© Springer International Publishing Switzerland 2017
Kenneth L. Koch and William L. Hasler (eds.)Nausea and Vomiting10.1007/978-3-319-34076-0_77. Nausea and Vomiting Related to Autonomic Nervous System Disorders
(1)
Division of Gastroenterology, Hepatology, and Nutrition, University of Louisville School of Medicine, 550 S. Jackson Street, [ACB A3L15], Louisville, KY 40202, USA
Keywords
Autonomic nervous systemNauseaVomitingCyclic vomiting syndromePostural orthostatic tachycardia syndromeOrthostatic intoleranceGastric electrical stimulationAdrenergic receptorCholinergic receptorEnteric nervous systemAbbreviations
5-HT
5 Hydroxy tryptamine
Ach
Acetylcholine
AFT
Autonomic function testing
ANS
Autonomic nervous system
CAN
Cardiovascular autonomic system
CCK
Cholecystokinin
CINV
Chemotherapy-induced nausea and vomiting
CNS
Central nervous system
CRF
Corticotropin-releasing factor
CTZ
Chemoreceptor trigger zone
CVS
Cyclical vomiting syndrome
DAN
Diabetic autonomic neuropathy
DMV
Dorsal motor nucleus
ENS
Enteric nervous system
GABA
γ-Aminobutyric acid
GI
Gastrointestinal
HPA
Hypothalamic-pituitary-adrenal
HRV
Heart rate variability
HUT
Head-up tilt
ICC
Interstitial cells of Cajal
IGP
Intragastric pressure
LES
Lower esophageal sphincter
NE
Norepinephrine
NK
Neurokinin
NMH
Neurally mediated hypotension
nNOS
Neuronal nitric oxide synthase
NO
Nitric oxide
NTS
Nucleus tractus solitarius
OI
Orthostatic intolerance
PNS
Peripheral nervous system
POTS
Postural orthostatic tachycardia syndrome
QSART
Quantitative sudomotor axon reflex test
T1DM
Type 1 diabetes mellitus
T2DM
Type 2 diabetes mellitus
TSS
Total symptom score
TST
Thermoregulatory sweat test
VIP
Vasoactive intestinal polypeptide
Introduction
The functions of the gastrointestinal (GI) tract are independent of voluntary control with exception of the initiation of swallowing and control of the external anal sphincter. Autonomic GI functions are controlled by the intrinsic enteric nervous system (ENS) and the extrinsic neural systems (sympathetic and parasympathetic nervous systems) via the autonomic nervous system (ANS). Thus, it is not surprising that disorders of the autonomic nervous system can manifest in a wide variety of GI symptoms. ANS disorders that affect the GI tract primarily affect the neuromuscular functions rather than sensory or secretory functions [1].
Orthostatic intolerance (OI) including postural orthostatic tachycardia syndrome (POTS) is one of the most common autonomic disorders; it has a wide variety of GI manifestations ranging from nausea and vomiting to abdominal pain and constipation [2–4]. Treatment of the underlying orthostatic intolerance was found to resolve the GI symptoms [5]. Chronic intestinal pseudo-obstruction is associated with nausea and vomiting and shows many associated features suggestive of autonomic neuropathy [1]. Patients with gastroparesis exhibit features of autonomic neuropathy. Human studies have shown increased sympathetic activity in nausea and vomiting caused by vestibular stimulation [6, 7]. Chemotherapy-induced nausea and vomiting (CINV) was associated with abnormalities on autonomic testing [8]. In a study of 8 children with functional abdominal pain, abnormalities in autonomic function testing (orthostatic intolerance and abnormal sweat test) were noted in at least 75 % of patients [9]. Conversely, patients with dysautonomia of various etiologies have associated nausea and vomiting. Most of the antiemetic agents, especially the newer ones like 5-HT3 antagonists and NK1 antagonists, may act in part via autonomic pathways. Disorders of the ANS can be associated with GI manifestations of nausea and vomiting. Some examples of these include orthostatic intolerance, POTS, cyclical vomiting syndrome (CVS), gastroparesis, migraines, and familial dysautonomia.
Overview of the ANS
The ANS is a part of the peripheral nervous system (PNS) and controls involuntary body functions. The ANS innervates all the organs in the body; it regulates respiration, the cardiovascular system including vasomotor activity, thermal regulation, abdominal and pelvic organs, glands, skin, iris of the eyes, and some reflexes. The primary function of the ANS is to maintain internal homeostasis in response to changes in the environment. ANS function is mediated via centrally integrated responses as well as local and regional reflexes initiated via the organ or the environment. Afferent neurons receive the information, relay it to coordinating centers in the central nervous system (CNS) where appropriate signals are generated, and then send it to the effector organs via efferent neurons.
The autonomic nervous system itself is divided into the sympathetic (thoracolumbar) and parasympathetic (craniosacral) limbs. While the sympathetic and parasympathetic limbs have opposing effects, these two limbs work together in coordination to maintain optimal functional status of the body in response to different stresses and demands at different times. The enteric nervous system (ENS) has also been included as the third limb of the ANS by some authorities [10–12].
Autonomic efferent fibers exit the CNS at different locations: parasympathetic fibers exit from cranial and sacral regions starting at the level of cranial nerves while sympathetic fibers exit from thoracolumbar region ending near the 2nd lumbar spinal cord segment. Intervening regions of the CNS give rise to somatic nerves. The efferent ANS is a two-neuron visceral motor system. The cell bodies of these presynaptic efferent neurons arise either in the brain stem or in the spinal cord gray matter (CNS) and the axons that arise from the CNS (preganglionic fibers) end at autonomic ganglia which are located at three areas: sympathetic trunk, preaortic ganglia, and near the walls of organs that are innervated. The first two are mostly sympathetic while the last one is parasympathetic, also called the intramural ganglia. Second order neurons (postsynaptic neurons) have their cell bodies in these ganglia and their axons end at the target organs.
Afferent autonomic neurons have their cell bodies in the cranial and dorsal root ganglia. Their peripheral fibers originate in the walls of the viscera and the axons travel alongside somatic afferent nerves through cranial nerves or dorsal spinal roots into the CNS. In the case of the vagal visceral afferent fibers, the cell bodies are in the superior and inferior vagal ganglia. Their central processes end in the vagal nucleus or nucleus tractus solitarius (NTS) of the medulla [13].
At each synaptic site, autonomic neurons secrete different transmitters to serve different functions. All preganglionic and parasympathetic postganglionic neurons secrete acetylcholine (Ach). Most postganglionic sympathetic neurons use norepinephrine (NE) with the exception of those supplying the adrenal medulla and sweat glands where ACh is secreted.
Certain autonomic signals are coordinated at the autonomic ganglia without reaching the central coordinating areas, in addition to organ-to-organ communication. Examples of entero-enteric reflexes include slowing of gastric emptying in response to acid in the proximal small bowel [14] and stimulation of pancreatic secretion when nutrients enter the small intestine [15]. The afferent neurons that convey the signals to the ganglia involved in these reflexes are called intestinofugal neurons; these neurons are unusual in the sense that their cell bodies are located in the gut wall and the axons terminate in the ganglia [15].
Brief Review of the Autonomic Pathways of Nausea and Vomiting
Nausea and vomiting may occur together or independent of each other and this may be due to differing pathophysiologic mechanisms. Newer antiemetic agents like the 5-HT3 and NK1 receptor antagonists while being very effective for vomiting still are unsatisfactory for the management of nausea [16–19].
The mechanism of vomiting involves a central emetic center. Rather than being a distinct anatomical area, this center is more of a functional zone or a signal generating area in the medulla and comprises the reticular formation, NTS, dorsal motor nucleus of the vagus (DMV) and ventrolateral medulla [20–22]. This center receives input from various areas of the body, including vagal afferents from the gastrointestinal tract, stimuli from the vestibular and visual areas, psychogenic stimuli from the cerebral cortex and chemoreceptor trigger zone (CTZ) [21]. The CTZ is located in the area postrema, is functionally outside of the blood brain barrier (BBB), and is activated by various endogenous and exogenous chemicals including medications. Efferent fibers from the emetic center travel via mainly the vagus nerve but also via cranial nerves V, VII, IX, and X [23]. (See Chap. 2 for review of the pathophysiology of vomiting).
Mechanism of Nausea and Vomiting of GI Tract Etiology
Chemoreceptors and mechanoreceptors in the GI tract can sense various chemical (e.g., acids, irritants, toxins) and mechanical (distension) stimuli. Detection of these various stimuli is the function of the enterochromaffin cells (ECC), which in turn release mediators that stimulate the vagus nerve [24]. Afferent vagal fibers take this signal to the emesis center. A variety of chemical mediators are involved including 5-hydroxytryptamine (serotonin, 5-HT) acting on the 5-HT3 receptor, substance P acting on the NK1 receptor, and cholecystokinin (CCK). Various other mediators are involved in enhancing or attenuating the effects of these mediators. For example in the case of 5-HT release, acetylcholine (muscarinic M3 receptors), norepinephrine (β receptors), histamine (H2 receptors), and 5-HT itself increase 5-HT release, whereas γ-aminobutyric acid (GABA) B receptors, vasoactive intestinal polypeptide (VIP), and somatostatin inhibit 5-HT release [25]. Antagonists to these various chemical mediators form the basis of antiemetic medications.
The site of action of these antiemetic medications is either central or peripheral or both. 5-HT receptors exist at the vagus nerve endings as well as in the brainstem areas including NTS, DMV, and area postrema [26]. Neurokinin (NK) receptors have also been found in the CNS vagal neurocircuits as well as in the vagal afferents [26–28].
Pathophysiology of Nausea
Historically, nausea and vomiting have been thought to be a continuum of the same pathophysiologic mechanism caused by similar stimuli and involving the same neural circuits but of varying intensity and/or duration. A temporal association exists between the stimulus and subsequent nausea via a constant level of stimulation and the intensity of nausea increases in parallel to the duration of stimulus [29]. The neural pathways involved in the pathogenesis of nausea have not been clearly defined; the hypothalamus and inferior cerebral cortical gyrus may be involved [30].
Experimental methods of studying nausea include gastric distention using a barostat [31]. Distension of the distal stomach induced nausea and gastric dysrhythmia and these effects were not blocked by granisetron (a 5-HT3 antagonist) or atropine. Distal gastric distension also induced bloating and pain and these symptoms were also not alleviated by 5-HT3 blockers.
A variety of physiological changes accompany nausea [32]. These include features of sympathetic activation (tachycardia, sweating, and vasoconstriction) as well as an increase in plasma levels of vasopressin, and gastric dysrhythmias. There is a correlation between the onset of nausea and rise in plasma vasopressin level [29]. In human studies, exogenous administration of vasopressin produced nausea and gastric tachyarrhythmia [33]. (See Chapter. 1 for review of the pathophysiology of nausea).
Role of the Vagus Nerve in Pathogenesis of Nausea and Vomiting
The vagus nerve plays a critical role in the pathogenesis of nausea and vomiting. Vagal afferents carry stimuli in the viscera to the CNS. The importance of the vagus nerve is illustrated in studies of vagotomy or vagal nerve stimulation. Wang and Borison showed that vagotomy abolished nausea and vomiting caused by intragastric administration of copper sulfate [34]. Electrical stimulation of the vagus nerve has been shown to elicit a vomiting response [35]. In ferret models, gastric or duodenal mucosal stimulation caused activity in the vagal afferents [36]. The afferent signals travel in the vagus nerve in the C type fibers.
Studies have shown increased c-fos activity in the reticular formation, nucleus tractus solitaries, dorsal motor nucleus of the vagus, and ventrolateral medulla during vomiting, highlighting the importance of these areas in the emetic response [22, 37, 38]. Area postrema ablation studies have shown that administration of emetogenic substances can cause abolishment or attenuation of vomiting [39].
Multiple autonomic reflexes accompany the vomiting response. These include excessive salivation, tachycardia, breath holding, sweating, and the motor activities of the upper GI tract including lower esophageal sphincter (LES) relaxation, retrograde peristalsis, and contraction of the abdominal muscles. The NTS plays a critical role in the coordination of these reflexes [40]. All the structures that have communication with the NTS also play important roles in regulation of the medullary reflexes involved in nausea and vomiting [22, 41]. Activation of the vagal afferents by emetogenic stimuli activates regions in the NTS that control the sensory aspects of swallowing and cardiovascular and respiratory functions [21, 22, 38]. Neurons of the NTS communicate with the adjacent DMV from where preganglionic parasympathetic neurons arise, which transmit the integrated response back to the GI tract [41]. DMV neurons are also under complex control by innervation from the NTS itself, as well as by numerous neurotransmitters (e.g., opioid peptides [42, 43], Serotonin [44], tachykinins [45, 46], and dopamine [47, 48]).
Postganglionic neurons are of two types—excitatory and inhibitory. Excitatory neurons are cholinergic and cause muscle contraction. Inhibitory neurons that cause muscle relaxation are nonadrenergic and noncholinergic and release either VIP or nitric oxide (NO). During the actual act of vomiting, vagal efferent signals cause retropulsive activity in the intestine, gastric contraction, and relaxation of the lower esophageal sphincter (LES) and pyloric sphincters [49–51].
It has been shown that receptors to 5-HT exist in the brainstem areas including NTS, DMV, and area postrema. This, along with the observation that centrally applied 5-HT3 receptor antagonists block CINV, suggests that the action of 5-HT may not be limited to peripheral sites [26, 38, 52, 53]. In addition, 5-HT released from ECC may also activate neurons in the ENS to modulate GI motility [27, 54, 55]. Neurokinin receptors have also been found in the CNS vagal neurocircuits as well as in the vagal afferents [28, 56, 57].
Role of Sympathetic Nervous System in Nausea and Vomiting
Very little is known about the role of sympathetic limb of ANS in nausea and vomiting; studies and literature related to this subject are limited. Nausea and vomiting reflex is predominantly controlled by the vagal limb of ANS, so most of the studies on nausea and vomiting are focused on the vagus nerve. Since parasympathetic and sympathetic systems have opposite but complementary functions, it can only be assumed that while parasympathetic arm is actively participating in the process, the sympathetic is exerting a complementary balancing effect. Nevertheless, studies have shown changes in sympathetic function in diseases that are associated with nausea and vomiting. Adult cyclic vomiting syndrome (CVS) is associated with an increase in sympathetic activity and vagal inhibition leading to alteration in gut motility [58, 59]. Schaub et al. have shown that nausea is associated with increased sympathetic and decreased parasympathetic tone [60]. Animal studies support the previous findings that there is increased sympathetic activity just before retching [61]. Other human studies have concluded that instead of direct effect on the gut, vestibular stimulation increases sympathetic outflow to the skin, producing cutaneous expression of motion sickness [6]. Some researchers hypothesize that sympathetic activity can be a defensive reaction against nausea and vomiting [7]. Additionally, Uchino et al. also explain similar results from their animal study claiming that the increased sympathetic activity suppresses the emetic response evoked by motion sickness.
In a study of patients with anorexia nervosa, Abell et al. found impaired sympathetic autonomic function in anorexic patients with statistically significant lower resting diastolic blood pressure (BP) and skin conductance and impaired response to the cold pressure test compared to the control group [62]. Abell et al. also found a statistically significant correlation between the sympathetic adrenergic measure of vasoconstriction to cold stress and the slope of solid gastric emptying in diabetic patients presenting with symptoms of gastroparesis [63].
Orthostatic Intolerance and POTS
Orthostatic intolerance (OI) refers to a variety of symptoms as a result of intolerance to an upright posture. These symptoms include loss of consciousness or lesser cognitive deficits like dizziness, vertigo, difficulty in concentration or memory loss, as well as headaches, fatigue, changes in blood pressure, tachycardia, and GI symptoms like nausea and abdominal pain [64]. OI includes conditions like orthostatic hypotension including neurally mediated hypotension and syncope (NMH) as well as postural orthostatic tachycardia syndrome (POTS) and is estimated to affect some 500,000 patients in the US [65]. Up to one-third of patients with POTS may develop neurally mediated syncope [66]. Neurally mediated syncope is the result of impaired cerebral perfusion due to sudden change in autonomic nervous system activity [67]. Vaso-vagal, situational (e.g., cough, swallowing, micturition) or carotid sinus syncope are examples of neurally mediated syncope. Syncope in these conditions is preceded by symptoms such as pallor, diaphoresis, nausea, abdominal discomfort, yawning, sighing, and hyperventilation.
According to the consensus statement on the definition of these disorders, POTS is defined by a sustained heart rate increase equal to or more than 30 beats/min within 10 min of standing or head-up tilt in the absence of orthostatic hypotension. The standing heart rate for all subjects is often ≥ 120 beats/min [67]. This definition does not apply to patients with a lower resting heart rate and the rise in pulse rate needs to be at least 40 beats per minute (BPM) in children.
The manifestations of POTS result from decreased cerebral perfusion and increased sympathetic activation [67]. Patients with OI and POTS can exhibit a variety of GI symptoms: in a series of adults with POTS, a considerable number of patients presented with GI symptoms of nausea (39 %), bloating (24 %), diarrhea (18 %), constipation (15 %), and abdominal pain (15 %) [2–4, 68].
In the pediatric literature, there have been case reports and case series of patients presenting with abdominal pain thought to be of functional origin; further testing revealed they had autonomic dysfunction. Treatment of the underlying OI led to resolution of the abdominal pain [9, 69, 70]. One pediatric study (age range 9–17 years) looked at 24 patients whose main GI symptoms were abdominal pain (71 %), nausea (56 %), and vomiting (50 %). When specifically asked, these patients also described other OI symptoms like lightheadedness and dizziness. Upon autonomic testing, 4/24 (17 %) were found to have POTS, 12/24 (50 %) had NMH and 8/24 (33 %) had both POTS and NMH. Follow-up was available for 18 of these patients and 14/18 (78 %) had complete symptom resolution with treatment of the OI [5].
The pathogenesis of POTS is not clearly understood and likely represents a clinical endpoint or syndrome. POTS has been classified into different subtypes including hypovolemic, neuropathic and hyperadrenergic [71, 72]. The pathophysiology of POTS can be better understood based on the different subtypes.
Neuropathic POTS
[72, 73] is characterized by peripheral sympathetic denervation in the lower limbs as demonstrated by abnormal sudomotor tests. There is also impaired norepinephrine (NE) release in the lower limb in response to upright posture [73]. In one retrospective study, more than half of the patients had evidence of peripheral sudomotor denervation [68]. The underlying pathophysiologic disturbance in these patients is believed to be impaired peripheral vasoconstriction causing pooling of venous blood [74].
Hyperadrenergic POTS
In certain cases, genetic mutations have been identified to explain the etiology of POTS. In a 2000 study, the gene responsible for POTS was identified in one family. This gene codes for a NE transporter, and deficiency in this gene product allows for excessive NE levels [77]. POTS occurs more commonly in patients with connective tissue disorders like Ehlers–Danlos syndrome (EDS) [78, 79].
Mast cell activation
Autoimmune mediated autonomic neuropathy
An autoimmune basis for POTS is suggested based on the fact that an antecedent viral infection and antibodies to ganglionic ACh receptors are seen in some POTS patients. In a large retrospective study of 152 patients, an antecedent viral infection was seen in 90 % and antibodies to ganglionic ACh receptors in 14.6 % of patients [68].
Hypovolemic POTS
A relative hypovolemia has been proposed as a possible pathophysiologic mechanism of POTS. Reduced plasma, red cell, and total blood volumes have been found in many POTS patients [3, 82, 83]. A study found reduced daily urinary sodium excretion (100 mEq/L Na/24 h) in 28.9 % of POTS patients [68]. Yet another study found low plasma renin activity in hypovolemic POTS patients; it has been suggested this may be due to renal autonomic denervation [84].
Cyclic Vomiting Syndrome (CVS)
CVS is a clinical syndrome of recurrent episodes of nausea and vomiting separated by symptom-free periods. First described in 1882 by Samuel Gee in a series of 9 pediatric patients and initially thought to be a childhood disorder, it is now known to affect patients in all age groups. There appear to be similarities and differences in pediatric versus adult onset of the disease [85, 86]. A number of other symptoms, chief among them abdominal pain, may accompany the episodes and the disorder is known to be associated with other disorders as discussed below.
The Rome III criteria for the diagnosis of CVS require certain features be fulfilled for the preceding 3 months, with symptom onset at least 6 months before diagnosis. The criteria are as follows:
- (i)
Stereotypical episodes of nausea and vomiting
- (ii)
Three or more discreet episodes in the preceding year; and
- (iii)
Absence of symptoms between attacks [87].
In both adults and children, the episodes of nausea and vomiting last from hours to days and are accompanied by abdominal pain. Episodes are triggered by physical or mental stress as well as certain foods. Migraine headaches are associated with both adult and pediatric CVS.
In children the incidence of CVS has been reported to be approximately 3 per 100,000 children per year [88] with a prevalence of 0.04–2 % [59, 89]. The disorder is diagnosed at a median age of 7.42 years (range 1.8–15) [88]. Factors triggering the episodes include physical or mental stress like infections, anxiety, or panic attacks. Anxiety-provoking situations for children may include exams or social events. Vomiting attacks are accompanied by pallor (87 %), lethargy (91 %), anorexia (74 %), nausea (72 %), and abdominal pain (80 %) [90]. In the natural history of the disease, as the patients grow, they may “grow out” of the episode, start having migraines instead of vomiting episodes, or may continue having CVS [91].
CVS in adults was reported by Abell in 1988 in a series of 8 patients. Since then the disorder has been increasingly recognized in adults; currently in the medical literature, adult studies predominate over pediatric [85]. The essential features of adult CVS are the same as pediatric CVS—episodes of nausea and vomiting interspersed with symptom-free periods, similar exacerbating factors and association with abdominal pain, which can be severe, in 58–71 % of patients [86, 92]. A cyclic vomiting-like syndrome has also been associated with chronic cannabis abuse and this is also referred to as cannabinoid hyperemesis syndrome [93]. Other variants of CVS include some diabetic patients without gastroparesis who experience episodic vomiting with symptom-free intervals as in CVS [94]. Another group of diabetic gastroparesis patients may have cyclic episodes of vomiting and meet the criteria for both conditions. Some patients may have recurrent morning nausea with limited vomiting which resolve later in the day [85]. Associated conditions seen in adults with CVS include migraine headaches, psychiatric disease, gastroesophageal reflux disease, irritable bowel syndrome, gallbladder disease, and insulin-dependent diabetes mellitus [85].
Pathogenesis of CVS
The pathogenesis and pathophysiology of CVS remain to be elucidated. The role dysregulated central neural pathways and neuroendocrine mediators involved in the afferent and efferent brain–gut pathways of nausea and vomiting may be part of the pathophysiology of CVS [85]. In one model of pathogenesis, it is thought that in individuals who are susceptible to developing the condition due to certain factors (e.g., family history of migraines, autonomic and GI dysfunction, and mitochondrial dysfunction) triggering factors (physical and mental stress), initiate the vomiting cascade. Why this leads to repeated bouts of emesis lasting hours to days is unknown [85].
Role of the Hypothalamic-Pituitary-Adrenal (HPA) Axis in CVS Pathogenesis
The stress response involves the endocrine, nervous, and immune systems to maintain homeostasis when faced with stressors. This response involves important centers located in the hypothalamus, the anterior lobe of the pituitary gland, and the adrenal gland which is commonly referred to as the hypothalamic-pituitary-adrenal (HPA) axis [95].
Corticotropin-releasing factor (CRF) is the principal regulator of the HPA axis and is involved in the synthesis and release of cortisol from adrenal cortex. The CRF also appears to have important roles outside of the HPA axis including regulation of the ANS, learning and memory, feeding, and reproduction-related behaviors [96–100]. The peptides in the CRF family include CRF and urocortin (Ucn) 1, 2, and 3 [95] and CRF receptors are of two main subtypes, namely, CRFR1 and CRFR2 [101]. CRFR2 is present in peripheral organs including heart, skeletal muscle, skin, and the gastrointestinal tract [101–103].
During periods of stress, CRF peptides, via their action on CRF receptors, inhibit gastric activity and accelerate colonic peristalsis and defecation [104]. The gastrointestinal motor response to CRF also seems to involve pathways independent of the HPA axis and mediated by modulation of the autonomic nervous system [105, 106].
CRFR1 receptors are present on intestinal mast cells [107]. There is evidence that intestinal mucosal mast cells are involved in communication between the gastrointestinal tract and the central nervous system [108]. Activation of mast cells by CRF may cause release of 5-HT, nerve growth factor, proteases, and proinflammatory cytokines. Thus, CRF may indirectly be involved in activating the emetic response by activating mast cells and indirectly stimulating vagal afferents [107–109].
Role of the Autonomic Nervous System in CVS Pathogenesis
Nausea and vomiting are accompanied by signs and symptoms of autonomic activation even when they occur in the absence of a clinical syndrome like CVS. For example, pallor, tachycardia, and sweating indicate sympathetic arousal, and salivation and relaxation of the gastric fundus are due to vagal activity. Furthermore, the retro peristaltic activity of the upper GI tract is part of the actual act of emesis.
The involvement of the ANS in CVS has been explored in multiple studies. A study of 21 patients with CVS, 40 patients with migraines, and 36 healthy controls explored the association of CVS and migraines with other disorders thought to have autonomic abnormalities as their pathogenic basis—orthostatic intolerance, reflex syncope, interstitial cystitis, Raynaud’s syndrome, chronic regional pain syndrome (CRPS), irritable bowel syndrome, functional dyspepsia, functional abdominal pain, fibromyalgia, and chronic fatigue syndrome [110]. Adult CVS was significantly associated with orthostatic intolerance and fibromyalgia as compared to controls.
Abnormalities in rate of gastric emptying have been noted in CVS patients. One study looked at the prevalence of rapid gastric emptying in 545 patients referred to a tertiary academic motility center for a variety of symptoms ranging from nausea and vomiting to abdominal pain and diarrhea. Rapid gastric emptying (less than 50 % retention at 1 h) was noted in 48 of these patients. Thirty-five percent of these patients were diagnosed with CVS [111]. Fifty-nine to seventy-seven percent of adult CVS patients were found to have rapid gastric emptying in the interepisodic period [112, 113]. However, during the symptomatic period, in those patients who could tolerate a gastric emptying test, delayed gastric emptying was noted in some patients [113]. Abnormalities in ANS function have been suggested as accounting for these observed disturbances in gastric emptying [85].
Multiple studies have directly assessed autonomic function in CVS patients. Initial studies looked at pediatric patients and found evidence of autonomic dysfunction. A study by To et al. in 14 pediatric CVS patients found enhanced sympathetic and reduced parasympathetic vagal modulation of the heart [114]. Chelimsky and Chelimsky found CVS in children to be associated with primarily sympathetic dysfunction, affecting mainly the vasomotor and sudomotor systems and they postulated a vagally modulated sympathetic effect as the best mechanistic model to account for all current physiologic data on cyclic vomiting and gastroparesis [115]. Subsequent adult studies have shown similar findings. Twenty-one adult patients with CVS underwent autonomic testing in a study by Hejazi [58]. Autonomic abnormalities, both sympathetic and parasympathetic, were noted in 9 (43 %) of these 21 patients with a predominance of sympathetic disturbances. ANS abnormalities were seen only in the adult onset CVS patients. The authors suggested that adult CVS may represent a manifestation of dysautonomia as opposed to pediatric onset CVS where other factors (migraines and mitochondrial DNA abnormalities) may be more important. Venkatesan et al. performed autonomic testing in 20 adult CVS patients and 20 controls [116]. Ninety percent of adult CVS patients had abnormalities on the tests of sympathetic function, either OI or abnormal sudomotor tests; however, parasympathetic function was preserved in all CVS patients.
Gastroparesis
Following ingestion of a meal a series of gastric neuromuscular events occur [117]. The gastric fundus undergoes receptive relaxation. At this phase there is a decrease in intragastric pressure (IGP) followed by gradual increase until the satiety point is reached [118, 119]. Receptive fundic relaxation is mediated by vagal reflexes [120]. The food is then further broken down and mixed with gastric secretions by trituration which is mediated by contractions of the gastric body and antrum. The impulse for these contractile waves is generated in the interstitial cells of Cajal (ICC) which function as gastric pacemakers. The pacemaker signals arise at the proximal gastric body along the greater curvature and propagate distally. The triturated food is then emptied into the duodenum at a controlled rate of 3 pumps per minute, which is the same rate as the gastric peristaltic contractions [117]. The pylorus acts as a gatekeeper, regulating the size and amount of gastric contents that are allowed through [121].
The sequence of these events is coordinated by interactions between gastric smooth muscle, the enteric nervous system, ICCs, and the autonomic nervous system [122]. Disorders of these structures may result in gastric motor dysfunction [123–125], which may lead to delayed gastric emptying (gastroparesis), rapid gastric emptying (dumping syndrome), or other motor dysfunctions such as impaired fundic relaxation causing functional dyspepsia.
Vagal afferents from the stomach are stimulated by mechanical and chemical stimuli and efferent vagal fibers control gastric GI motor and secretory functions [126]. Gastric accommodation is mediated by a vago-vagal reflex [120]. Vagotomy, or electrical vagal stimulation performed to inhibit vagal function in humans, had modulatory effects on food consumption, inducing early satiety and weight loss [127]. Studies using the recently developed endoscopic functional luminal imaging probe (EndoFLIP), to measure pyloric resistance have shown correlation between pyloric diameter and compliance with gastroparesis symptoms [128, 129].
Gastroparesis is a disorder of gastric function due to delayed gastric emptying in the absence of mechanical obstruction [130]. Symptoms include early satiety, nausea, vomiting, and bloating with a predominance of nausea and vomiting [131]. Upper abdominal discomfort is present in 46–89 % of patients [131, 132], likely due to visceral hypersensitivity to gastric distension [133]. While not the predominant symptom, when present it is not alleviated by treatment of the underlying motility disorder [132].
Gastroparesis has been reported to affect up to 5 million individuals in the United States [134] with a predominance of female patients. In a study of 343 patients with functional dyspepsia, delayed gastric emptying was seen in 33.5 % of patients [135]. In a population-based study in Olmstead County, the prevalence of definite gastroparesis per 100,000 population was 37.8 in women and 9.6 in men [136].
The three main etiologies of gastroparesis are diabetes, idiopathic, and postsurgical. In a study of 146 patients, the etiologies were idiopathic in 36 %, diabetes in 29 %, postsurgical in 13 %, and other causes in 22 % [131].
Diabetic Gastroparesis
Gastroparesis is one of the complications of diabetes and occurs in both type 1 diabetes (T1DM) and type 2 diabetes (T2DM), though the prevalence is less in T2DM. In Olmstead County, the proportion of diabetics developing gastroparesis over 10 years was 5 % for T1DM and 1 % for T2DM. In tertiary centers, the prevalence was much higher: 40 % for T1DM and 10–30 % for T2DM [136–138].
The rate of progression of gastroparesis seems to be slower for T2DM compared to T1DM. It is unclear whether good glycemic control can prevent or slow the progression of gastroparesis [139, 140]. The occurrence of gastroparesis in diabetics not only induces GI symptoms, but poses serious problems for diabetes management. Erratic nutrient and medication absorption lead to problems with glycemic control. The first sign of development of gastroparesis may be problems with blood glucose control even in the absence of GI symptoms [140].
Pathophysiology of Gastroparesis
Diabetes can lead to rapid gastric emptying in the early stages and later to the development of delayed gastric emptying [138, 141, 142]. Diabetes-related vagal neuropathy can lead to impaired fundic relaxation causing dyspepsia [143, 144]. A variety of changes have been noted in the vagus nerve, enteric nervous system, and ICC. Vagal nerve damage has been observed both in the enteric nervous plexus and outside of the GI tract [145, 146] along with loss of nerves in the motor vagal neurons and the sensory sympathetic ganglia [147–149], and depletion of ICCs [150, 151] as well as enteric nervous system neurons [152]. In streptozocin-induced diabetes in rats, there were alterations in the presence and/or expression of neurotransmitter enzymes like neuronal nitric oxide synthase (nNOS) in vagal visceral afferents [153].
Depending on the stage of the disease, the prevalence of diabetic cardiovascular autonomic neuropathy varies from 7.7 % for recent onset T1DM to 90 % in potential pancreas transplant recipients [154, 155]. Twenty percent of randomly selected asymptomatic diabetics had abnormal cardiovascular autonomic function [124]. Diabetic autonomic neuropathy (DAN) affects the vagus nerve, and due to the widespread vagal innervation of almost all organs, it can manifest in a variety of symptoms such as resting tachycardia, exercise intolerance, orthostatic hypotension, constipation, gastroparesis, erectile dysfunction, sudomotor dysfunction, impaired neurovascular function, brittle diabetes, and hypoglycemic autonomic failure [124].
It is important to note that DAN not only poses symptomatic problems but portends an increased risk of mortality. Among diabetic patients with asymptomatic autonomic neuropathy, 5-year mortality rates of 27 % were noted compared with an 8 % mortality rate with normal autonomic function tests [156]. Mortality rates of 53 % versus 15 % over the 5-year period were found in diabetic patients with and without abnormal autonomic function tests. Deaths were due to renal failure, and sudden death and presence of gastric symptoms in patients with abnormal autonomic function tests carried a particularly poor prognosis [157]. Thus, diabetic gastroparetics may benefit from formal autonomic testing.
Three tests, R-R variation, Valsalva maneuver, and postural blood pressure testing, have been recommended for longitudinal testing of the cardiovascular autonomic system (CAN) [158]. Reduced heart rate variability (HRV) is the earliest indicator of CAN [124] and is associated with increased risk of coronary heart disease and death [124, 159]. HRV is the change in the time intervals between adjacent heartbeats and is reflective of the functioning of the ANS [160]. HRV indices provide a noninvasive assessment of cardiovascular control mechanisms. Commercial devices are available to provide automated measurement of HRV.
Autonomic Function Testing (AFT)
Clinical tests have been developed that assess the functional integrity of different aspects of the autonomic function system. Standard tests of autonomic functions include sudomotor (sympathetic cholinergic), cardiovagal (parasympathetic), and sympathetic adrenergic system function.
Sudomotor function tests These tests assess the integrity of the sweat-inducing pathways which involve sympathetic cholinergic pathways and comprise the quantitative sudomotor axon reflex test (QSART) as well as the thermoregulatory sweat test (TST) [161].
Quantitative sudomotor axon reflex test (QSART) evaluates the functional integrity of the postganglionic sympathetic sudomotor axon and is performed by acetylcholine iontophoresis into the skin. A capsule with a hygrometer is placed over the skin on the test site and the amount of sweat under the capsule is measured at various times. Sweating response is recorded at one site in the upper extremity and three sites in the lower (forearm, proximal leg, distal leg, and foot) and the results are compared with established normal values. QSART test assesses peripheral small fiber neuropathy.
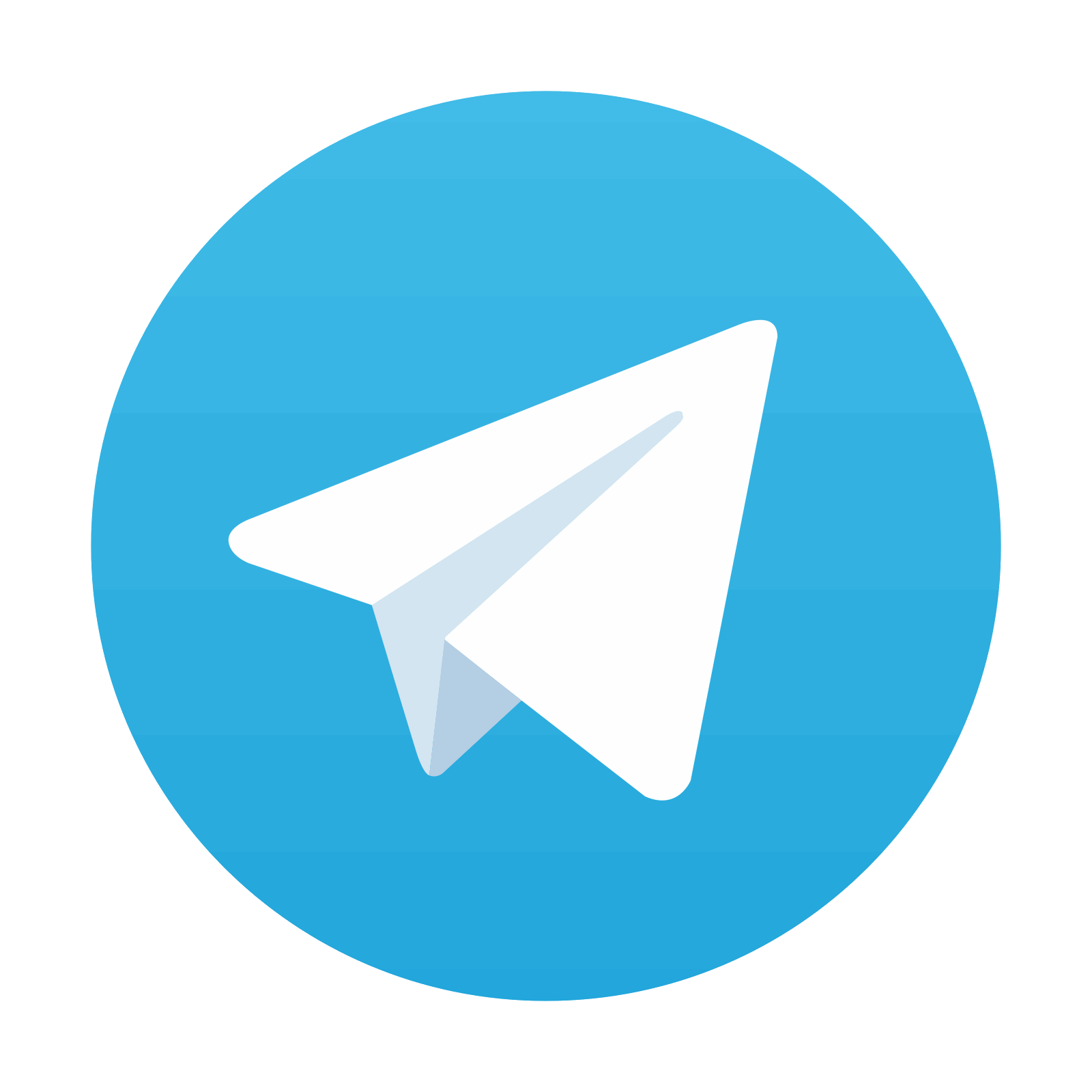
Stay updated, free articles. Join our Telegram channel

Full access? Get Clinical Tree
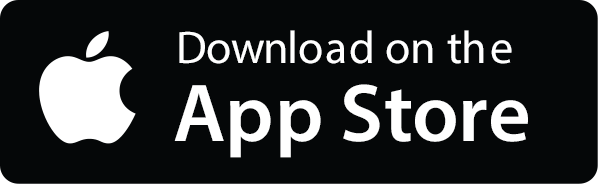
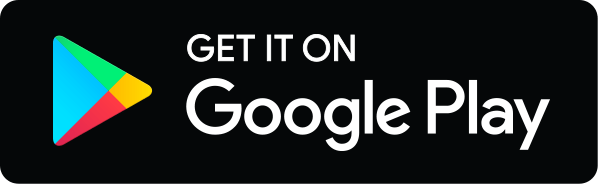