Abstract
The irritable bowel syndrome (IBS) may well reflect a neurological problem emerging from disordered neurophysiology in the enteric nervous system (ENS), the central nervous system (CNS), or in both simultaneously. A normally functioning ENS is essential for a symptom-free gut: the gut does not work at all when the ENS is absent (e.g., Hirschsprung’s disease). This chapter deals mainly with enteric neuroimmune pathophysiology to the exclusion of multiple other explanations, hypotheses and theories, such as small intestinal bacterial overgrowth, gas generating foods, fructose malabsorption, maladjustment to life circumstances, among others that speak to IBS.
Concepts for the neuropathophysiology of the hallmark symptoms of IBS, which include abdominal pain, defecation urgency, bloating, and alterations in stool consistency, emerge in this chapter. Emphasis is focused on gaining acquaintance with the neurophysiology of visceral pain, ENS control of intestinal motility, and ENS control of mucosal secretion as a requirement for productive basic and translational interpretation of the hallmark symptoms.
Mastocytotic enteritis is explored as a biomarker in the context of the diarrhea-predominant form of IBS. This involves release by enteric mast cells of multiple inflammatory mediators that act in paracrine signaling manner at receptors expressed by ENS neurons and intramural sensory afferent terminals. Afferent sensitization amplifies nociceptive input to the CNS and becomes a factor in IBS-related pain.
Enteric mast cells use immunological memory to detect specific foreign antigens as they appear and reappear inside the gut lumen throughout the lifetime of an individual. Paracrine signaling relays the information on foreign antigen identity to the ENS. Paracrine signals, coming from mast cells, are interpreted in turn by the ENS as a labeled code for the presence of an antigenic threat in the intestinal lumen. The ENS interprets the signal as a potential threat to homeostatic integrity and “calls up,” from its program library, secretory and propulsive motor behavior organized for rapid and effectively expulsion of the menace. Commonalty of symptoms between postinfectious IBS and acute psychogenic stress in IBS are likely reflections of neuroimmune communication that includes degranulation of enteric mast cells.
Autoimmune-related enteric neuropathy underlies symptoms in disorders that include lower esophageal sphincter achalasia, intestinal pseudoobstruction, small-cell carcinoma in the lungs, and IBS. Functional symptoms in a subset of IBS patients reflect enteric neuronal damage or ablation related to circulating antienteric autoimmune antibodies.
Keywords
Diarrhea-predominant IBS, Constipation-predominant IBS, Enteritis, Enteric neuropathy, Musculomotor neurons, Secretomotor neurons, Disinhibitory motor disease, Degenerative ENS neuropathy, Paraneoplastic syndrome, Neurogenic secretion, Abdominal pain, Sensory afferents, Postinfectious IBS, Serotonin, Dorsal spinal columns, Cingulate cortex, Spinal sensory wind-up, Ascending spinal pathway, Descend spinal pathway, Intestinal power propulsion, Psychogenic stress, Immuno-neural communication, Enteric mast cells, Brain-gut connection, Histamine, Alosetron
67.1
Irritable Bowel Syndrome
The irritable bowel syndrome (IBS) is a functional disorder present in 10%–20% of worldwide population. A functional disorder is the one for which no abnormal physical or metabolic process can be found to explain patienťs symptoms. Predominant symptoms of IBS are abnormal defecation and abdominal pain, both of which may be exacerbated by emotional stress. Abnormal defecation can be diarrhea, constipation or periodic change from one to the other over time. Urgency to stool and liquid incontinence often accompany the diarrheal state. Patients with the constipation-predominant form of IBS might report bloating, straining, and sensations of incomplete evacuation. A significant subpopulation of IBS patients is hypersensitive to distension of the rectosigmoid region of the large bowel and the hypersensitivity can extend to other regions of the digestive tract, including the esophagus.
Basic gastrointestinal physiology in this chapter addresses normal physiology of the systems from which IBS symptoms emerge. Insightful knowledge of integrated systems physiology is necessary for understanding the pathophysiology that underlies IBS symptoms and for development of rational therapeutic strategies. The systems involved in the defecation-related symptoms are the intestinal secretory glands, the musculature, and the nervous system that controls their activity. Abdominal pain and discomfort, which arise from these systems, fits into the domain of sensory neurophysiology. This chapter aims to examine the pathophysiology of IBS in terms of current concepts related to physiology of intestinal secretion, motility, nervous control, and sensing functions.
67.2
Neuropathy in the Brain-in-the-Gut
Intestinal behavior reflects the integrated function of the musculature, mucosal epithelium, and vasculature. The enteric nervous system (ENS) organizes and coordinates the activity of the three effector systems to generate functionally effective patterns of behavior. The ENS is a local minibrain that contains a library of programs for several different patterns of adaptive intestinal behavior (see Chapter 15 ). Mixing in the digestive state, the migrating motor complex in the interdigestive state and emetic patterns of small intestinal motor behavior are examples of outputs for three of the neural programs in the ENS library. During emesis, the program includes reversal of peristaltic propulsion in the upper jejunum and duodenum to propel rapidly the contents toward the open gastric pylorus and relaxed antrum and corpus.
Structure, function, and neurochemistry of ganglia of the ENS are unlike other autonomic ganglia, most of which function mainly as relay-distribution centers for signals from the central nervous system (CNS). Neurons in the ganglia of the ENS are interconnected to form an independent nervous system with mechanisms for integration and processing of information like those in the brain and spinal cord. Instead of localizing neural control entirely within the CNS, a minibrain has evolved within the walls of the digestive tract distributed close to the effector systems that must be controlled and regulated along several meters of bowel.
The ENS contains as many neurons as the spinal cord. The large number of neurons, required for program control of digestive functions, would greatly expand the CNS if placed there. Rather than packing the neural control circuits exclusively within the skull or vertebral column and transmitting control signals over long unreliable transmission lines to the gut, vertebrate animals have evolved with most of the neural networks, required for automatic feedback control, spatially distributed along the digestive tract in close relationship to the effector systems that must be controlled and integrated for effective whole organ function.
Like the CNS, the ENS has interneurons and motor neurons that are interconnected by chemical synapses into functional neural networks. Also like the CNS, the ENS networks receive input from sensory neurons. The sensory neurons, most of which have their cell bodies in spinal dorsal root ganglia and terminals in the intestinal wall and spinal cord, have receptor regions specialized for detecting changes in thermal, chemical, or mechanical stimulus energy (see Chapter 17 ). The receptor regions transform changes in stimulus energy into signals coded by action potentials that subsequently are transmitted along sensory nerve fibers to be received and processed in the CNS. Interneurons are connected by synapses into networks that process sensory information and control the behavior of motor neurons. Multiple connections among many interneurons use the same mechanisms of chemical neurotransmission as in the CNS. Interneurons form integrative circuits that contain the programs for motor behavioral patterns, such as the migrating motor complex, haustral formation in the colon, and postprandial mixing movements in the small intestine (see Chapter 15 ). Motor neurons are the final common output pathways for transmission of control signals from the interneuronal networks to the effector systems.
67.2.1
Enteric Motor Neurons
The ENS pool of motor neurons includes both excitatory and inhibitory musculomotor neurons. Enteric excitatory musculomotor neurons release neurotransmitters that evoke contraction of the musculature and secretion from mucosal glands. Acetylcholine and substance P are the primary neurotransmitters released by excitatory musculomotor neurons for stimulation of muscle contraction. Acetylcholine, vasoactive intestinal polypeptide, and ATP are excitatory neurotransmitters responsible for evoking secretion from the intestinal glands (i.e., crypts of Lieberkühn).
Enteric inhibitory musculomotor neurons release neurotransmitters that act to suppress contractile activity of the musculature. Vasoactive intestinal polypeptide, nitric oxide, and ATP are among the neurotransmitters implicated as inhibitory neurotransmitters at neuromuscular junctions throughout the digestive tract.
67.2.2
Inhibitory Musculomotor Neurons
Enteric inhibitory musculomotor neurons are a central focus in consideration of ENS neuropathy because their loss is manifest as profound changes in contractile behavior of the intestinal musculature. The changes in motor behavior associated with degeneration of inhibitory motor neurons reflect the specialized physiology of the musculature. The musculature of the digestive tract is described as a self-excitable electrical syncytium consisting of interstitial cells of Cajal (ICCs) that function as pacemakers for the circular muscle coat, which is responsible for generating the contractile forces for propulsion. The term “ electrical syncytium ” implies that action potentials and pacemaker potentials spread by way of gap junctions from smooth muscle fiber to muscle fiber in three dimensions in much the same way as in cardiac muscle. The action potentials trigger contractions as they spread through the musculature. The ICCs are a nonneural pacemaker system of electrical slow waves that are electrically coupled to the musculature and account for the self-excitable characteristics of the muscle (see Chapter 13 ). Within this organization, the electrical slow waves are an extrinsic factor to which the circular muscle responds. Consideration of these physiological characteristics of the musculature evokes the question of why the circular muscle fails to respond with action potentials and contractions to every pacemaker cycle and why action potentials and contractions do not spread in the syncytium throughout the entire length of intestine whenever they occur at any point along the bowel. The explanation is that the circular muscle in a segment of intestine can only respond to invading electrical slow waves from ICCs when the inhibitory motor neurons in the ENS of that segment are inactivated by input from the control circuits formed by interneurons. Likewise, action potentials and associated contractions can propagate only into regions of musculature where the inhibitory motor neurons are inactivated. Consequently, activity of inhibitory musculomotor neurons determines when the omnipresent slow waves initiate a contraction, as well as the distance and direction of propagation once the contraction has begun.
Because some of the inhibitory musculomotor neurons to the circular muscle fire continuously, action potentials and contractions of the muscle are permitted only when the inhibitory neurons are inactivated by input from interneurons in the control circuits. Firing behavior of inhibitory musculomotor neurons to smooth muscle sphincters (e.g., lower esophageal and internal anal sphincter) is opposite to that of the intestinal circular muscle coat. Inhibitory musculomotor neurons to the sphincters are normally quiescent and are switched to firing mode with timing appropriate for coordinated opening of the sphincter with physiological events in adjacent regions. When inhibitory motor neurons fire, they release inhibitory neurotransmitters that relax ongoing muscle contraction in the sphincteric muscle and prevent excitation-contraction in the musculature on either side of the sphincter from spreading into and closing the sphincter.
In nonsphincteric circular muscle, the activity state of the inhibitory innervation determines the length of a contracting segment by controlling the distance of spread of action potentials within the three-dimensional electrical geometry of the smooth muscle syncytium. Contraction can occur in segments in which ongoing inhibition is inactivated while adjacent segments with continuing inhibitory input cannot contract. The boundaries of the contracted segment reflect the transition zone from inactive to active inhibitory motor neurons. The directional sequence in which the inhibitory musculomotor neurons are inactivated establishes the direction of propagation of the contraction. Normally, inhibition is progressively inactivated in the aboral direction, resulting in contractile activity that propagates in the aboral direction. During emesis, the inhibitory motor neurons must be inactivated in a reverse sequence to account for small intestinal propulsion that travels toward the stomach. In general, any treatment or condition that ablates the intrinsic inhibitory neurons results in paroxysmal contractile behavior of the intestinal circular muscle coat.
Several conditions, associated with ablation of enteric inhibitory musculomotor neurons, are associated with conversion from a hypoactive contractile condition of the circular muscle to a hyperactive contractile state. Observations of this nature, commonly made by manometric recording methods in vivo, reinforce the evidence that a subset of the pool of inhibitory motor neurons is tonically active, and that blockade or ablation of these neurons releases the circular muscle from the inhibitory influence. The behavior of the muscle in these cases is tonic contracture and recurring disorganized phasic contractile activity reminiscent of fibrillation in the myocardium.
67.2.3
Disinhibitory Motor Disease
The physiology of inhibitory neuromuscular relations in the intestine predicts that spasticity and “achalasia” (i.e., failure to relax) will accompany any condition where inhibitory musculomotor neurons are destroyed. Without ENS inhibitory neural control, the self-excitable smooth muscle contracts continuously and behaves as an obstruction. This happens because the muscle is freed to respond to the pacemaker ICCs (i.e., electrical slow waves) with contractions that propagate without amplitude, distance, or directional control. Contractions spreading in the uncontrolled syncytium collide randomly resulting in chaotic-ineffective behavior in the affected intestinal segment.
Loss or malfunction of inhibitory musculomotor neurons is the pathophysiological starting point for disinhibitory motor disease, which includes several forms of chronic intestinal pseudoobstruction and sphincteric achalasia. Neuropathic degeneration of the ENS includes loss of inhibitory musculomotor neurons and is a progressive disease that in its early stages may be manifest as symptoms that could be interpreted as a functional gastrointestinal disorder like IBS.
Intestinal pseudoobstruction is a pathophysiological condition in which the symptoms resemble those of a mechanical obstruction to forward propulsion, but without the presence of a mechanical obstruction (e.g., intussusception and strictures). Chronic intestinal pseudoobstruction can be myopathic or neuropathic. The neuropathic form of chronic intestinal pseudoobstruction is a form of disinhibitory motor disease linked with neuropathic degeneration in the ENS. Failure of propulsive motility in the affected length of bowel reflects loss of the neural microcircuits that program and control the repertoire of motility patterns required for the necessary functions of that region of bowel. Pseudoobstruction occurs in part because contractile behavior of the circular muscle is hyperactive but disorganized in the denervated regions. Hyperactivity determined manometrically in vivo is a diagnostic sign of the neuropathic form of chronic small bowel pseudoobstruction. The hyperactive and disorganized contractile behavior reflects the absence of inhibitory nervous control of the muscles that are autogenic when released from the braking action imposed by the inhibitory musculomotor neurons of the ENS. Chronic pseudoobstruction is therefore symptomatic of the advanced stage of a progressive enteric neuropathy and underscores a fact that propulsive motility does not occur in the absence of the ENS. Retrospective review of patient histories suggests that some IBS-like symptoms can be an expression of early stages of ENS neuropathy.
Degenerative noninflammatory and inflammatory ENS neuropathies are two kinds of disinhibitory motor disorders that culminate in pseudoobstruction. Noninflammatory neuropathies can be either familial or sporadic. The mode of inheritance can be autosomal recessive or dominant. In the former, the neuropathological findings include a marked reduction in the number of neurons in both myenteric and submucosal plexuses, and the presence of round, eosinophilic intranuclear inclusions in about 30% of the residual neurons. Histochemical and ultrastructural evaluations reveal the inclusions not to be viral particles, but rather proteinaceous material forming filaments that is in some ways are reminiscent of Alzheimer’s disease in the brain. Members of two families have been described with intestinal pseudoobstruction associated with the autosomal dominant form of ENS neuropathy. The number of enteric neurons are decreased in these patients, with no alterations found in the CNS or parts of the autonomic nervous system outside the gut.
Degenerative inflammatory ENS neuropathies are characterized by a dense inflammatory infiltrate confined to enteric ganglia. Paraneoplastic syndrome, Chagas disease, and idiopathic degenerative disease are recognizable forms of pseudoobstruction related to inflammatory neuropathies.
Paraneoplastic syndrome is a form of pseudoobstruction where commonality of antigens between small-cell carcinoma of the lungs and enteric neurons leads to autoimmune attack, which results in the loss of neurons. Most patients with symptoms of pseudoobstruction in combination with small-cell lung carcinoma have immunoglobulin-G autoantibodies that react with their enteric neurons. These antibodies react with a variety of molecules expressed on the nuclear membrane of neurons, including Hu and Ri proteins, and with cytoplasmic antigens (Yo protein) of Purkinje cells in the cerebellum. Immunostaining with sera from paraneoplastic patients shows a characteristic pattern of staining in enteric neurons. The detection of antienteric neuronal antibodies is a means to a specific diagnosis. Induction of apoptosis is involved in the mechanisms by which the antibodies damage the neurons.
Association of enteric neuronal loss and symptoms of pseudoobstruction in Chagas disease, like paraneoplastic syndrome, reflects autoimmune attack on the neurons with accompanying symptoms that mimic the situation in sphincteric achalasia and paraneoplastic syndrome. Trypanosoma cruzi , the blood-borne parasite that causes Chagas disease, has antigenic epitopes similar to enteric neuronal antigens. This antigenic commonality activates the immune system to assault the ENS coincident with its attack on the parasite.
Idiopathic inflammatory degenerative ENS neuropathy occurs unrelated to neoplasms, infectious conditions, or other known diseases. De Giorgio et al. and Smith et al. described two small groups of patients with early complaints of IBS-like symptoms that progressively worsened and were later diagnosed as idiopathic degenerative inflammatory neuropathy based on full-thickness biopsies taken during exploratory laparotomy, which revealed chronic intestinal pseudoobstruction. Each patient had inflammatory infiltrates localized to the myenteric plexus. Sera from the two cases reported by Smith et al. contained antibodies against enteric neurons similar to those found in secondary inflammatory neuropathies (i.e., anti-Hu), but with different immunolabeling patterns characterized by prominent cytoplasmic rather than nuclear staining.
Recognition of the brain-like functions of the ENS leads to a conclusion that early neuropathic changes are expected to be manifest as symptoms that worsen with progressive neuronal loss. In diagnostic motility studies (e.g., manometry), degenerative loss of enteric neurons is reflected by hypermotility and spasticity because inhibitory musculomotor neurons are included in the missing neuronal population.
67.2.4
Enteric Autoimmune Neuropathy
Serum from a large group of IBS patients (84% vs. non-IBS controls) were found to contain high titers of antienteric neural antibodies reminiscent of those in paraneoplastic syndrome and Chagas disease. Antibody immunostaining occurred in the nucleus and cytoplasm of ENS neurons in the IBS patients. Protein microarray analysis detected antibody reactivity for autoantigens in serum with antienteric neuronal antibodies and no reactivity for the same autoantigens in samples not containing antienteric neuronal antibodies. The antibodies in the sera from the IBS patients recognized only three antigens out of an 8000 immunoprotein array. The three antigens were as follows: (1) a nondescript ribonucleoprotein (RNP-complex); (2) small nuclear ribonuclear polypeptide A; and (3) Ro-5200-kD, which is a biomarker for Sjögrens syndrome. These findings suggest that IBS symptoms in a subset of patients might reflect enteric neuronal damage or loss in the ENS caused by circulating antienteric autoimmune antibodies. Early stages of the autoimmune neuropathy appear to be expressed as IBS-like symptoms that progress to symptoms of chronic intestinal pseudoobstruction in parallel with progressive loss of neurons from the ENS ( Fig. 67.1 ).

As discussed above, individual patients that have been treated for IBS symptoms for several years, present with signs of mechanical intestinal obstruction. Exploratory laparotomy then confirms a diagnosis of intestinal pseudoobstruction and full-thickness biopsy specimens show ENS ganglionitis. This raises the following question: if the IBS-like symptoms and eventual pseudoobstruction reflect an autoimmune enteric neuropathy, why do patients have IBS symptoms for a long time before presenting with pseudoobstruction? The answer may be that omnipresent neuronal stem cells divide and give rise to daughter cells that differentiate into one or the other of the specific classifications of ENS neurons and thereby replace neurons killed by autoimmune attack. Replacement “wiring” of newly minted neurons into their synaptic networks may repair the networks and restore their functionality. Neurogenesis of this nature is known to be ongoing continuously in specific regions of the adult brain where the new neurons become integrated into functional microcircuitry. Evidence that a comparable phenomenon occurs in the ENS has begun to emerge (see Chapter 12 ).
67.3
Neurogenic Secretion: Diarrhea and Constipation
Disordered defecation in IBS is related directly to the physiology of enteric secretomotor neurons. Secretomotor neurons are excitatory motor neurons in the submucosal plexus of the ENS, which innervate and stimulate secretion from the intestinal secretory glands ( Fig. 67.2 ).

67.3.1
Secretomotor Neurons
Secretomotor neurons have a single axon with characteristic shapes described as Dogiel Type I and electrophysiological behavior like S-type enteric neurons (see Chapter 14 ). When they fire, they release acetylcholine and vasoactive intestinal polypeptide at the neuroepithelial junctions.
Collateral projections from secretomotor axons innervate submucosal arterioles ( Fig. 67.2 ). The collateral innervation of the blood vessels links secretomotor activity in the crypts to submucosal blood flow. Active firing of secretomotor neurons releases acetylcholine simultaneously at neuroepithelial and neurovascular junctions. Acetylcholine acts at the blood vessels to release nitric oxide from the endothelium, which in turn dilates the vessels and increases blood flow in support of stimulated secretion.
Secretomotor neurons have receptors that receive excitatory and inhibitory synaptic input from other neurons in the integrative circuitry of the ENS and from sympathetic postganglionic neurons. Their excitability can be greatly influenced also by paracrine messages from nonneural cell types, such as enterochromaffin cells and immune/inflammatory cells in the mucosa and submucosa. Activation of the excitatory receptors on secretomotor neurons stimulates the neurons to fire and release transmitters at their junctions with the glandular epithelium and regional blood vessels. The overall result of secretomotor neuronal firing is stimulation of secretion of H 2 O, NaCl, bicarbonate, and mucus from the intestinal glands.
67.3.2
Inhibitory Input to Secretomotor Neurons
Inhibitory inputs from other neurons hyperpolarize the membrane potential of secretomotor neurons which decreases the probability of firing ( Fig. 67.2 ). The physiological effect of inhibiting secretomotor firing is suppression of mucosal secretion.
Postganglionic neurons of the sympathetic nervous system are an important source of inhibitory input to the secretomotor neurons. Norepinephrine released from sympathetic nerve terminals in the submucosal plexus acts at alpha 2 noradrenergic receptors to inhibit firing of the secretomotor neurons. Inhibition of secretomotor firing reduces the release of excitatory neurotransmitters at the junctions with epithelial cells of the crypts. The result is reduced secretion of water and electrolytes. Suppression of secretion in this manner is part of the mechanism involved in sympathetic nervous shutdown of gut function in homeostatic states where blood is shunted from the splanchnic to systemic circulation.
67.3.3
Neurogenic Secretory Diarrhea and Neurogenic Constipation
Knowledge of the neurobiology of submucosal secretomotor neurons is central to understanding the pathophysiology of secretory diarrhea, as well as constipation. In general, secretomotor hyperactivity is associated with neurogenic secretory diarrhea; hypoactivity is associated with decreased secretion and a constipated state. Suppression of secretomotor firing by antidiarrheal agents, such as opiates, clonidine, and somatostatin analogs, is manifest as harder-drier stools. Stimulation by chemical mediators, such as vasoactive intestinal peptide (VIP), serotonin and histamine, is manifest as liquid or semisolid stools.
Watery diarrhea may be caused by several different pathophysiological events. For example, secretomotor neurons may be overly stimulated by circulating VIP released from VIPomas, excessive serotonin release from mucosal enterochromaffin cells or histamine release from inflammatory/immune cells in the mucosa/submucosa. Release of histamine or other inflammatory mediators associated with diarrhea not only stimulates the firing of secretomotor neurons but also simultaneously acts at presynaptic inhibitory receptors to suppress the release of norepinephrine from the postganglionic sympathetic axons that provide inhibitory input to secretomotor neurons. Thus, two pathological factors are involved in the production of neurogenic secretory diarrhea. One is over stimulation of secretomotor neuronal firing and the other is presynaptic suppression of norepinephrine from sympathetic postganglionic neurons that results in removal of the sympathetic braking action on the secretomotor neurons ( Fig. 67.2 ).
67.3.4
Neurogenic Constipation
Treatment of pain with morphine and allied opioid agonists has constipating side effects. Opiates and opioid drugs silence secretomotor neuronal firing and stimulation of secretion by hyperpolarizing the membrane potential of the neurons. Suppression of secretomotor firing reduces secretion, which in concert with delayed intestinal transit, results in lowered liquidity of the small and large intestinal contents. Drier-harder stools are the result.
Lubiprostone ( Amitiza ) is a drug approved for treatment of adult idiopathic constipation and constipation-predominant IBS in adult women and for reversal of the constipating effects of opioid pain killers. Lubiprostone works by directly opening chloride (Cl − ) channels in the small and large intestinal epithelium and bicarbonate (HCO 3 − ) secretory channels in the duodenum. Water and sodium (Na + ) follow Cl − into the lumen and this increase the liquidity and softens the contents, all of which is beneficial for constipation. Bicarbonate secretion in the duodenum is an important component of the physiological control of optimal pH for digestion and mucosal cytoprotection against acid in the stomach.
67.3.5
Excitatory Input to Secretomotor Neurons
Secretomotor neurons have excitatory receptors for several neurotransmitters, which include acetylcholine, VIP, substance P, and serotonin ( Chapter 19 ). The serotonergic receptors belong to the 5-HT 3 receptor subtype. Efficacy of blockade of 5-HT 3 receptors by a 5-HT 3 antagonist in the treatment of diarrhea in the diarrhea-predominant population of women with IBS suggests that over stimulation of secretomotor neurons by serotonin is a significant factor in this form of IBS ( Fig. 67.3 ). Observations that IBS symptoms of cramping abdominal pain, diarrhea, and fecal urgency are commonly exacerbated in the postprandial state and evidence for elevated postprandial release of serotonin raise suspicion that overactive release of serotonin from the enterochromaffin cell population might underlie the diarrheal symptoms of IBS. This is reinforced by suggestions of elevated numbers of serotonin-containing enterochromaffin cells, as well as mast cells in the mucosa of IBS patients. Moreover, enteric mast cells express the 5-HT1A receptor subtype, stimulation of which degranulates mast cells and releases mediators that stimulate secretomotor neurons and elevate secretion in the colon.

67.4
Abdominal Pain and Discomfort
Primary causes of abdominal pain of digestive tract origin are distension and exaggerated muscular contraction. Stimuli such as pinching and burning of the mucosa applied via fistulae or mucosal biopsy procedures do not evoke pain. Hypersensitivity of the mechanosensors for stretch (distension) and contractile tension are implicated as pain factors in IBS. Hypersensitivity to distension is present in a substantial subset of IBS patients. Filling of a balloon placed in the rectosigmoid region evokes sensations of discomfort and pain at lower distending volumes for IBS patents than for normal subjects ( Fig. 67.4 ). Hypersensitivity to distension is not restricted to the distal bowel. Patients, who are diagnosed with functional dyspepsia, also experience discomfort and pain at lower distending volumes in the stomach that normal subjects and hypersensitivity to distension is present in the esophagus of patients with noncardiac chest pain. IBS patients experience the same kind of hypersensitivity to electrical stimulation applied to the lining of the rectosigmoid region as they do during balloon distension in the rectosigmoid. The hypersensitivity to direct electrical stimulation of the sensory innervation implicates abnormal sensory neurophysiology rather than mechanical factors (e.g., wall tension and compliance) as underlying the decreased sensory thresholds present in IBS patients. Identification of the sensory defect is an area of current active investigation and is yet to be determined satisfactorily. The lower sensory threshold in IBS might reflect: (1) sensitization of intramural mechanoreceptor endings; (2) sensitization of neurotransmission in the spinal cord; (3) abnormal processing of arriving sensory information in the brain; and (4) a combination of all three possibilities.

67.4.1
Peripheral Neuropathological Factors
Mechanosensitive spinal afferents in the walls of the specialized organs of the digestive tract detect and signal contraction of the musculature and distension of the visceral wall (see Chapter 17 ). The mechanosensing structures at the afferent terminals behave as if they are attached “in-series” with the long axes of the smooth muscle fibers ( Fig. 67.5 ). The in-series arrangement accounts for activation of the sensors by either muscle contraction or distension. The cell bodies of the neurons that give rise to intestinal afferents are in nodose ganglia and dorsal root spinal ganglia ( Fig. 67.6 ). Mechanosensitive information is transmitted along spinal afferents to the spinal cord by way of dorsal root ganglia and along vagal sensory afferents to the brain stem by way of the nodose ganglia.


Vagal and spinal afferent fibers are predominantly unmyelinated C fibers or thinly myelinated Aδ-fibers, which transmit different modalities of sensory information at low conduction velocity. In general, vagal afferents transmit physiological information on the nature and composition of the luminal contents, the presence or absence of motility and on contractile tension in the musculature, while spinal afferents transmit pathophysiological information related to potentially noxious mechanical or chemical stimuli arising through tissue injury, ischemia, or inflammation. Sensations of pain and discomfort of digestive tract origin reflect transmission in spinal afferents and information processing in the spinal cord and brain. Sensory information transmitted by the vagus nerves appears not to reach the level of conscious sensation because patients with high spinal cord transections and intact vagal pathways experience little or no sensations of digestive tract origin. On the other hand, the persistence of conscious perception of sensations from the digestive tract following a surgical vagotomy (e.g., as outdated treatment for peptic ulcer disease), reflects transmission over spinal afferents.
The streams of mechanosensory information that are transmitted from the small and large intestine to the CNS are generated by low-threshold, high-threshold and silent afferents (see Chapter 17 ). Low-threshold afferents respond to weak changes in wall tension and are thought to transmit the minute-to-minute information required for functional autonomic negative-feedback control. High-threshold afferents require stronger changes in wall tension, which might be produced by distension of the lumen or strong contraction of the musculature, for activation. The high-threshold afferents are postulated to be responsible for the range of sensations from mild to severe pain that are associated with excessive distension or exceptionally strong muscle contraction. Silent afferents are of pathophysiological interest because they become active and highly sensitive to stimuli during inflammatory states.
67.4.2
Spinal Afferents Innervate Enteric Mast Cells
Enteric mast cells express substance P NK1 receptors and calcitonin gene-related peptide (CGRP) receptors in guinea pig and human small intestine. Stimulation of intramural afferents by antidromic electrical stimulation of mesenteric nerve bundles or by capsaicin releases substance P and CGRP inside human and guinea pig intestinal segments in vitro. Backfiring of the afferents, in this manner, evokes slow excitatory postsynaptic potentials mediated also by tachykinin NK1 and CGRP receptors in ENS interneurons. Stimulation of intramural afferent endings by capsaicin evokes slow EPSP-like responses that mimic the responses to backfiring of afferents leaving the intestine in mesenteric nerves. These responses are suppressed by antagonists that bind to protease-activated receptor II.
Mast cell stabilizing drugs suppress slow EPSP-like excitation evoked by stimulation of the intramural afferent fibers. Histamine and mast cell protease II, in these intestinal preparations, are released by (1) exposure to substance P or CGRP; (2) capsaicin; (3) compound 48/80; (4) elevation of mast cell Ca 2 + by ionophore, A23187; (5) antidromic electrical stimulation of afferents leaving the gut in mesenteric nerves. The mast cell stabilizers, cromolyn or doxantrazole, suppresses release of protease II and histamine when evoked by substance P, CGRP, capsaicin, A23187, electrical stimulation of afferents, or compound 48/80. Neural blockade by tetrodotoxin prevents mast cell protease II release in response to antidromic electrical stimulation of mesenteric afferents. These findings support a hypothesis that afferent innervation of enteric mast cells releases histamine and mast cell protease II, both of which are known to act in diffuse paracrine manner to influence the behavior of ENS neurons and to elevate the sensitivity of spinal afferent terminals.
Immunohistochemistry confirms coexpression of immunoreactivity for vanilloid receptor TRPV1, substance P and CGRP in small diameter intramural fibers in the ENS and identifies them as primary spinal afferents. Retrograde tracing results show that spinal afferents in mesenteric nerve trunks enter the intestinal wall and branch to send projections into ENS ganglia ( Fig. 67.7 ). Inside the ganglia, some of the afferents appear to form synapses with neuronal cell bodies, while others have specialized terminals that discharge action potentials in response to mechanical stimulation in like manner to mechanoreceptors elsewhere. Connections of this nature might be indicative of local axon reflex connections between afferent collaterals and enteric neurons, as well as other cell types such as occurs in the classical “triple response of Lewis,” which like the case for enteric mast cells, involves afferent-evoked release of histamine from cutaneous mast cells ( Fig. 67.7 ).

Histamine and mast cell protease II when released from enteric mast cells diffuse in paracrine manner, to influence the behavior of ENS neurons (see Section 15.3.5 ) and to elevate the sensitivity of spinal afferent terminals to their preferred modality. A heuristic model suggests that sensitization and elevated firing frequencies of the afferent terminals feed forward to amplify mast cell release, which further sensitizes the terminal and continues a forward feed that intensifies actions in the ENS and sensory input to the spinal cord. Intensified input to the spinal cord, if from nociceptors, might be interpreted as pain and/or discomfort at the level of consciousness ( Figs. 67.6 and 67.7 ).
67.4.3
Postinfectious IBS
A significant proportion of patients develop IBS-like symptoms following an acute bout of infectious enteritis. Hypochondriasis and adverse life events are reported to double the risk for development of postinfective IBS. Nevertheless, the question of whether the association between acute infectious enteritis and IBS reflects low-level inflammation (e.g., microscopic enteritis) and chronic exposure of the neural and glial elements of the ENS to elevated levels of serotonin, histamine, mast cell proteases, or inflammatory mediators is yet to be fully resolved.
67.4.4
Serotonin Receptors on Sensory Afferents
Application of 5-HT evokes increased firing in extrinsic afferents via 5-HT 3 receptors that can be blocked by selective antagonists, such as alosetron. Intramural terminals of both spinal and vagal sensory nerves express 5-HT 3 receptors (see Figs. 67.6 and 67.7 ). Reported efficacy of alosetron in the treatment of abdominal pain and discomfort in the diarrhea-predominant form of IBS in women suggests that these symptoms might reflect overactive endogenous release and elevated levels of 5-HT at receptors on ENS neurons, such as secretomotor neurons, or on immune/inflammatory cells, such as enteric mast cells. Persistence of 5-HT at its receptors, due to defective reuptake by the serotonin transporter (SERT), is a second possibility for hyperstimulation of intramural serotoninergic receptors. Active uptake mediated by SERT, which is expressed by enteric neurons and the mucosal epithelium, restricts accumulation and action of 5-HT at its receptors following its release. Downregulation of transporter mRNA expression and expression of immunoreactivity for the serotonin transporter were reported to be present in mucosal biopsies taken from the large intestine of IBS patients. Watery stools and enhanced propulsive motility occurred in mice with a deletion in the serotonin transporter gene and the mice sometimes alternated between diarrhea and constipation in a way reminiscent of the subset of IBS patients that are classified as “alternators.” Association between functional polymorphisms in SERT and the SERT gene (SLC6A4) and IBS materialized as an appealing hypothesis in view of efficacy of selective SERT inhibitors in low doses in treatment of IBS. Nevertheless, association of the SERT gene promoter polymorphism 5-HTTLPR with IBS and the expression pattern of SERT in the intestinal mucosa of IBS patients are conflicting and unresolved.
67.4.5
Receptors for Inflammatory Mediators on Sensory Afferents
Alongside 5-HT 3 receptors are receptors for bradykinin, ATP, adenosine, prostaglandins, leukotrienes, and mast cell proteases expressed on spinal sensory nerve terminals. These are mediators released in paracrine manner, any one of which has potential for elevating the sensitivity of intestinal sensory nerves, especially in disordered conditions of inflammation or ischemia. Findings that a reduced threshold for painful responses to balloon distension in the large bowel is associated with degranulation of mast cells in animal models supports this possibility. Treatment with mast cell stabilizing drugs prevents the lowering of the pain threshold during mucosal inflammation in animal models and suggests that mast cell stabilization might turn out to be an efficacious treatment in human IBS.
67.4.6
Serotonergic Receptors on Enteric Mast Cells
Serotonin, released from enterochromaffin cells in the mucosa of the small intestine, elevates 5-HT concentrations in the environment of enteric mast cells, degranulates the mast cells and releases mediators that become paracrine signals to the ENS, spinal afferents, and secretory glands. Mast cells in human and guinea pig preparations express the 5-HT 1A receptor subtype and spontaneous release of histamine can be quantified in guinea pig and human small intestinal preparations. The selective 5-HT 1A receptor agonist, 8-hydroxy-PIPAT, evokes release of histamine in the small intestine. A selective 5-HT 1A receptor antagonist, WAY100135, suppresses this stimulation of histamine release by 5-HT or 8-hydroxy-PIPAT. The Mast cell stabilizing drugs, doxantrazole and cromolyn sodium, suppress the release of histamine evoked by 5-HT or 8-hydroxy-PIPAT in guinea pig and human preparations. The observations support a hypothesis that serotonergic degranulation of enteric mast cells and release of preformed mediators, including histamine, is mediated by the 5-HT 1A serotonergic receptor subtype. Association of serotonin with the pathophysiology of IBS and other functional gastrointestinal disorders prompts a question of whether selective 5-HT 1A receptor antagonists might have therapeutic application in disorders of this nature.
67.4.7
Central Neuropathological Factors
Much of the evidence points to altered sensory transduction as the underlying cause of the exaggerated sensitivity to distension found in IBS patients. Intramural spinal afferents become hypersensitive to mechanical stimuli and thus transmit nociceptive information to the CNS. On the other hand, there might be cases where normally functioning mechanosensors transmit accurate information, which is misinterpreted in processing circuits in the spinal cord and/or brain to evoke conscious perceptions of abnormal sensations from the gut.
67.4.8
Ascending Sensory Pathways in the Spinal Cord
Canonical ascending spinal pathways involved in the transmission of nociceptive signals from the digestive tract are the spinothalamic, spinohypothalamic, spinosolitary, spinoreticular, and spinoparabrachial tracts ( Fig. 67.8 ). A pathway in the dorsal spinal columns is a transmission conduit for visceral pain information to higher processing centers in the brain. Spinal afferents, which transmit nociceptive information, provide direct synaptic input to second-order neurons in the dorsal column nuclei (i.e., nucleus gracilis and nucleus cuneatus). Second-order neurons in the spinal dorsal horn also provide input to the dorsal column nuclei ( Fig. 67.8 ). From the dorsal column nuclei, the pain signals are transmitted via the ipsilateral dorsal column nuclei to the contralateral ventroposterolateral nucleus of the thalamus. This pathway in the dorsal columns may be more important in visceral nociceptive transmission than the spinothalamic and spinoreticular tracts.

A midline myelotomy that severs axons in the human dorsal columns attenuates otherwise intractable visceral pain. Stimulation of the dorsal columns in patients with severe IBS evokes an immediate increase in the intensity of their abdominal pain. These clinical observations in humans are consistent with experimental results in animals. Severing the axons of the dorsal columns in rats or monkeys reduces the neuronal responses to colorectal distension in the ventral posterolateral nucleus of the thalamus and of neurons in the dorsal column nuclei, particularly in the nucleus gracilis.
67.4.9
Sensory Processing in the Cerebral Cortex
Modern methods of brain imaging provide insight into questions related to abnormal processing of sensory information in the cerebral cortex of IBS patients. Functional magnetic resonance imaging (fMRI) and positron emission tomography (PET) are the methods used to study information processing in the higher brain centers that underlie an individual’s experience of conscious sensations ( Chapter 16 ).
Results from imaging studies suggest that unlike somatic sensation, which has a main homuncular representation in the primary somatosensory cortex, visceral sensation is mainly represented in the secondary somatosensory cortex. Differences in processing in these specialized regions might account for the vagueness of one’s ability to localize visceral sensation in relation to somatic sensation. Beyond the sensory cortices, fMRI and PET images show representation of both somatic and visceral sensation to be similar in the limbic and paralimbic regions of the cortex (e.g., anterior insular, anterior and posterior cingulate, prefrontal and orbitofrontal cortices ). These areas are known to be involved in the individual’s motivational and emotional mood states and in the cognitive components of visceral sensations.
Gender differences are noticeable in the cortical representation of balloon distension in the rectosigmoid region in healthy subjects. While activation in the sensory/motor and parieto-occipital areas does not differ between genders, more extensive activation appears in the anterior cingulate and prefrontal cortices in women. The volume of evoked cortical activity in females is greater than in men for perception levels in the range from the urge to defecate to sensations of fullness, mild discomfort and pain. The functional significance of these gender differences is unclear; nevertheless, they are reminiscent of the greater perceptual responses reported in female patients with functional gastrointestinal disorders (e.g., IBS).
In healthy subjects, either painful distension by a balloon in the rectosigmoid region or anticipation of a potentially painful distension is associated with increased blood flow in the anterior cingulate cortex. In IBS patients, activation of the anterior cingulate cortex was reported not to occur in response to painful distension or the anticipation of painful distension of the balloon in response to a warning from the investigator. On the other hand, an fMRI study found that patients with IBS showed enhanced activation of the midcingulate cortex in response to rectal distension. Selective activation of the prefrontal cortex appeared to take place coincident with decreased activation of the anterior cingulate cortex in the study of Silverman et al. The cingulate cortex attracts attention because it is generally thought to be an integrative center for both emotional experience and specific representation for pain, which might account for linkage of pain and emotional state. This cortical region is formed around the rostrum of the corpus callosum and has projections into the motor regions of the cortex ( Fig. 67.9 ). The “affective” areas of the cingulate cortex have extensive connections with the amygdala and periaqueductal gray matter and with autonomic nuclei in the brain stem. The cingulate cortex integrates autonomic and endocrine functions and is involved in recall of emotional experiences. Cognition appears to reside in the caudal portion of the anterior cingulate cortex together with circuits delegated to premotor functions and processing of nociceptive information.

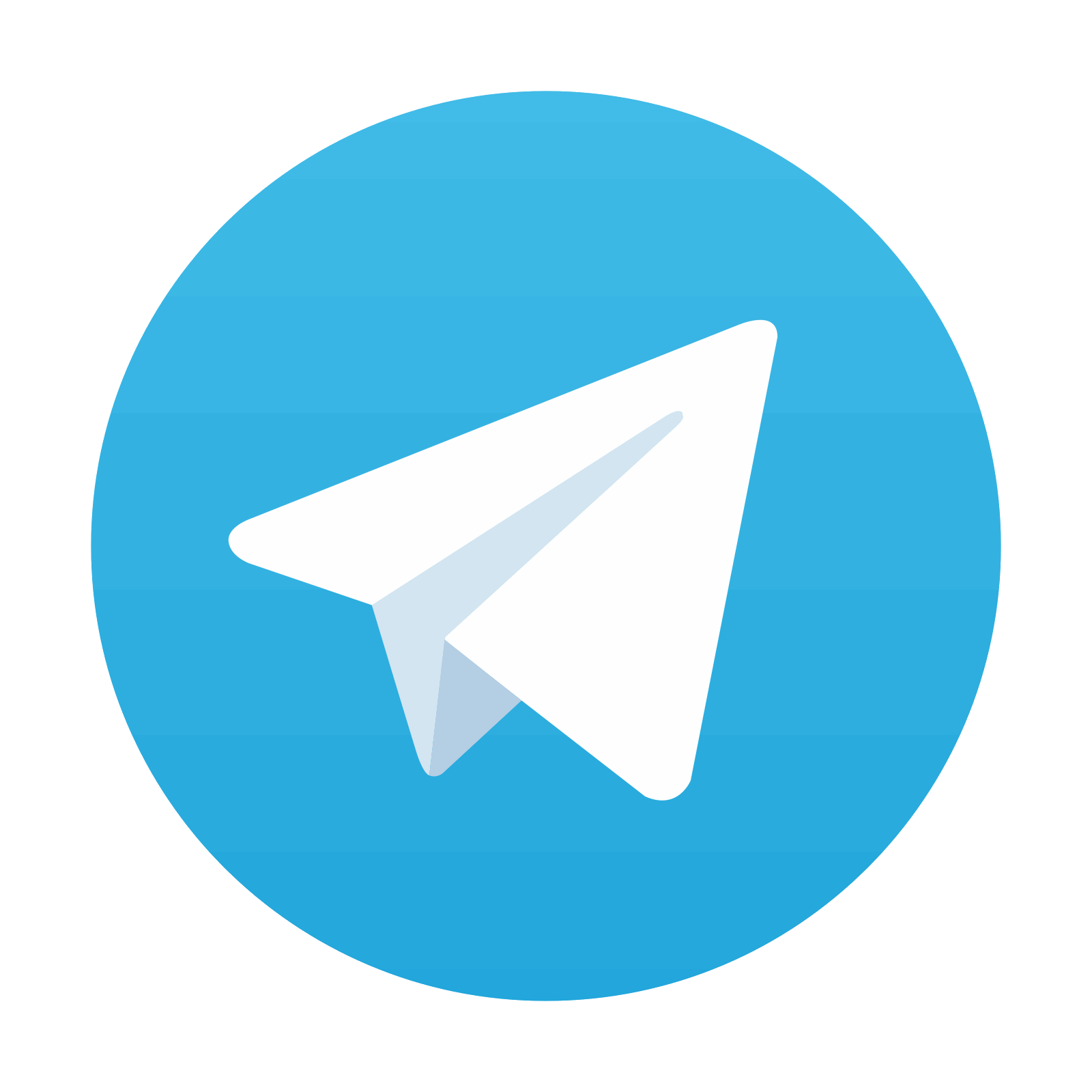
Stay updated, free articles. Join our Telegram channel

Full access? Get Clinical Tree
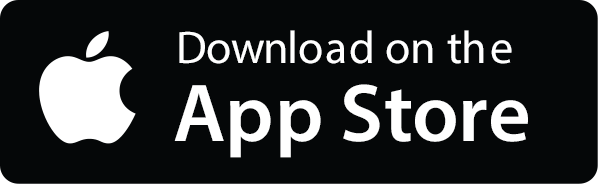
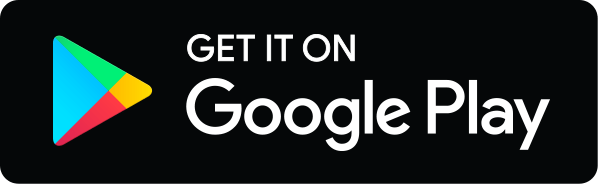
