Abstract
The mammalian intestinal epithelium serves as a critical barrier between the host organism and the contents of the gut, providing for regulated uptake of food and fluid as well as protection from pathogens and toxins. This single-cell lining is constantly subjected to insult and injury and must be efficiently repaired. The initial and critical phase of repair is restitution, in which epithelial cells at the wound perimeter migrate into the injured region and reseal the barrier. Failure or dysregulation of these processes is implicated in the pathogenesis of a number of intestinal and extraintestinal disease states. A thorough understanding of the regulatory mechanisms promoting and limiting the wound healing response in the gut epithelium will open new therapeutic avenues. This chapter will discuss the basic mechanisms of cell migration involved in restitution, the laboratory methods used to investigate this process, and current knowledge of the molecular and cellular mechanisms by which the organism regulates wound healing in the small intestine and colon.
Keywords
Intestinal restitution, Colonic restitution, Wound healing, Cell migration, Signal transduction, Inflammatory bowel diseases, Colon cancer
29.1
What Is Restitution?
The epithelial lining of the mammalian small intestine and colon is a rapidly renewing population forming a single-cell barrier along the entire length of the lower gastrointestinal (GI) tract. Ulcerated wounds in the epithelium are typically repaired by coordinated molecular, cellular, and tissue processes that serve to reestablish the epithelial sheet continuity and normal cellular phenotypes. Conceptually, the repair process can be broadly segmented in vitro into early and late phases, though these are not guaranteed to be temporally distinct in vivo. The early phase is known as restitution, in which epithelial cells move into the injured or denuded regions, thereby providing an interim seal. Restitution is predominantly characterized by cellular migration and is independent of proliferation. The end result of restitution is that the wound is covered by a sheet of epithelial cells with stretched morphologies. The late phase of repair involves cellular proliferation and tissue morphogenesis, which restore the original density of cells and crypt/villus structures, respectively ( Fig. 29.1 ).

29.1.1
Restitution In Vitro
Studies of mechanically generated wounds in layers of intestinal epithelial cells grown in vitro have detailed the cellular changes that underlie restitution. At the most basic level, restitution activates migration pathways that are conserved across nearly all eukaryotic species and utilized in multicellular organisms during embryogenesis, immune responses, and tissue repair (reviewed in Ref. ). Migration behaviors are evoked from a fundamentally distinct polarization of intestinal epithelial cells, as many cytoskeletal proteins, such as villin, normally localized to the apical brush border are relocated to the migration front. Intestinal epithelial cells at the edge of the wound reorient and project lamellipodia, flattened extensions of the cell, in the anticipated direction of migration. These lamellipodia also contain microspikes, or small filopodia, which are rod-like extensions that stick into the wounded area and run perpendicular to the lamellipodial front, or leading edge. The formation of these specialized structures facilitates there the construction and maturation of new focal adhesions, concentrated areas where integrin-like cellular proteins bind to the extracellular matrix, and link to the cellular cytoskeleton. These focal adhesions provide the necessary traction force during cellular locomotion. They also serve as foundations for the newly placed actin fibers in the lamellipodium and filopodia and the remodeling of existing actin stress fibers that run the full length of the cell. During a single cycle of cellular propulsion, myosin motors displace these actin fibers, deforming the cell shape toward the leading edge. The stress fibers disconnect from the focal adhesions at the “rear,” or trailing edge, of the cell, likely due to increased tension. As the cell moves forward, the leading-edge structures and their focal adhesions must be remodeled and relocated so that the propulsive cycle can be repeated ( Fig. 29.2 ).

The sequence of events described above is generalizable to individual cells migrating in space (nonconfluency), but the epithelial cells of the intestine have special properties because they are also able to migrate as a sheet during restitution. Sheet migration has an advantage of maximizing the residual barrier function of the intestinal epithelium during restitution. Studies of sheet migration in cell culture have demonstrated specialized “leader” and “follower” cells following wounding. Leader cells are depolarized and exhibit lamellipodia and other migratory characteristics; they are the first to enter the wounded area and drag follower cells, which maintain their polarization, behind them. The resulting repair pattern resembles discrete “fingers” of cells moving into the wounded area; these fingers highlight the initial spatial distributions of these leader cells. Within these fingers, other cells can later adopt a leader phenotype and contribute to the restitutive process. This pattern of repair thus maintains polarized cell function in most cells at the wound edge by dictating the migratory phenotype to only a specialized set of cells.
The need for coordinated wound healing by intestinal epithelium gives rise to a complementary restitutive mechanism known as “purse-string” wound closure. This mechanism was first discovered in embryonic epidermal tissue, but has since been shown in intestinal epithelial cell culture. Instead of invoking classical migration pathways, cells at the wound edge generate a shared arc of actin fibers. This arc may be linked between cells at adherens junctions. The cells then undergo a coordinated contraction of the arc, akin to closing a purse by pulling on a string, that rapidly pulls the cells together into the wounded area. In purse-string restitution, epithelial cells maintain their polarity. Thus, they can participate in wound repair without undergoing an energy-intensive transdifferentiation/dedifferentiation procedure.
Cell migration and purse-string wound closure are not mutually exclusive. The size and shape of the wound may determine which mechanism is predominantly used. Purse-string closure is only observed in wounds with convex edges (e.g., round wounds). Larger wounds are healed by migration, while smaller wounds may be healed by either migration or purse-string closure. In straight-edge wounds, repair fingers created by migrating leader cells may result in locally round edges that can be healed by the purse string mechanism. However, because of relative difficulties in optimizing culture, wounding, and visualization systems to analyze molecular mechanisms of purse-string healing, most molecular studies of restitution focus on the more-reproducible cell migration phenomenon.
29.1.2
Restitution In Vivo
Recent advances in intestinal wound models and imaging techniques in mice have defined some of the changes in intestinal tissue architecture during epithelial repair. Normal colon contains a repeating array of thin crypts that are axially oriented, with progenitor cells located at the crypt base and differentiated cells at the luminal surface. After ulceration, surviving crypts at the wound edge shorten and open laterally—these lateral extensions provide a path for epithelial cells to migrate into the wound. These migratory cells are referred to as wound-associated epithelial (WAE) cells and can be identified by their expression of claudin-4. As restitution progresses, the crypt lateral openings extend and deepen; surface reconstructions of the epithelium show a network of “canals” or “channels” that represent lengthy, lateral invaginations of crypt structures. Resumption of proliferation characterizes the late phase of ulcer healing. During this phase, new crypts are seeded along the lateral extent of the wound canals, and WAE cells are replaced by homeostatic absorptive and secretory cell lineages.
Restitution in vivo is assisted by the activities of immune, mesenchymal, and microbial cells, which directly activate restitutive signaling pathways in epithelial cells. These are described in more detail later in this chapter. An important indirect mechanism assisting restitution is the contraction of tissue structures after injury, which reduces the effective wound area. Colons with severe experimental colitis are shorter. Villus structures in the small intestine are also contracted after injury. The contraction phenomenon is tetrodotoxin-sensitive, suggesting that it is partially mediated by neuronal activity.
29.2
Intracellular Signals Involved in Restitution
Due to the highly interconnected nature of signaling networks inside of cells, hundreds to thousands of proteins and smaller biomolecules impact restitution. For simplicity, however, a discussion of the intracellular mechanisms of restitution can be centered on two structures: actomyosin (actin- and myosin-containing) filaments and focal adhesions. Biomolecules involved in the regulation of formation and breakdown of these structures have demonstrated effects on cell migration, the central cellular process underlying epithelial restitution.
Formation of lamellipodial and filopodial structures requires higher-order assembly of actin filaments (reviewed in Refs. ). These filaments are formed against the edge of the cell, leading to deformation and protrusion of the plasma membrane. In lamellipodia, actin filaments are arranged in a meshwork, with filaments oriented ~ 70 degrees to one another. In filopodia, actin filaments are bundled straight together by fascin proteins. Several scaffolding and enzymatic proteins are critical to the assembly actin filaments. The ARP2/3 complex is responsible for nucleating F- (filamentous-) actin branches from the surface of existing filaments. ARP2/3 complexes are bound to WASP/WAVE-family proteins, which are nucleation-promoting factors that dissociate from ARP2/3 after nucleation. The formation of branched actin networks is especially important for lamellipodia. A second class of F-actin-nucleating machinery centers on the formin (also known as diaphanous) complex. Unlike ARP2/3, formin proteins can nucleate unbranched actin. Formin proteins follow the growing (or “barbed”) end of the filament, protecting it from premature capping. During the filament elongation process, formin proteins attract unpolymerized, or G- (globular-) actin, by binding to profilin proteins carrying the G-actin. To enhance directional propagation of actin filaments, cofilin protein frees actin monomers from the filamenťs “pointed” end, thereby enabling recycling of these monomers to the growing end.
The F-actin assembly process is regulated by the activities of small GTPases (reviewed in Ref. ). When bound to GTP, these GTPases interact with downstream effectors of actin polymerization. When bound to GDP, the GTPases are inactive. Guanine nucleotide-exchange factors (GEFs) replace GDP with GTP in the binding pocket of the GTPase; thus, GEFs activate GTPase-mediated downstream signaling. In contrast, GTPase-activating proteins (GAPs) increase the GTP-hydrolyzing activity of the GTPase; thus, GAPs inactivate GTPase-driven signaling. A second class of inhibitor of GTPase signaling consists of the guanine nucleotide-dissociation inhibitors (GDIs), which bind to GTPases and sequester them to the cytosol, thereby interfering with the GTPase-effector interactions normally occurring at the plasma membrane.
Specific GTPases have targeted functions in the protruding structures associated with cell migration. In filopodia, CDC42·GTP activates WASP and formin proteins to nucleate F-actin filaments. In lamellipodia, the RAC1GTPase achieves a similar function (creation of an actin meshwork) through activation of WAVE and formin proteins. The Rho family proteins including RhoA, RhoB, and RhoC are responsible for nucleating actin stress fibers throughout the cell. Thus, the coordinated activities of CDC42, RAC1, and Rho GTPases mediate cellular-wide remodeling of the actin cytoskeleton to promote restitution.
In order to generate the force for locomotion, intestinal epithelial cells primarily utilize myosin II motors attached to the actin filaments (reviewed in Ref. ). Immunohistochemical stains have demonstrated that myosin II is enriched at the lamellipodium and the leading edge. Myosin II is composed to three pairs of subunits: two heavy chains, two regulatory light chains, and two essential light chains that support the heavy chains. Phosphorylation of the regulatory light chains increases the ATPase activity of myosin, thereby facilitating the intramolecular power stroke that displaces the actin filament. There are more than a dozen kinases known to phosphorylate nonmuscle myosin II, including myosin light-chain kinase (MLCK) and Rho-associated, coiled coil-containing kinase (ROCK). In turn, MLCK and ROCK are regulated by upstream cellular activities including Ca 2 + -calmodulin signaling (in the case of MLCK) and RhoA GTPase activity (in the case of ROCK). In addition to phosphorylating myosin directly, ROCK negatively regulates the MLC phosphatase, thereby further favoring the activation of myosin.
The cytoskeletal attachment to the underlying extracellular matrix through focal adhesions enables enough force to be generated to bend the cell during migration. A central protein that contributes to the structure of focal adhesions and regulates their assembly, disassembly, and actin attachment is focal adhesion kinase (FAK) (reviewed in Ref. ). FAK also represents a signaling hub through which information about cell state can be traded with the cell motility machinery. FAK-knockout mice have impaired developmental morphogenesis that has been ascribed to impaired migration, but paradoxically exhibit an excess number of focal adhesions. FAK therefore seems to be important for the remodeling of focal adhesions, which is particularly important for restitution because the focal contacts must be disassembled and reassembled as the cell moves. At focal adhesions, integrins binding to specific matrix ligands are linked to intracellular actomyosin filaments through talin and paxillin proteins. FAK binds to both talin and paxillin. FAK can be phosphorylated at multiple sites by multiple kinases, but it can importantly also be autophosphorylated (e.g., at Tyr397). Phosphorylated FAK can potently interact with SH2-domain containing proteins including Src-family kinases (e.g., Src, Yes, Fyn), PLCγ, p120Ras GAP, PI3K, and SOCS. FAK attains peak catalytic activity when bound to and phosphorylated by Src-family kinases. Active FAK-Src can then phosphorylate p130Cas, which influences RAC1 activation and nucleation of F-actin, and paxillin, which may stimulate remodeling of the focal adhesion. In addition, by activating GEFs (such as p190), ROCK, Cdc42, and WASP proteins, FAK promotes cytoskeletal fluidity and actinomyosin contraction. When activated, FAK can bind GRB2 and coordinate downstream ERK- (MAPK-) signaling, thereby enhancing the effect of growth factor-related cues. Certain phospho-signals on FAK also mark it for detachment from the focal adhesion, ubiquitination, and subsequent degradation. Thus, FAK regulates focal adhesions, actin assembly, and migration through diverse molecular mechanisms.
29.3
Intracellular Modulation of Restitution Through Growth Factor Signaling and Polyamines
There are numerous potential points for modulation of the cellular signaling network in restitution. Growth factor signaling and polyamine signaling “plug” into many of these pathways and potently enhance restitutive behaviors in intestinal epithelial cells. Among the best-described mediators of epithelial cell migration in the growth factor class are the epidermal growth factor (EGF) receptor (R) family ligands EGF and TGF-α, and the antiproliferative peptide TGF-β. Both epidermal growth factor receptor (EGFR) and TGF-β pathways are targeted by microbial and immune signals to promote restitution (described below in Section 29.6 ). Both EGFR and TGF-β can, like FAK, be thought of as signaling “hubs” in restitution. Other growth factors or signaling peptides that promote restitution in the intestinal epithelium include HGF, which enhances both motility and proliferation through its cognate receptor c-Met, various fibroblast growth factor (FGF) family members, VEGF, and GLP-2. Studying the mechanistic basis for growth factor- and polyamine-mediated effects on restitution has helped to define critical intracellular signals that may serve as therapeutic targets to enhance wound healing.
29.3.1
Epidermal Growth Factor Receptor (EGFR)
EGF receptor family ligands, including EGF, TGF-α, betacellulin, amphiregulin, neuregulins, and heparin binding EGF-like growth factor (HB-EGF), represent a major growth factor family with profound impact on intestinal epithelial cell restitution and proliferation. These ligands bind to, stimulate dimerization of, and activate members of the ErbB receptor tyrosine kinase family, of which EGFR is the canonical and best-described member. EGFR is activated in epithelial cells immediately after injury. After EGFR ligand binding and autophosphorylation, numerous signaling molecules are rapidly activated. These include adaptor proteins (e.g., Grb2), Src-family kinases, FAK, the PLCγ/PKC cascade, PI 3-kinase, the p38 MAPK cascade, ERK MAP kinases, Akt, NF-κB, and Rac. All of these effectors except ERK MAPK seem to be required for EGF-stimulated migration. The interdependent relationships between these signal messengers have not been fully resolved. Many of them are activated in parallel, but there also might be hierarchical relationships between them. For example, p38 activation is downstream of Src activation but not PI3K or PLC pathways.
An interesting question is how EGFR activity promotes restitution, a proliferation-independent process, even though the EGFR signaling pathway is also strongly associated with proliferation and cell survival. A provocative idea is that although EGF activates a wide variety of signals, each downstream signal (or group of signals) may be linked to a distinct cellular function. Preferential activation of a specific set of signals can dictate the dominant cellular function at a given time. This has been supported in some cell lines but not others. For example, inhibition of p38 kinase after EGF stimulation of leads to an increase in ERK activation, which then increases the proliferative program in the cell, while decreasing the migration rate. We have also observed that EGFR activation regulates a select set of microRNAs, and that different microRNAs control intestinal epithelial migration versus proliferation (unpublished data, C.Y. Liu). Costimulatory growth factors, cytokines, or fatty acids present in the wound bed may bias the downstream signaling cascades toward generating the restitutive phenotype.
29.3.2
Transforming Growth Factor Beta (TGF-β)
A variety of growth factors and cytokines potentiate restitution and many of these do so through convergence on the TGF-β pathway. EGFR activation can cross talk with TGF-β signaling in the context of cell migration. The activation of TGF-β signaling in the wound repair context usually involves upregulated transcription, translation, secretion, and cleavage of TGF-β from intestinal epithelial cells. TGF-β can then activate in an autocrine manner the TGF-βRII and TGF-βRI receptors sequentially. In the canonical pathway, activated receptors signal to Smad proteins to induce transcription of target genes involved in wound healing. When applied to intestinal epithelium, TGF-β strongly increases migration rate but has no effect on cell proliferation, consistent with cellular behaviors in restitution. The exact mechanisms by which TGF-β enhances restitution remain to be elucidated. TGF-β can activate specific MAPK signals. In addition, TGF-β activity can modify the types of integrins expressed at focal adhesions; specific sets of integrins expressed at the cell surface may be optimally adapted for wound healing.
29.3.3
Polyamines
Polyamines, including the abundant spermine, spermidine, and putrescine, have essential roles in restitution. They are small molecules harboring multiple amine groups that are produced from the rate-limiting ornithine decarboxylase (ODC) enzyme. Polyamine levels are tightly regulated in the cell (reviewed in Ref. ). Depletion of polyamine synthesis through inhibition of ODC leads to broad defects in cell migration. These defects are manifested at the molecular level through reduced Cdc42, Rac1, and RhoA expression, decreased integrin clustering, and reduced phosphorylation of FAK, leading to defective protrusion structures and focal adhesions. Compensation experiments with intermediate signaling moieties have defined critical mediators of polyamine-driven restitution. Expression of constitutively active Rac1 or MEK, an upstream activator of MAPK, restored cell migration in the context of ODC blockade. The effect of polyamines is partially independent of EGFR.
Polyamine depletion has highlighted the importance of ionic signaling in the maintenance of restitutive responses. Inhibition of ODC results in the reduced expression and altered activity of voltage-gated potassium channels (Kv1.1) in intestinal epithelial cells. The resting membrane potential of the cell therefore increases, which in turn lessens the driving force for calcium ion entry into the cytosol. Cytosolic calcium concentration is a key determinant of cell migration. Increasing the cytosolic calcium concentration increases the expression of the RhoA GTPase, phosphorylates and frees β-catenin from adherens junctions (thereby increasing cell mobility), activates ROCK and myosin II, and increases migration; removal of calcium sources has the opposite effect. TRPC1 has an essential role in refilling intracellular calcium stores in intestinal epithelium; removal of TRPC1 abrogates cell migration. Thus, polyamines regulate cell migration by electrochemically tuning intracellular calcium levels.
29.4
Restitution Is a Distinct Cellular Function
Cellular migration is the primary behavior that defines the restitutive response. Cell migration is featured in other contexts in the GI tract such as chemotaxis and normal epithelial turnover. While so-called “restitutive” migration may share some features with these other types of migration, there are also important features that distinguish restitution. Chemotaxis is directed cell motility toward a chemical gradient. In a wound bed, there are undoubtedly chemotactic agents such as cytokines, growth factors, nucleotides, and phospholipids secreted by immune, mesenchymal, microbial, and dying epithelial cells. Growth factors, such as EGFR ligands, hepatocyte growth factor (HGF), lysophosphatidic acid (LPA), and trefoil factor peptides (TFFs), that are effective in chemotactic assays are also able to promote restitution. Part of the overall restitutive response may be thus mediated by chemotactic signaling. However, chemotaxis and restitution have important differences. The first difference is ontological: chemotaxis is measured as movements of individual cells in subconfluent cultures arranged in Boyden chambers, but restitution is a collective migration. Second, restitution occurs in vitro even when there is no obvious chemical gradient, and the ability of EGF and HGF to stimulate migration even in the absence of a gradient suggests that there are active, nonchemotactic components to restitution. Third, restitution requires a distinct set of intracellular signaling pathways. For example, EGF-stimulated restitution of mouse small intestinal epithelial cells requires protein kinase C (PKC) activity, while chemotaxis to EGF in this cell model does not. Also, phosphorylation of a different set of tyrosine residues on the EGFR protein is required for restitution versus chemotaxis.
Intestinal epithelial cells undergo a constant pattern of homeostatic displacement, but this is easily distinguished from the migration processes of restitution. In healthy intestine, new intestinal epithelial cells are birthed from multipotent stem cells located near the base of the crypt. As cells continue in their lifespan, they move up the crypt and acquire increasing lineage specificity. Differentiated cells at the end of their lifespan are shed into the lumen at the top of the crypt (in the colon) or villus (in the small intestine). The movement of cells up the crypt occurs while they maintain their apical-basal polarization and microvillar structures. In restitution, however, epithelial cells undergo remodeling of their cytoskeleton and adopt a flattened (as opposed to columnar) morphology. Movement of intestinal epithelial cytoskeletal markers, such as villin, from the apical microvilli to the leading edge is critical for restitutive processes. There are also significant transcriptional differences between restitutive epithelia and the stem- and absorptive cells constituting homeostatic epithelia. These observations suggest that restitution is not simply an extension of normal homeostatic mechanisms with added migratory capacity; instead, restitution is a process carried out by epithelial cells that have been reprogrammed to a distinct molecular and functional state. It is important also to distinguish restitution from rapid resealing of the epithelial barrier after normal cell shedding, which occurs as a choreographed remodeling of junctional proteins in neighboring cells in concert with the extrusion of the apoptotic cell.
What are the characteristics of the restitutive cellular state in relation to the hierarchy of differentiated cells in healthy intestine? There is general agreement that restitutive cells are not proliferative, and the process of restitution as a whole does not require proliferation. However, after the wound is resealed, it is possible that the recently migrated cells adopt a proliferative progenitor cell phenotype and initiate crypt morphogenesis and regeneration in situ. Thus, dedifferentiation and proliferation could be eventual outcomes that are associated with restitution. Alternatively, restitutive cells at the resealed surface could revert to homeostatic absorptive or secretory differentiated cell identities. Finally, restitution may be a terminal fate commitment, and the involved cells are gradually replaced by normal epithelia generated by enhanced proliferation at neighboring crypts. There is not currently a clear answer to this question. Some of the aforementioned mechanisms are employed for wound healing in other organs, such as skin. In the colon, recent work using organoid models and biopsy injury supports the idea that entry into the restitutive state is irreversible when triggered by prostaglandin signaling initiated at the wound bed, supporting an “adaptive cellular reprogramming” model in which restitution is a terminal fate specification downstream of even homeostatic differentiation states. Mice deficient in the EP4 prostaglandin receptor showed delayed wound healing. Future work will need to resolve whether restitutive cells stimulated by different cues exhibit multilineage plasticity, a strong possibility due to the overall epigenetic liquidity of intestinal crypt cells.
29.5
Modeling Restitution With Intestinal Wounding Models
29.5.1
In Vitro Models
Cultured cell monolayers : A classic wound healing model used to test responses in a variety of adherent cell types (including epithelium and fibroblasts) is the scratch assay (a sample protocol is presented in Ref. ). In this model, a pipette tip or a drill press is used to make a linear or circular scratch wound in a confluent monolayer of intestinal epithelial cells (e.g., IEC-6, YAMC, T84, Caco2, and others) grown in a tissue culture dish. Depending on the mechanical force applied during the wounding, matrix molecules coating the plate’s bottom may be preserved in the denuded region. The migration of wound-bordering cells is monitored by light microscopy at various time points (usually hours) after the wound formation, and the rate of restitution can be estimated from the wound size remaining at these different times ( Fig. 29.3 ). A variation of the scratch assay is the fence assay, wherein cells are grown to confluency around a barrier in the dish; to induce wound formation, this barrier is then removed, exposing a cell-free region (e.g., in Ref. ). To induce a population-wide restitution phenotype that can be measured with bulk assays, a repeating pattern of scratches is made on the culture dish. Major insights into the fundamental cellular and molecular processes of restitution have come from the use of pharmacological inhibitors, growth factors, and genetically or transcriptionally modified cells in the scratch wound assay. In addition, the coculture of epithelial cells with immune or stromal cells separated by a filter or cell-culture insert has enabled the evaluation of soluble factors secreted by these nonepithelial cell types in the modulation of epithelial restitution.

Enteroids/organoids : The culture of “mini-guts,” also known as enteroids (containing only epithelial cells) or organoids (containing epithelial and nonepithelial cells), has revolutionized in vitro studies of GI epithelia (beginning with the work of Sato et al. ). These cultures preserve the hierarchy of stem and differentiated cells of the source epithelium and permit basic studies of epithelial morphogenesis in a three-dimensional (3D) context. The ability to establish these cultures from biopsies and resected samples of healthy and diseased human intestine speaks to their power to link cellular changes to human disease. Moreover, techniques to genetically modify the cells within these cultures have enabled the reconstruction of initiating events in colon cancer and will likely be extended to exploration of genetic determinants of phenotypic changes including epithelial repair and regeneration.
Despite the excitement generated by this culture technology, there are few established models of wound healing or restitution. Because enteroids and organoids are usually grown as distinct 3D structures within a soft gel (such as Matrigel), it is not as simple to induce a mechanical wound like a scratch. It is conceivable that one could repeatedly pull off cells using a micropipette, but doing so manually could pose challenges to experimental reproducibility. Several studies have used laser irradiation to induce epithelial cell death within a defined spatial window; however, this technique has not been applied to study wounds that are > 1–5 cells wide. Future approaches may achieve wounding with chemotherapeutic agents to inhibit stem cell proliferation, by shifting the mechanical properties of the extracellular matrix, or some combination of the above approaches. Current efforts are also targeted at plating enteroids at a mature stage as monolayers on top of a thin gel —it would be seemingly straightforward to induce a scratch-type wound in this plating configuration—however, the properties of epithelial cells grown this way have not been extensively characterized.
One may also conjecture that the enteroid/organoid culture system by its very nature models wound healing. During the crypt or single-cell isolation process used to initiate or passage these cultures, an intact epithelium is disrupted through chelation of divalent cations and protease treatment. The resulting epithelium must then be regenerated from these disconnected parts. The signal transduction pathways and biological processes needed to reform the organoids might mimic those used in restitution; for example, EGF is an essential cofactor that is added to establish these cultures and is also critical for restitution in vitro and in vivo. The extracellular matrix (often Matrigel) in which organoids are embedded is usually derived from tumor matrix and contains a wide variety of growth factors and cytokines. High-throughput profiling studies of organoid cultures show that they may also resemble the intestine during development. As an increasing number of efforts to characterize these culture systems are made, there will be new insights into what processes in vivo they model.
29.5.2
In Vivo Models
In vivo models of intestinal restitution typically involve the creation of ulcers over which the migration of surviving adjacent epithelium can be assessed. These ulcers can be produced in a variety of injury modalities, including mechanical wounding, chemical injury, genetic models, chemotherapy and radiation, and infection, that resemble human GI insults. Each injury model has its own nuances regarding the severity of injury and the types of epithelial responses that are triggered. Mild injury may lead to a transient loss of epithelial cells within individual crypts that are replenished with a proliferative burst of stem cells without necessitating the activation of cellular migration pathways. In some models, ulcerations are only seen with severe injury, but the tissue microenvironment associated with these injuries can vary regarding the general types of immune cells, hormones, microbial agents, and matrix factors involved in the response. When evaluating whether a specific agent or gene has effects on restitution, it is important to clarify what animal models were used. Moreover, when interpreting the animal’s overall outcome after exposure to these injury models, one must note that many other biological responses (e.g., epithelial survival and morphogenesis, immune cell signaling) are at play in addition to restitution, and without detailed examination of the specimen it can be virtually impossible to ascribe differential outcomes to restitution. Nonetheless, there are a few in vivo models where the epithelial restitutive response has an important role in dictating the overall outcome.
Mechanical wounding : Direct mechanical wounding of the rodent distal colon can be achieved by manual biopsy of inner colonic surface. The animal (usually a mouse) is sedated and a mini-colonoscope is passed into the rectum, and wounds are generated using biopsy forceps attached to the end of the colonoscope. Superficial wounds of approximately 1 mm 2 surface area each can be generated in the colon. Depending on the skill of the investigator, multiple wounds can be created in a single animal, allowing for observation of restitution at different sites along the proximal-distal axis. Wounds of this size in the mouse are typically healed within 7 days. Because the colonic ulcers in this model are all created at the same time, this model has been useful to identify the time-locked onset and duration of the restitutive response, the cellular markers defining the restitutive cellular front, and influence of immune and support cells on the wound healing response. However, this model requires specialized equipment and specially trained staff. A variation of the mechanical wounding model involves the insertion of a rotating brush into the rectum, obviating the need for an expensive miniature colonoscope, but this procedure poses a significant risk for colonic perforation. A sophisticated variation of the colonoscope method modifies protocols for confocal endomicroscopic imaging and utilizes a 2-photon laser to induce photodamage to the colonic epithelium; restitution and repair of the mucosal surface occurs within a week and can be monitored over time.
Chemical models : Chemical models of intestinal wounding are common because of their relative ease-of-use. The administration of ~ 40 kDa dextran sulfate sodium (DSS) in the drinking water of rodents over 3–7 days induces epithelial injury, ulceration, and inflammation of the distal colon. DSS-induced colitis is mediated by innate immune cells, as the lymphocyte lineage has been shown to be dispensable for injury. The animal suffers reversible weight loss, bloody stools, and diarrhea. Imaging studies have shown distinct phases of restitution and crypt regeneration in the DSS colitis model. While the exact pathogenic mechanisms underlying DSS-induced injury are not known, a primary cause may be increased epithelial apoptosis, stem cell ablation, and loss of epithelial integrity as early events, which pave the way for subsequent mucosal immune responses. DSS administration spares the small intestine and upper GI tract. Thus, the DSS model enables the observation of a strong epithelial regenerative response in the context of colonic inflammation in a relatively practical model. Other chemical colitis models useful for studying restitution in vivo include intrarectal administration of acetic acid, which induces mucosal changes resembling human ulcerative colitis, and of oxazolone and 2,4,6-trinitrobenzenesulfonic acid (TNBS), which are haptenating agents that induce an adaptive immune-mediated colitis similar to human inflammatory bowel disease.
Nonsteroidal antiinflammatory drugs : Administration of nonsteroidal antiinflammatory drugs (NSAIDs) to rodents is another method to induce intestinal injury. These models demonstrate the importance of prostaglandins for maintenance of epithelial integrity and mobilization of wound healing processes. Subcutaneous injections of indomethacin induce a Crohn’s-like disease in mice and rats specifically affecting the jejunum and ileum. Depending on the injection schedule, an acute or chronic disease can be modeled. Like DSS, the mechanism of action of indomethacin has not been elucidated, but it may involve synergistic effects with specific bile acids. Another NSAID, piroxicam, can be used to accelerate the onset of colitis in animals with susceptible genetic backgrounds. These models are useful for studying epithelial responses to NSAID-induced injury and may identify restitutive targets in iatrogenic disease throughout the GI tract.
Genetic models : There is a plethora of gene knockouts that induce spontaneous colitis in mice (e.g., Refs. ). These genes affect a variety of host processes, including immune tolerance of the intestinal microbiome, epithelial barrier function, T cell signaling, the unfolded protein response, and so forth. One commonly used genetic model of colitis is the knockout of the cytokine interleukin (IL)-10 (encoded by the Il10 gene). In humans, defects in the IL-10 pathway are associated with infant-onset IBD with extensive distal colonic and anal involvement. IL-10 is involved in the suppression of immune responses to the colonic microbiome; IL10 −/− mice raised in a germ-free environment do not develop colitis. In conventionally housed animals, the colitis usually begins after weaning and intensifies with age. However, there is considerable variability in colitis onset and severity between and within animal care facilities, likely reflecting the effects of differing microbial ecosystems. Coinhibition of signaling proteins, such as EGFR, involved in epithelial restitution along with IL-10 accelerates the onset and increases the intensity of disease. Thus, genetic colitis models combined with transgenic targeting to intestinal epithelium may identify pathways involved in restitution. One should note that the outcomes of colitis in these models may be influenced by factors other than restitution; direct examination of ulcer lesions combined with staining for specific markers of WAE or cytoskeletal proteins may be required to interpret results in relation to restitution.
Chemotherapy and radiotherapy : Mucositis in the GI tract is a major adverse event in patients receiving radio- and chemotherapy. Chemotherapeutic agents and radiation are also used to induce injury in the rodent intestine; restitution pathways can be studied in these models with the ultimate goal of reducing the burden of mucositis in cancer treatments. Administration of high-dose methotrexate, doxorubicin, or ionizing radiation induces crypt cell apoptosis, ulceration, and regenerative responses in the small intestine and colon. Radioablative models have been used to determine the identities of reserve stem cell populations in the mouse small intestine. Interestingly, differing results are obtained depending on the dose and schedule of radiation given. In addition, application of both chemotherapy and radiation will alter the number and function of immune cells, especially neutrophils; thus, these may not represent optimal models for understanding the crosstalk between epithelium and immune cells in restitution.
Infection models : Mouse infection models enable the study of intestinal epithelial restitution in a context mimicking human infectious colitis. In particular, Citrobacter rodentium infections in mice emulate enteropathogenic Escherichia coli and enterohemorrhagic E. coli infections in humans. Gavage of overnight cultures of C. rodentium induces acute colitis that can range from mild to severe depending on the inbred strain of mice. While mild disease induces epithelial hyperplasia, immune cell infiltration, and goblet cell depletion, severe disease causes crypt loss and marked erosion of the epithelial surface, thereby providing a context for restitution.
29.6
Regulation of Restitution
Numerous bioactive peptides and small molecules influence restitution ( Fig. 29.4 ). These prorestitutive agents have various and overlapping sources in the wound bed and invoke both autocrine and paracrine-signaling processes. It is helpful to conceptualize the regulation of restitution by discussion of the major cellular sources of these signals, keeping in mind that a given signal can be derived from multiple cell types (e.g., prostaglandins, potent regulators of restitution, can be made by epithelial cells, immune cells, and stromal cells).

29.6.1
Luminal Factors
A variety of luminal components, derived from myriad sources, including microbial and host production and secretion, and from the diet, modulate restitution. While most homeostatic growth factor signaling is initiated by receptors located on the basolateral aspect of the intestinal epithelium, luminal growth factors may gain access to their cognate receptors after the epithelial barrier is injured. The altered polarization of epithelial cells in restitution may also affect where certain receptors are localized (e.g., at the membrane ruffles of the leading edge), thereby giving luminal factors new access to the cell signaling machinery. Other luminal factors may rely on nonreceptor mechanisms to regulate restitution.
Trefoil factors are small (7–12 kDa), protease-resistant proteins that have an essential role in intestinal epithelial restitution. There are three isoforms, TFF1, TFF2 (spasmolytic peptide), and TFF3 (intestinal trefoil factor), which can function to promote cell migration as monomers or dimers. While TFF1 and TFF2 are expressed in the upper GI tract, TFF3 is present at high levels in the small intestine and colon. TFF3 is made in the theca of goblet cells and is secreted into the lumen, where it associates with the mucus. Trefoil factor proteins are found in uninjured intestine, but their expression and secretion increase after injury. Their expression is especially notable in the so-called “ulcer-associated cell lineage” that arises adjacent to crypts after ileal ulceration in Crohn’s disease. Importantly, TFF3-null mice are unable to repair minor colonic ulcers generated using the DSS colitis model or chemotherapeutic/radiotherapeutic model ; most of these mice die after intestinal injury. In vitro, exogenous TFFs can increase restitution rates 3–6-fold, with no stimulatory effect on proliferation.
Despite their importance to restitution, the molecular mechanisms underlying TFF function remain unknown. A TFF receptor has not yet been identified, and it is debatable whether one should exist (discussed in Refs. ). Treatment of cells with TFF3 activates EGFR and induces β-catenin phosphorylation, which may link TFF function directly to the actin cytoskeleton. EGFR is required for many of TFF3’s downstream effects, but direct binding of TFF3 to EGFR has not been demonstrated. The activity of TFF does not depend on TGF-β. TFF3 treatment also leads to increased phosphorylation of FAK.
In addition to trefoil factors, the intestinal lumen also contains growth factors such as EGF and TGFα, which are potent activators of restitution. The ulcer-associated cell lineage is notable for its high expression and secretion of EGF. EGF and TGFα are produced by epithelial cells and subepithelial myofibroblasts within the stem cell niche. EGFR ligands are also produced by salivary glands, duodenal Brunner’s glands, and pancreatic secretions. The expression of EGFR ligands is upregulated during injury, thereby helping to initiate wound healing.
Short chain fatty acids (SCFAs), including butyrate, propionate, and acetate, are derived from bacterial fermentation of dietary carbohydrates and can be present in high, but varying, amounts along the luminal-to-basilar crypt axis. SCFAs can have profound impacts on epithelial cell physiology. For example, butyrate modulates colonic epithelial cell proliferation. Butyrate, proprionate, and acetate all enhance intestinal epithelial migration. Butyrate-induced cell migration depended on RNA and protein synthesis, suggesting that it requires butyrate’s metabolic-biosynthetic effects, and not the activation of a specific receptor. SCFAs also stimulated the epithelial production of growth factors and cytokines, such as IL-8, that, in turn, could promote restitution. Recent work has highlighted an additional mechanism through which SCFAs activate free-fatty acid receptor subforms 2 and 3 (i.e., FFAR2 and FFAR3) to stimulate the release of GLP-1 and GLP-2, specifically from epithelial L-type enteroendocrine cells located in the distal intestinal tract. These GLPs in turn promote the production of growth factors from subepithelial myofibroblasts (described in Section 29.6.5 ) to enhance epithelial restitution.
Another chemical mediator in the lumen, bile acids play important roles in digestion, where they bind to lipids in the intestinal lumen and facilitate their absorption. An emerging literature suggests that they also have important roles in regulating migration and proliferation of intestinal epithelial cells, though different bile salts can have striking differently effects. Exposure of epithelial cells to taurodeoxycholic acid augmented migration and inhibited proliferation. These responses were driven by NF-κB induction of TGF-β expression. In contrast, taurine-conjugated acid increases proliferation, while deoxycholic acid reduces proliferation. These effects were mediated through EGFR and FXR proteins. Thus, supplementation with specific bile salts may represent attractive strategies to promote wound healing.
29.6.2
Danger Signals and Signaling
Though epithelial wound healing is a complex phenomenon in vivo and is facilitated or inhibited by various tissues and ecosystems, the epithelium has the innate ability to recognize the presence of a wound and secrete or release danger signals. Signals released by dying cells, or surviving cells at the wound edge, can directly stimulate epithelial migration. For example, it is known that in vitro, a break in the continuity of the epithelial sheet is sufficient for EGFR activation. This is mediated by increased epithelial production of EGFR ligands. Matrix metalloproteinases (MMPs), which cleave immature forms of ligands from the cell membrane, sense the loss of normal cellular junctions and respond by upregulating their activity. Epithelial cells also serve as integrators of the tissue factors that promote restitution. Cytokines produced by epithelia and immune cells induce epithelial expression and release of TGF-β, which can then activate restitutive responses through autocrine signaling.
In addition to protein secretion, epithelial cells may encode restitutive ligands on secreted extracellular vesicles, including exosomes (40–60 nm diameter) and microparticles (100–1000 nm diameter). Secreted vesicles can potentially travel long distances to promote cell migration at the wound edge. Recent work has demonstrated that annexin A1, a resolving factor in inflammation, is upregulated on the surface membrane secreted extracellular vesicles after epithelial damage by intestinal epithelial cells. The extracellular N-terminus of annexin A1 activates formyl peptide receptor 1 (FPR1) in the target cell, leading to phosphorylation of FAK and enhanced cellular restitution. Annexin A1 can also be cleaved from extracellular vesicles to a soluble FPR1-binding active peptide. Thus, in restitution direct signaling of soluble factors and extracellular vesicles are not exclusive.
Dying cells in a wound may release damage-associated molecular patterns (DAMPs), which include free nucleotides (e.g., adenine, uracil), amino acids (e.g., glutamine, arginine), DNA-binding proteins (e.g., HMGB1), phospholipids (e.g., LPA), prostaglandins, and molecular chaperones (e.g., heat shock proteins). DAMPs classically signal to innate immune cells to trigger an inflammatory response at the site of the wound. Their activity is mediated by pattern-recognition receptors. DAMPs have important roles in the regulation of restitution. ATP, ADP, UDP, LPA, glutamine, prostaglandins, and arginine individually accelerate intestinal epithelial migration. ATP and ADP, in particular, also inhibit enterocyte proliferation, and their effects are independent of TGF-β pathways. Extracellular HMGB1 has been shown to stimulate migration in nonepithelial cells, but studies in intestinal epithelium demonstrate that it inhibits restitution by activating TLR4. The PRR RIG-I, which senses double-stranded self and nonself nucleic acids, is a positive regulator of restitution. Though the signaling mechanisms are unclear, RIG-I may promote migration by directly associating with the actin cytoskeleton at cellular junctions.
29.6.3
Microbiome
In addition to the effects of microbial fermentation products (i.e., SCFAs), the recognition of specific microbial patterns, originating from both commensals and pathogens, by the intestinal epithelium has a major influence on restitution. Deletion of intestinal epithelial “sensors” of microbial-associated molecular patterns, such as the toll-like receptor (TLR) family, leads to worsened epithelial outcomes in mouse colitic injury models. Ablation of TLR2, TLR4, TLR5, or TLR9 can worsen acute DSS colitis or cause spontaneous colitis. Treatment of mice with TLR9 agonist (bacterial DNA) improves recovery from radiation-induced intestinal injury. Removal of the MyD88 adaptor protein, critical for transduction of canonical downstream TLR signals, also results in worsened colitis. These studies have demonstrated that the worsened colitis in these genetic models partially results from impaired epithelial restitution. Mechanistically this is likely due to the role of TLRs in the normal expression of growth factor-like signals. In TLR9 knockout mice, production of amphiregulin, an EGFR ligand, and VEGF, both important factors for epithelial migration, are impaired. TLR2 knockout mice have reduced production of trefoil factors. TLR4 induces expression of EGFR ligands in response to bacterial challenge. It should be noted that some effects of TLR signaling on epithelial restitution may be context dependent. In newborn mice, TLR4 has also been shown to have negative effects on restitution by directly regulating FAK. In immature intestinal epithelial cells, the lipopolysaccharide (LPS) endotoxin present on Gram-negative bacteria and TLR4 ligand potently inhibits restitution in vitro and enhances the preservation of integrin-matrix focal adhesions.
How might individual commensals be signaling to promote restitution? The human bacterial commensal Lactobacillus rhamnosus GG (LGG), which is commonly used in probiotics and serves as a model experimental commensal, accelerates healing of intestinal wounds in the cell-culture scratch model and mouse DSS and biopsy injury models. Several molecular mechanisms have been elucidated to explain the effects of LGG. Detection of LGG and other bacterial formylated peptides by the intestinal epithelial formyl peptide receptors (e.g., FPR1, FPR2) results in the production of reactive oxygen species (ROS) through NADPH oxidase activity. ROS signaling in turn induces the oxidation of cysteines in the redox-sensitive tyrosine phosphatases LMW-PTP and SHP-2 and ultimately leads to increased phosphorylation of FAK. A second possible mechanism for LGG’s effects involves its secretion of two proteins, p75 and p40, that transactivate EGFR in colonic epithelial cells. Instead of directly binding to EGFR, LGG p40 stimulates the release of EGFR ligands, especially HB-EGF, by regulating the catalytic activity of ADAM17, a protein involved in cleaving membrane-bound EGF-like precursors to form active ligands. HB-EGF in turn stimulates epithelial migration through increased downstream expression and membrane polarization of α-5 and β-1 integrins, which leads to enhanced cell spreading.
The relationship between commensals and epithelial restitution is not just unidirectional. While commensal coculture with intestinal enteroids directly induces epithelial responses such as stem cell proliferation, mucosal signals also promote a permissive environment for certain prorestitutive commensals within a wound bed. After biopsy injury, FPR1 and NADPH oxidase enzymes within the mucosa generate an anaerobic environment that favors the expansion of Akkermansia anaerobes, among others. Members of this specific, transient wound-associated microbiome are then able to stimulate epithelial migration into the injured region through ROS signaling. Thus, epithelial cells communicate back to the microbiome as a way of enhancing wound repair.
Restitution is also promoted by eukaryotic commensals. Loss of CARD9, an adaptor protein that is involved in integration of signals downstream of pattern recognition receptors of fungi, results in mice that exhibit increased epithelial damage after DSS challenge. In addition, Saccharomyces boulardii is a nonpathogenic yeast that is associated with improved outcomes in gut health, including the prevention of diarrhea and treatment of IBD. S. boulardii secretes soluble factors that activate α2β1 integrin collagen receptors in intestinal epithelial cells, while reducing the interaction of αVβ5 integrin-mediated interaction with vitronectin, ultimately leading to the enhanced phosphorylation of motogenic signaling molecules such as FAK and paxillin.
29.6.4
Immune Cells and Signals
An intestinal ulcer exposes the underlying stromal tissue and mucosal immune system to luminal contents. An immune response is critical to prevent long-lasting penetration of microbes and toxins into and beyond the denuded area. The recruitment of immune cells, especially macrophages and neutrophils, to the injured region requires expression of cytokines and chemokines by epithelial cells. Epithelial restitution can thereby be regulated by autocrine signaling and by paracrine signaling of cytokines and growth factors secreted by the recruited immune cells (e.g., IL-15 ). In fact, removal of macrophages or changing their ability to secrete cytokines in vivo attenuates the proliferation of undifferentiated epithelial progenitors marking the adoption of the regenerative state and slows overall healing of ulcers. Interferon signaling in macrophages induces macrophage-specific apolipoprotein signaling that stimulates epithelial restitution in trans. Macrophages isolated from CD patients are less efficacious at stimulating epithelial restitution, an effect that has been attributed to their reduced secretion of HGF.
A central theme of the recent studies is that many cytokines traditionally associated with inflammation foster epithelial repair. This may seem paradoxical, because abnormally high expression of cytokines exaggerates the immune response and causes epithelial damage. Indeed, opposing effects are often seen with the same cytokine depending on the experimental model. These apparent discrepancies in the scientific literature are generally reconcilable, because a cytokine’s pro- or antirestitutive effect may depend on its concentration, combinatorial activation of target receptors, tissue source, cellular target, and overall context in which the cytokine is expressed. An example of cytokine duality with respect to epithelial restitution is TNF, which is produced by macrophages and monocytes. Modest TNF concentrations activate epithelial TNFR2 to stimulate restitution, but high TNF concentrations lead to preferential activation of TNFR1 and thus inhibit restitution. Mechanistically these dual outputs of TNF stimulation extend to downstream signaling events. TNFR2 activates cell migration through a Src family tyrosine kinase-dependent pathway. TNF also may either transactivate EGFR or downregulate EGFR signaling through increased internalization and sequestration of the receptor.
Cytokine receptors, whose expression was once thought to be mostly confined to immune cell subsets, are expressed by intestinal epithelia and contribute to epithelial responsiveness to cytokines in the context of restitution. In addition to the expression of TNF, macrophages and monocytes recruited to the wound area release IL-1, IL-2, and IFNγ, which activate the cognate epithelial receptor to promote migration through increasing available bioactive TGF-β peptides. The receptor for the Th17-inhibiting cytokine IL-27 is found on intestinal epithelium, and treatment with IL-27 enhances epithelial proliferation and migration. This effect is generated through a broad set of intermediaries, including p38 MAPK, ERK, AKT, STAT1, STAT3, and STAT6. Similar results are seen for IL-28 and its receptor. Likewise, intestinal epithelial cells express receptors for oncostatin M, a cytokine released by T cells, monocytes, and dendritic cells. Oncostatin M accelerates restitution in a STAT3-dependent manner. In contrast to the prorestitutive effects of cytokine signaling, IL-13/IL-4 receptors are expressed on epithelial cells; however, activation of these receptors with IL-13, an important cytokine in the pathogenesis of UC, reduced migration velocity in vitro. Certain cytokines may also act by upregulating expression of heterologous cytokine receptors on the epithelial cell surface. For example, IFNγ increases the apical epithelial polarization of the IL-10 receptor, whose activity is associated with reduced epithelial damage in DSS colitis.
Accumulating evidence points to a prominent role for the chemokine family of peptides, and their cognate G-protein coupled receptors, in control of intestinal restitution and repair. Chemokines have traditionally been viewed as chemoattractants for immune cells (for review see Ref. ), but they also exert important functions in wound healing. For example, injury and IL-1 stimulate epithelial cell release of CXCL8 (IL-8), which in turn stimulates colonocyte motility in vitro. CXCL12 binding to CXCR4 stimulates intestinal epithelial cell wound closure through a mechanism involving phospholipase C (PLC)-driven calcium mobilization and enhanced, ROCK-dependent localization of E-cadherin to the epithelial cell membrane, which potentially reduces the leakiness of the epithelium during migration. CCL20, acting through the CCR6 receptor, stimulates restitution via a pathway requiring phosphatidylinositol (PI) 3-kinase activity, calcium mobilization, and the small GTPase Rho; interestingly, the antimicrobial peptide human β-defensin also binds this receptor and produces the same response. It should be noted that chemokines can indirectly regulate restitution by triggering the recruitment of immune cells that themselves release prorestitutive signals.
Future studies will elucidate new roles for special types of mucosal immune cells in the modulation of intestinal epithelial restitution. While the proper balance of M1-polarized (proinflammatory) versus M2-polarized (antiinflammatory) macrophages has been documented for optimal tissue repair, recent studies have revealed that neutrophils also positively regulate restitution. Neutrophils are associated with severe epithelial damage, and their epithelial transmigration is hallmark of crypt abscesses and fulminant IBD. Neutrophils also are important for limiting pathogen invasion. However, in a wound healing context neutrophils secrete IL-22 in a IL-23-dependent manner. In turn, IL-22 binds to its cognate receptor expressed on intestinal epithelium and stimulates epithelial migration in vitro. The infiltration of IL-22-secreting neutrophils is associated with reduced animal susceptibility to experimental colitis. IL-22 may synergize with neutrophil-derived TGF-α and TGF-β1 in the promotion of mucosal healing. Innate lymphoid cells also have a demonstrated role in modulating epithelial repair. ILC2s secrete amphiregulin, an EGFR ligand that promotes restitution and protects against intestinal injury, in response to activation with IL-33. ILCs also secrete a host of cytokines that have been documented to promote restitution.
29.6.5
Extracellular Matrix and Stromal Cells
Homeostatic intestinal epithelial differentiation and stem cell function are directed by signals released by the subepithelial myofibroblasts lining the crypt. It is no surprise that these stromal support cells also have important functions in directing the repair process after intestinal injury and inflammation. Fibroblasts secrete growth factors and cytokines, such as EGF, TGFβ, keratinocyte growth factor, EGF, and acidic and basic FGFs, that promote epithelial migration. These signals converge and act through TGFβ signaling components in epithelial cells. After mechanical injury, stromal cells next to the ulcer secrete Wnt5a, a noncanonical Wnt ligand, that also potentiates epithelial TGFβ signaling, a process that is essential for epithelial regeneration. Stromal-derived prostaglandin synthesis after injury converts homeostatic epithelium the WAE phenotype. The mesenchymal signals leading to growth factor or prostaglandin secretion during times of challenge are of major interest, because these signals represent a potential avenue to modulate epithelial repair. For example, treatment of fibroblasts with GLP-2 potentiates their ability to promote epithelial restitution. This effect is reliant upon the mesenchymal secretion of VEGF and TGFβ. More recently, the function of mesenchymal microRNAs (miR-143/145) has been shown to be essential for epithelial regeneration after DSS injury; loss of the miR-143/145 cluster results in defective mesenchymal-to-epithelial IGF signaling, ultimately leading to reduced epithelial migration and proliferation after injury. Mesenchymal stem cells can also activate a targeted prorestitutive environment in stromal regions at sites of intestinal mucosal injury.
After injury, myofibroblasts remodel the ECM to create a permissive environment for restitution. The ECM functions as more than a surface or substrate for cell migration; it is a dynamic interface that modifies epithelial cell signaling and motility and is in turn modified by these cells. The immediate basement membrane is comprised primarily of collagen IV, laminins (mostly laminin V), fibronectin, and proteoglycans. The underlying interstitial matrix contains collagens I and III as well as some fibronectin, glycoproteins, and proteoglycans. In both cell and organ culture models, neutralizing antibodies against ECM components inhibit migration, and the same cell type moves at different rates on matrix of different composition. Furthermore, the composition of the matrix can determine not only a cell’s basal motility rate but also its responsiveness to growth factors for accelerated migration. For example, Caco-2 cell basal migration is more rapid on collagen I than laminin, but EGF and TGF-α stimulate migration only on laminin, not collagen I. Fibronectin, which is deposited at elevated levels during DSS colitis, enhances Caco-2 BBE cell restitution.
Although other ECM-binding molecules such as dipeptidyldipeptidase and the laminin receptor have been described, the best-characterized cellular receptors for ECM proteins are the integrins (for reviews of integrin biology, see Refs. ). These transmembrane proteins are heterodimers consisting of α and one β chain. Because multiple α and β chain types are expressed in cells, there is an array of potential receptor combinations. Laminins primarily engage α6β4 and α3β1 dimers. Collagen is recognized by α1-containing dimers, largely α1β1, α2β1, and α3β1, while fibronectin can bind a variety of dimers containing β1 chains (especially α5β1), αIIbβ3, αvβ6, and α4β7. Interestingly, overexpression of α5β1 integrin in Caco-2 cells results in fibronectin-driven EGFR phosphorylation. The positive effect of HB-EGF on restitution can be partially attributed to increased expression of α5β1 integrin. Consistent with experiments blocking ECM constituents, neutralization of integrins can selectively block basal or growth factor-enhanced motility on specific matrix components. Studies of laminin-driven migration, for example, show that neutralizing antibodies to α3β1 and to α6 integrin inhibit lamellipodial formation in and restitution of wounded T84 cells on laminin. Individual chemokines can also change the preferential attachment substrate for epithelial cells in the context of migration. For example, CXCL12 increases the proportion of laminin-binding integrins on the cell surface. It remains to be uncovered how specialized sets of cytokines and chemokines (e.g., Th1 vs. Th2) may coordinate with specific stromal reactions to generate an optimal microenvironment for epithelial restitution after diverse forms of injury.
Integrins are linked to the actin cytoskeleton through intracellular complexes involving α-actinin, talin, and vinculin. Integrin-driven signaling through these complexes allows for transmission of extracellular data (e.g., force, composition of the matrix) to the intracellular mechanisms responsible for remodeling the actin cytoskeleton in restitution. Signaling molecules associated with focal adhesions, such as FAK, Cas, and Src also bridge the communication between integrins and intracellular phosphorylation and signaling cascades. Global changes in cytoskeletal and cytoplasmic tyrosine phosphorylation are seen with the adoption of the restitutive cellular phenotype, and these changes are coordinated by the ECM.
In addition to receiving integrin-mediated signals from the ECM and surrounding microenvironment, which are translated to changes in cell behavior, epithelial cells feed information back to the extracellular environment to modify their migrating environment. This “inside-out signaling” utilizes many of the same pathways, which are themselves the targets of outside-in signaling. Integrin affinity and avidity for the ECM are regulated by physical stress from the cytoskeleton, modification by tyrosine kinase activity, paxillin binding, PLC γ, or PKC activation, as well as small GTPases. EGFR ligands stimulate expression and partner reorganization of particular α-integrin subunits in a matrix-dependent manner.
Additionally, cells constantly modify their environment either by direct release of ECM components and ECM-digesting proteases or by shedding peptides, which stimulate ECM remodeling by nearby myofibroblasts. Cultured human intestinal crypt cells stimulated with a combination of TNF and IFN-γ produce elevated levels of laminin-5 and -10. TGF-β can stimulate production of basement membrane components and integrin subunits. Inside-out and outside-in signals form a complex network signaling through common mediators; for example, integrin-linked kinase, which participates in ECM-initiated signaling events, is also required for basal fibronectin deposition by Caco-2 cells. Thus, intestinal epithelial cells involved in restitution can modify their own affinity for the basement membrane as well as modify that matrix directly or modulate the function of mesenchymal cells to yield production of an appropriate ECM.
29.7
Importance of Mucosal Restitution and Repair
29.7.1
Restitution and Mucosal Healing in Disease
The intestinal epithelial barrier is constantly exposed to injurious stimuli derived from dietary, microbial, iatrogenic, and other environmental sources. Failure to repair properly small intestinal ulcerations can lead to chronic disease and death. Improper exposure of luminal and crypt-associated microbes can induce aberrant immune responses that are hallmarks of chronic inflammatory diseases of the intestinal tract, such as the inflammatory bowel diseases Crohn’s disease and ulcerative colitis. Genome-wide association studies have identified polymorphisms in restitution-associated genes as significant contributors to the risk of developing IBD. Epithelial wound repair is a major but underserved therapeutic target in treatment of IBD, necrotizing enterocolitis, and chemotherapy-associated mucosal injury (mucositis). Enhancing restitution, or restoring normal restitutive processes, may help break the vicious cycle of injury and inflammation that characterizes these conditions. Because complications of these diseases include intestinal perforation and sepsis, halting the disease progression through restitution may prevent their associated fatalities.
Increasing evidence has shown increased intestinal permeability to be a hallmark and risk factor for developing a variety of extraintestinal pathologies. These diseases include autism, type I and type II diabetes, heart disease, multiple sclerosis, rheumatoid arthritis, and schizophrenia. Presumably the dysregulated exposure of the immune system to the luminal contents of the gut leads to altered cytokine signaling, priming the body for the development of these diseases, which all have significant immune pathogenesis. It is not clear how much defective restitution contributes to the overall observation of increased intestinal permeability; dysregulation of junctional proteins may be an important mechanism. Utilization of dye molecules of various molecular weights may help resolve whether the increased permeability is due to small (lack of tight junction integrity) or large (impaired restitution) breaks in the epithelium.
29.7.2
Pharmacological Targeting of Intestinal Restitution
Despite the therapeutic promise of targeting epithelial restitution in multiple GI diseases, the search for the optimal pharmacological approach continues. A major challenge is that antiinflammatory medications such as corticosteroids and NSAIDs have a negative effect on mucosal restitution. Finding adjunctive therapies that can restore normal healing processes during corticosteroid treatment, or discovery of substitute antiinflammatories that are neutral with respect to restitution, may lead to better outcomes in patients of diseases exhibiting chronic mucosal damage. For example, certain molecular targets of NSAIDs may have prorestitutive functions; specific activation of these targets may be key in the development of new therapies.
Because the intestinal lumen contains multiple sources of promigratory biomolecules, supplemental delivery of these compounds holds promise for enhancing restitutive responses. EGF enemas have been shown to be effective in the induction of remission in UC. Similar results have been demonstrated with butyrate enemas. Systemic or oral treatment with trefoil factors has demonstrated improvements in chemical colitis models in mice, but small-scale clinical trials in UC have not been conclusive. Improved delivery of luminal agents through genetic engineering of probiotics represents a promising therapeutic avenue. There is an ideological concern of increased carcinogenesis associated with prolonged enhancement of cell migration and proliferation. However, preclinical studies have suggested that successful wound healing overall prevents the development of injury-associated cancer, even when pathways associated with cancer in other organ systems, such as the EGFR pathway, are targeted.
Some approaches based on repositioning of current drugs have shown promise in preclinical studies. Rebamipide and ecabet sodium, commonly used for treatment of stomach ulcers, directly accelerate migration and wound closure by rat intestinal epithelial cells. Alteration of epigenetic profile by trichostatin A generates a restitutive gene expression profile in intestinal epithelium. Similarly, trials with keratinocyte growth factor (FGF-7), which is upregulated in intestine and skin following injury, have demonstrated protective activity against the development of oral mucositis in chemoradiotherapy patients. Specific dietary elements, such as polyamines, glutamine, and arginine, have positive effects on restitution and improved outcomes of intestinal injury in animals. However, other dietary components, such as omega-3 fatty acids, have reported negative effects on restitution. Naturopathic chemicals such as krill oil, root herbs, and tonics used in alternative medicine have also been demonstrated to enhance intestinal epithelial restitution.
An intriguing source of prorestitutive agents is milk. Certain peptides such as the milk-fat globule-epidermal growth factor 8 (MFG-E8, also known as lactadherin) protein found in breastmilk have recently been shown to promote intestinal epithelial migration. The amounts of MFG-E8 are decreased in ulcerative colitis, suggesting that restoration of its function may ameliorate the human disease through an epithelial mechanism. In addition, human breastmilk contains trefoil factors and EGF that have an established positive role in wound healing. Bovine β-casofensin, a peptide found in cow’s milk, also stimulates epithelial migration, with no effects on cell proliferation. Formula substitution (and deprivation of milk) in rodent pups is a risk factor for the development of necrotizing enterocolitis-like injury, which may partially result from lack of milk-derived protective factors in the context of restitutive signaling after hypoxic injury.
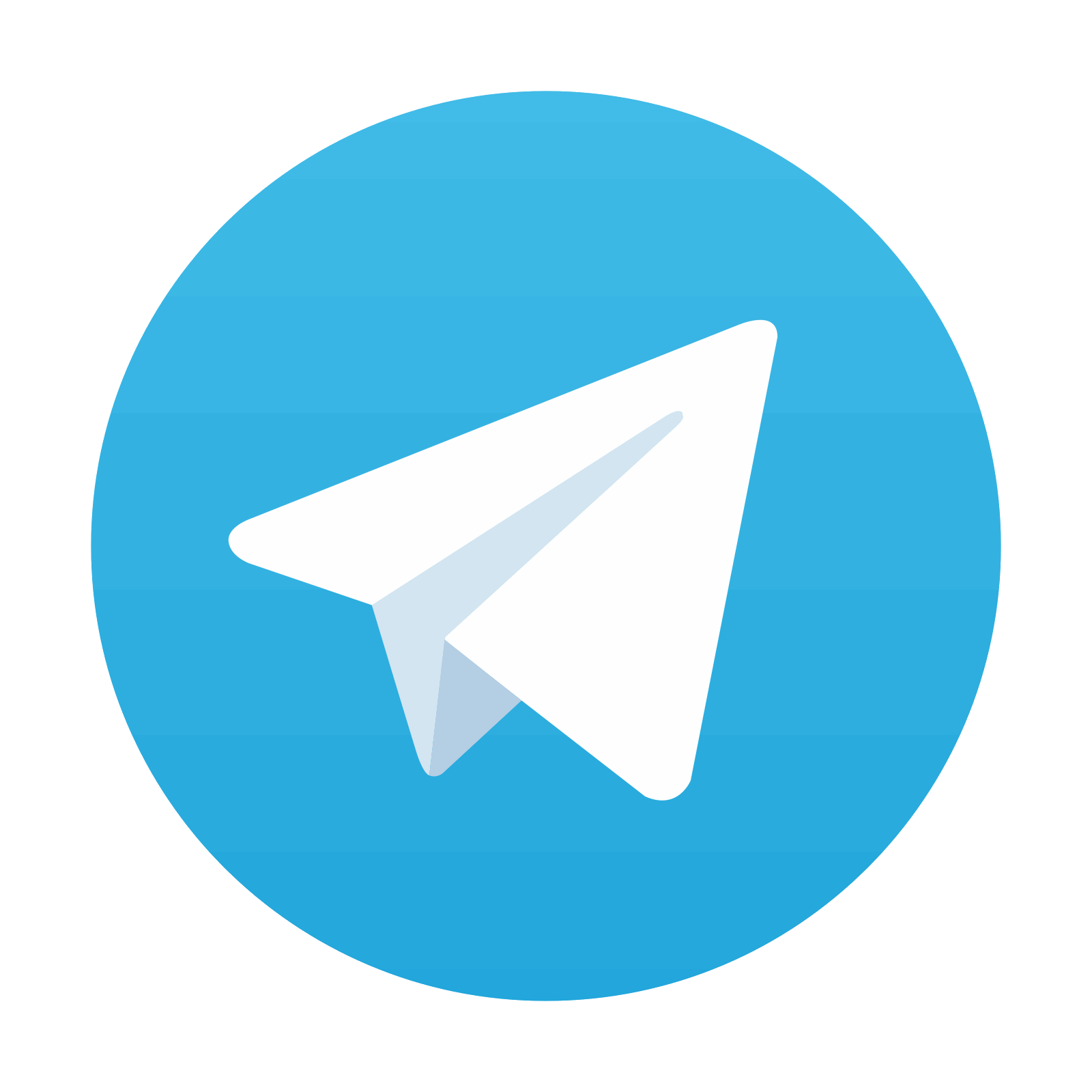
Stay updated, free articles. Join our Telegram channel

Full access? Get Clinical Tree
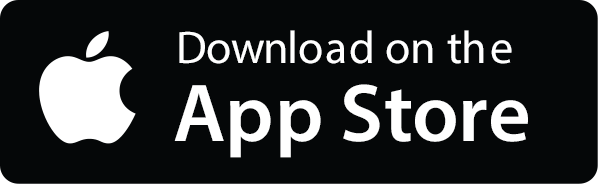
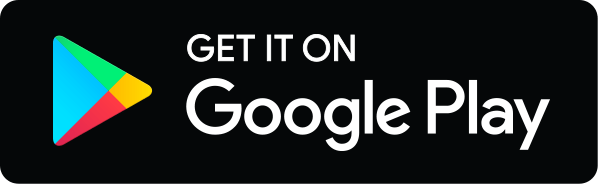