The high proline content of dietary gluten leads to peptides that are not easily degraded by gastrointestinal proteases, leading to an elevated concentration of potentially immunogenic epitopes in the small intestine. IgA-TG2, a ubiquitous intracellular and facultative extracellular enzyme that can associate with the extracellular matrix, plays a central role in celiac disease pathogeneis. TG2 targets certain glutamine residues found in dietary gluten and deamidates them to negatively charged glutamic acid residues. The negatively charged gluten peptides are able to bind with greater affinity to HLA-DQ2 or HLA-DQ8, leading to enhanced gluten-specific CD4+ Th1 cell activation. TG2 also deamidates a 33-amino-acid (33-mer) peptide from α2-gliadin, considered to be a prominent celiac disease antigen. The exact mechanism through which the immunogenic gluten peptides reach the lamina propria from the intestinal lumen is debatable, and several theories have been proposed. The release of zonulin, a gluten-induced mediator of tight junctional disassembly and leakage by intestinal epithelial cells, was suggested as a major mechanism, whereas others showed that enhanced transport of gluten into the lamina propria may occur via epithelial transcytosis. A third possibility, shown in a murine model of celiac disease, is that dendritic cells residing in the lamina propria preferentially bind gluten peptides through projecting protrusions between intestinal epithelial cells, reaching and thus sampling the intestinal lumen. Another hypothesis is that specialized intestinal epithelioid cells (M cells, an integral part of the follicle-associated mucosal lymphoid tissue) may preferentially process gluten peptides ( Fig. 1 ). A more detailed description of the pathophysiology of celiac disease is discussed elsewhere in this issue by Kupfer and Jabri.
A need for nondietary therapies
Currently, the only available therapy for celiac disease is strict lifelong adherence to a gluten-free diet (GFD). Although the GFD is proven to be a safe and effective therapy, it is not ideal. One problem is that gluten-free labeling laws in the United States and some European countries only include food products that contain less than 20 parts per million of gluten, whereas the threshold for what is considered safe or clinically acceptable exposure to gluten can vary widely for patients. Moreover, the GFD is expensive, not readily available in many countries, and may be lower in its nutritional value, which can significantly impact patient adherence and quality of life. Even with strict adherence to the diet, some patients can have progressive or unresponsive disease that requires combination therapy for management. Studies have also shown that histologic improvement does not necessarily correlate with improved clinical response or strict adherence to the GFD. In a recent survey study from the United Kingdom, 40% of patients with celiac disease expressed dissatisfaction with the GFD. Important reasons for this included dietary and social restrictions imposed by having to avoid all possible hidden sources of gluten, but also the higher costs and often lower palatability of gluten-free foods. All 300 patients expressed interest in novel therapies, with a vaccine being the preferred choice, followed by an anti-zonulin agent or peptidase therapy. The survey found that nearly 20% of patients use complementary or alternative medicine in their management of celiac disease. Generally, the initial goal for any nondietary therapy for celiac disease is to neutralize low doses of gluten ingested through unintentional or unavoidable gluten exposure.
It is anticipated that nondietary therapy for celiac disease will have to meet stringent criteria regarding safety and toxicity given that the current modality, the GFD, can be considered mostly safe. The expectation is that topical or luminal agents should have very low potential for toxicity. Consequently, approaches that alter the immune system may be considered only for severe cases given the potential for greater toxicity secondary to immunosuppression. To determine efficacy, subjective and objective outcome measures would be required. The U.S. Food and Drug Administration has produced guidelines on the use of patient-reported outcomes, and these are likely to be valuable in celiac disease. A need also exists for surrogate markers of disease activity as an objective measure of efficacy. Based on the current understanding of celiac disease pathogenesis, several potential targets of therapeutic intervention exist. This article discusses the therapeutic strategies that have been studied in preclinical models of celiac disease and/or show promise in phase I and II clinical trials ( Fig. 2 and Table 1 ).
Target | Compound | State of Development | References |
---|---|---|---|
Gluten modification | |||
Engineered grains | Genetically modified wheat variants with lower immunogenicity | Preclinical | |
Pretreated flour | Pretreatment with lactobacilli | Preclinical | |
Pretreatment with microbial TG and N -methyl-lysine | Preclinical | ||
Oral proteases | Prolyl endopeptidases, AN-PEP, STAN-1 | Phase I and II clinical trial | |
ALV003 | Phase II clinical trial | ||
Intraluminal therapies | |||
Gluten-sequestering polymers | Polymer resin, P(HEMA-co-ss) | Preclinical | |
Neutralizing gluten antibodies | Cow’s milk antigluten antibodies | Preclinical | |
Gluten tolerization and immune modulation | |||
Hookworm infection | Necator americanus | Phase II clinical trial | |
Mucosal tolerance | Genetically modified Lactococcus lactis | Preclinical | |
Gluten vaccination | Nexvax2 | Phase II clinical trial | |
Modulation of intestinal permeability | |||
Zonulin receptor antagonists | AT-1001 | Phase IIb clinical trial | |
Rho kinase inhibition | Fasudil | Approved drug | |
RhoA inhibition | BA-210 | Phase II clinical trial | |
Mitogen for intestinal epithelium | R-spondin 1 | Phase III clinical trial for Crohn disease | |
Downregulation of the adaptive immune response | |||
Tissue transglutaminase 2 inhibitors | ZED-101 | Preclinical | |
Inhibitory gluten peptides | Innate immunity inhibitory decapeptide, QQPQDAVQPF | Preclinical | |
HLA-DQ2 inhibitors | DQ2-blockers | Preclinical | |
Immune cell–targeted therapies | |||
CCR9 antagonists | Ccx282-B, CCX025 | Phase II clinical trials planned | |
Anti–integrin α4β7 | Vedolizumab (also known as LDP-02, MLN02, MLN0002) | Phase II clinical trial for Crohn disease | |
Anti–α4 integrin | Natalizumab | Phase II trial in Crohn disease | |
Inhibition of CD40-CD40L | Anti–CD40-CD40L | Preclinical | |
Anti–IFN-γ | Fontolizumab | Phase II clinical trial for Crohn disease, discontinued | |
Anti–TNF-α | Infliximab, adalimumab | Infliximab for RCD | |
IL-15 antagonists | AMG714 | Phase II clinical trial in rheumatoid arthritis and psoriasis | |
IL-10 agonist | Recombinant human IL-10 | Phase I clinical trial | |
Anti-CD3 | Visilizumab, teplizumab, otelixizumab | Visilizumab: phase II in UC, GvHD Teplizumab: phase II in T1D Otelixizumab: phase III in T1D | |
Anti-CD20 | Rituximab, tositumomab, ibritumomab | Approved drug | |
Bone marrow transplantation | Clinical trial in patients with EATL | ||
Mesenchymal stem cell therapy | Phase II clinical trial for Crohn disease |
Intraluminal therapies
Food Modification
Wheat strains can be selected for lower immunogenicity or engineered to decrease or eliminate immunogenic T-cell epitopes. Strategies have used RNA interference to silence the gluten genes that contain celiac disease immunogenic epitopes or to engineer wheat strains with reduced immunogenic epitope content. Hexaploid wheat strains have been bred from ancient diploid and tetraploid wheat species over thousands of years. These ancient wheat strains have been shown to confer significantly less immunogenicity, a property that goes hand in hand with reduced α, β, γ, and ω ´ gliadins. Psyllium has recently been studied as a replacement for gluten because it has minimal effects on food odor or texture while still retaining desired baking properties. Bread prepared from psyllium was rated highly among patients with celiac disease and nonceliac controls for its texture and taste. Studies have also evaluated the genetic modification of wheat through the deletion of key gliadin genes. Specifically, the deletion of the α-gliadin locus on chromosome 6 in the hexaploid modern wheat strain Triticum aestivum led to a decrease in T-cell stimulatory epitopes without significant alterations in baking properties. The deleted gliadin genes will likely need to be replaced by nonimmunogenic gliadin variants or avenins to approximate normal dough elasticity. This approach remains in preclinical investigation.
Lactic acid bacteria, such as the lactobacilli of sourdough, possess several peptidases. When added to sourdough for fermentation, lactobacilli are able to proteolyze the proline/glutamine-rich gluten peptides, including the highly immunogenic 33-mer peptide from α2-gliadin. A pilot double-blind study of 17 patients with celiac disease investigated symptomatic response to 2 types of bread containing 2 g of gluten: bread produced with baker’s yeast and bread produced with lactobacilli. Most patients who ingested the bread made from baker’s yeast developed enhanced intestinal permeability, as measured by the excretion of carbohydrates lactulose and L-rhamnose, whereas the patients who ingested the bread pretreated with lactobacilli did not show any intestinal permeability changes. However, patients were challenged for only 2 days in this study, and therefore firm conclusions cannot be drawn and doubt remains if immunogenic gluten peptidase will be completely inactivated. Another interesting finding is the presence of gluten-degrading proteases produced during germination of wheat. Unfortunately, bread made from flour of germinating wheat, rye, or barley leads to elimination of the major gliadin and glutenin proteins, resulting in unpalatable and crumbly bread. Another therapeutic approach in preclinical testing is to incubate gliadin with TG2 and lysine methyl ester. Lysine-modified gliadins lose their affinity for HLA-DQ2, leading to diminished activation of intestinal T cells. Pretreatment of whole wheat flour with lysine methyl ester and microbial transglutaminase (TG) derived from Streptomyces mobaraensis greatly diminished the stimulatory effect of the flour on the T-cell response. Flour pretreated with microbial TG also improves the texture and volume of bread. However, the use of microbial TG in food preparation remains a matter of debate. Further studies are required to develop and validate nonimmunogenic flours and to produce bread and other baked products with good consistency and adequate nutritional value.
Oral Proteases for Gluten Detoxification
Gluten proteins have a high proline and glutamine content, making them partly resistant to proteolysis by gastric, pancreatic, and intestinal brush border endopeptidases and exopeptidases, which have poor affinity for peptide bonds adjacent to proline and glutamine residues. This resistance results in incomplete proteolysis of gluten with the accumulation of long oligopeptides, such as the gliadin 33-mer and 26-mer peptide fragments, which can potently elicit an HLA-DQ2– or HLA-DQ8–restricted T-cell inflammatory response in patients with celiac disease. One strategy to prevent these peptides from reaching the lamina propria is to use prolyl endopeptidases (PEPs) expressed in various microorganisms, such as Flavobacterium meningosepticum or Aspergillus niger , that can cleave and thus inactivate these immunodominant, proline-rich peptides. In a randomized, double-blind, cross-over study involving 20 asymptomatic patients with biopsy-proven celiac disease in remission, patients consumed 5 g of gluten for 14 days and were subsequently crossed-over to consume gluten pretreated with PEP derived from F meningosepticum for an additional 14 days. The study found that pretreatment of gluten with PEP mitigated the development of fat or carbohydrate malabsorption in most patients who developed these abnormalities after the 2-week gluten challenge.
However, the use of PEP therapy can be limited by the length of the substrate and, except for the A niger enzyme, usually requires near-neutral pH for maximum activity and a long period (>1 h) to completely digest the immunogenic gliadin peptides. The ability of A niger PEP (AN-PEP) to degrade gluten was studied in a dynamic system closely mimicking the gastrointestinal tract, where it accelerated the degradation of gluten in the stomach compartment to such an extent that hardly any immunogenic gluten reached the duodenal compartment. In addition, the proenzyme of barley endoprotease B (EP-B2) is effective at digesting gluten in rat stomach in a dose- and time-dependent manner. Consequently, combination therapy with EP-B2 and PEP from Sphingomonas capsulata has been shown to effectively break down whole wheat gluten in vitro and in a rat model. PEPs and EP-B2 can be administered as lyophilized powders in capsules or tablets, and these formulations are currently in phase I/II clinical trials. Another enzyme cocktail, called STAN1, has been developed with modest capacity to detoxify gluten. This cocktail is undergoing phase II evaluation.
An additional drug candidate, ALV003, is being developed as an orally administered, fixed-dose (1:1 ratio by weight) mixture of 2 glutenases (ALV001 and ALV002). ALV001 is a modified recombinant version of the proenzyme form of cysteine endoprotease, EP-B2, from barley. ALV002 is a modified recombinant version of a PEP from the bacterium S capsulata (SC-PEP). To date, ALV003 has been evaluated in 3 phase I and IIa clinical studies. In general, ALV003 was found to be safe and well tolerated, with no dose-limiting toxicities. Moreover, it was found to be highly effective in degrading gluten primarily in the stomach before it reached the duodenal compartment. In a study of 20 patients with biopsy-proven celiac disease who were randomly assigned to gluten (16 g daily for 3 days) pretreated with ALV003 or placebo, no significant improvements were seen in clinical response with ALV003, although predigestion of gluten with ALV003 did improve immune responses. In other words, biopsy data showed less small intestinal mucosal injury in patients treated with ALV003 compared with those treated with placebo. The percentage of intraepithelial lymphocytes was essentially unchanged in the ALV003-treated patients but significantly increased in the placebo-treated patients. ALV003 is currently being investigated in further phase II studies with the goal of establishing its efficacy and safety in preventing gluten-related symptoms and immune responses in patients with celiac disease.
The major foreseeable problem with oral enzyme therapy is that whether the quantity of enzyme and the time available for it to act will be sufficient to degrade the immunotoxic gluten peptides in a daily gluten load (10–20 g) is unclear. However, oral enzyme therapy may eliminate the detrimental side effects from smaller gluten exposures and therefore may benefit patients’ sensitivity to low-level gluten exposure, and reduce the incidence of refractory celiac disease type I (RCD I) from inadvertent low-level gluten ingestion.
Gluten-Sequestering Polymers
Sequestration of gliadin intraluminally using an oral polymeric resin, poly(hydroxyethyl methacrylate-co-styrene sulfonate) [P(HEMA-co-ss)], was suggested as a strategy to block access of the immunotoxic gluten peptides to the mucosal immune cell compartment. In vitro studies showed that this polymer bound gliadin in a specific manner and prevented its digestion to immunogenic peptides. In vivo studies in HLA-DQ8+ transgenic mice sensitized with gliadin showed that the polymer reduced mucosal injury. Potential problems with this strategy include lack of specificity for gliadin and potential binding of other nutrient proteins. Moreover, which dose of gluten can be effectively bound in vivo by a given dose of polymer is unclear.
Neutralizing Gluten Antibodies
Orally acting IgG antibodies can be used to specifically bind and inactivate luminal antigens. Cows are a readily available source of large quantities of milk (colostrum)-derived antibodies. The large-scale production of cow’s milk gluten-neutralizing antibodies for oral use has been proposed as a safe therapeutic option. These antibodies could be labeled as food additive and used as adjunctive therapy to the GFD when trace ingestion of gluten is unavoidable, such as during travel, social, and business events. National Institutes of Health–sponsored preclinical testing is underway, with plans for a phase I trial in the United States in the near future.
Gluten tolerization and immunomodulation
Hookworm Infection
It has been proposed that chronic helminthiasis, such as hookworm infection, may alter pathogenic Th1-weighted immune responses in diseases such as inflammatory bowel disease and possibly celiac disease. A pilot, randomized, double-blinded, placebo-controlled study was performed over 21 weeks in 20 healthy helminth-naïve adults with celiac disease on a strict GFD who were infected with the hookworm Necator americanus. In general, hookworm administration in patients with celiac disease did not result in a clinically significant suppression of intestinal pathology although there was a trend toward reduced mucosal inflammation after wheat challenge. This study confirmed the safety and feasibility of using hookworm infection as a model to study immune responses in celiac disease and other intestinal inflammatory disorders.
Mucosal Tolerance Induction
Mucosal tolerance to gluten has been demonstrated in murine models of celiac disease. In both wild-type and HLA-DQ8 transgenic mice, intranasal administration of α-gliadin diminished T-cell proliferation and IFN-γ secretion after peripheral immunization. Based on these results, a strategy to induce gluten-specific tolerance was devised through the active delivery of immunogenic gliadin peptides to the intestinal mucosa by genetically modified Lactococcus lactis (LL). LL expresses a deamidated, HLA-DQ8-restricted immunodominant gliadin epitope (LL-eDQ8d). In a DQ8 transgenic mouse model, oral administration of LL-eDQ8d induced suppression of local and system T-cell responses to gliadin peptide and induced production of interleukin (IL)-10, transforming growth factor β, and Foxp3+ regulatory T cells. It will be important to know if T-cell responses to other gluten epitopes can be suppressed via this approach. Moreover, this approach has not been validated in any human study.
Gluten Vaccination
Protein-based desensitization is the cornerstone of therapy for various allergic diseases. The most likely mode of action is the induction of CD4+ regulatory T cells to suppress T-cell–mediated inflammation. In celiac disease, the aim of this therapy is to shift the T-cell response from proinflammatory to regulatory; in other words, to tolerance to gluten. Three select immunogenic 16-mer peptides derived from α-gliadin, ω ´ -gliadin, and hordein that account for 60% of the overall gluten T-cell response have been identified in a remarkable effort and studied in a TCR/DQ2 transgenic murine model. Gluten vaccination using these 3 peptides via subcutaneous injection induced enhanced tolerance in this celiac murine model through the suppression of CD4+ T-cell proliferation and IL-2 and IFN-γ production, and through increased expression of regulatory T cells. This gluten vaccine (ImmusanT, Nexvax2 [Cambridge, MA]), developed initially in Australia, has passed phase I clinical trials and is slated to begin phase II clinical trials. The vaccine is only suited for patients with celiac disease who possess the HLA-DQ2 haplotype (most patients with celiac disease) because the peptides are only presented by HLA-DQ2; a separate vaccine would have to be formulated for HLA-DQ8–positive patients. The vaccine is administered as a weekly or monthly subcutaneous injection with the goal of allowing patients with celiac disease to resume eating regular or near-normal levels of gluten-containing food without experiencing adverse effects. During the phase I trial, patients who received the highest dose of gluten peptides experienced gastrointestinal distress, inadvertently serving as positive controls, thus confirming that the peptides in the vaccine formulation include key gluten-derived targets. The vaccine is currently in phase II trials.
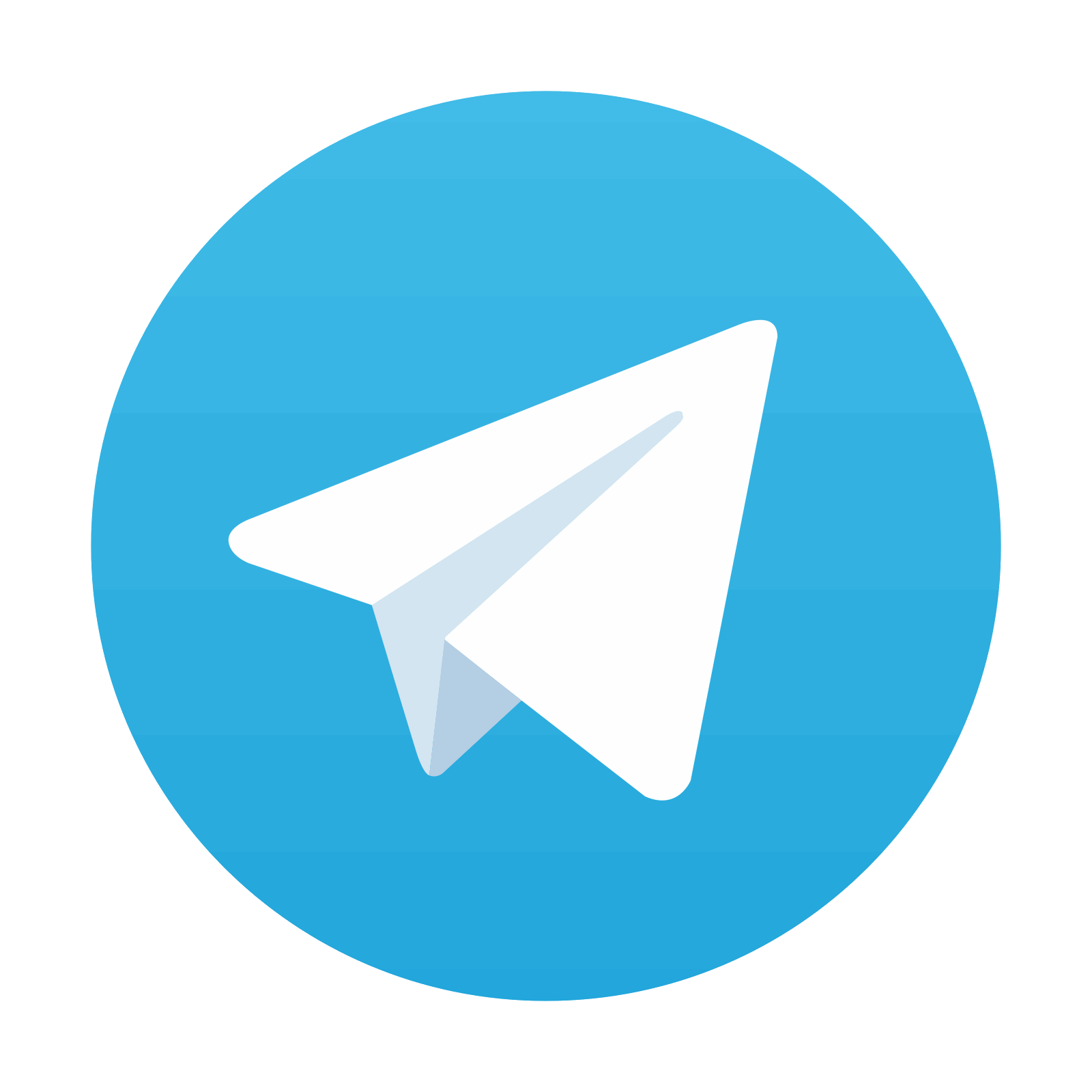
Stay updated, free articles. Join our Telegram channel

Full access? Get Clinical Tree
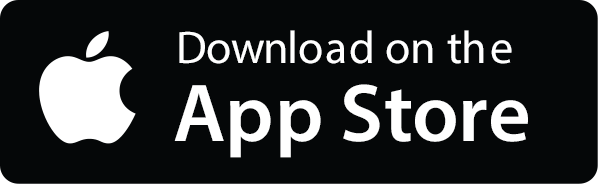
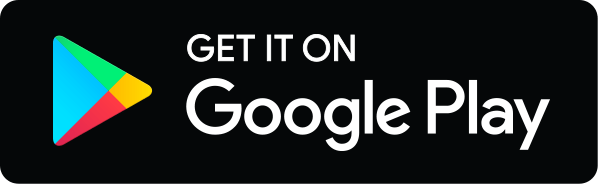