T cell transfer
Genetic
Spontaneous
Bacterial
CD45RBhigh → RAG−/− (SB + C)
WT → CD3εtg
IL-2−/−
IL-2Rα−/−
IL-10−/−
TCR−/−
Gαi2−/−
Mdr1a−/−
TNFΔARE (SB)
STAT3−/−
STAT4 tg
CD40L tg
A20−/−
TGFβ1−/−
TGFβR2−/−
N-Cadherin DN
GPx 1 + 2−/−
EP4−/−
R59D-JAB tg (SOCs-1 tg)
WASP−/−
MUC2−/−
IL10R2 × TGFβR DN
IEC/IKK−/−
Tbet−/− × RAG−/−(TRUC)
CD4+/TGFβRII DN
IL-7 tg
SAMP1/Yit (SB)
C3H/HeJBr
H. hepaticus
In retrospect, these results are not surprising given the differences in the immunopathogenetic mechanisms that promote intestinal inflammation in the DSS and TNBS models. For example, it is well known that the adaptive immune system is not required for development of DSS- or TNBS-induced colitis as distal bowel inflammation occurs in the absence of T and B cells [24, 25]. Indeed, recent studies have demonstrated that lymphocyte homing plays virtually not role in the pathogenesis of DSS- or TNBS-induced colitis [26–28]. There is also convincing evidence that the acute/self-limiting inflammation is a secondary response following the erosive and nonspecific injury of the colonic epithelium. Although these models are excellent in vivo systems for investigating wound responses in the gut, very few preclinical studies using these mouse models actually demonstrate how various pharmacologic agents or genetic manipulations affect epithelial cell restitution, proliferation, and/or mucosal barrier repair [1]. It is very likely that the vast majority of reports demonstrating anti-inflammatory properties of different therapeutics or experimental maneuvers may in fact be the result of more effective repair of the mucosal epithelium. When viewed in context of our current understanding of the immunopathogenesis of human IBD, these data indicate that erosive, self-limiting models of colitis may be of limited value for preclinical studies.
Other factors that have been suggested to limit the translation of preclinical data is the use of inbred strains of mice as surrogates for heterogeneous human populations as well as differences in intestinal microbiota [1, 16]. Flawed experimental design and/or data analyses as well as publication bias have also received a great deal of attention as factors that limit effective translation of preclinical studies to disease treatment [1–7, 29]. Furthermore, the inability of investigators within the academic and pharmaceutical/biotechnology communities to reproduce published studies demonstrating therapeutic efficacy of novel, small molecules or biologics in mouse models of disease has received a great deal of discussion in recent months. This is a particularly troubling situation that has garnered a great deal of attention by funding agencies and the publishing community [1, 2, 30–35]. In a recent review of the quality of methods reported in 58 studies using four of the most popular mouse models of IBD, Bramhall et al. reported that <2 % of these articles described all of the criteria that are considered to be essential for promoting reproducibility of mouse models of IBD [36]. They noted that while many of the studies described some of the criteria in reasonable detail, animal age, gender, housing conditions, and mortality/morbidity were poorly reported. When taken together, it appears clear that in order to more efficiently utilize the limited resources available for preclinical studies and accelerate the bench-to-bedside transition for promising therapeutics, investigators should utilize well-characterized, models of chronic intestinal inflammation that more closely mimics the immunopathology of human IBD [1, 16, 35, 37].
Mouse Models of Chronic Intestinal Inflammation
No single mouse model of chronic intestinal inflammation completely recapitulates the clinical and histopathological characteristics of human IBD; however, a number of different mouse models of chronic small and/or large bowel inflammation have been developed over the past two decades that have greatly advanced our understanding of disease pathogenesis (Table 15.1). Data obtained from numerous studies using a variety of chronic models have revealed several recurring themes. First, chronic gut inflammation requires the presence of a functioning adaptive immune system. Second, intestinal microbiota are required for the induction and/or perpetuation of chronic gut inflammation. Third, defective immuno-regulation of mucosal immune responses induces chronic intestinal inflammation and finally, the onset and severity of inflammation may be greatly dependent upon the genetic background of the animal. Taken together, these studies suggest that chronic intestinal inflammation develops as a result of a dysregulated immune response to components of the intestinal microbiota [38–40]. In addition, these data strongly suggest that mouse models of chronic gut inflammation are in general, much more relevant to human IBD than are acute/self-limiting models. Of the many mouse models of chronic gut inflammation that have been developed, a few of these have been used by sufficient numbers of investigators to yield detailed information on the penetrance, severity and pathogenesis of the inflammation. Below, we discuss a few of these models focusing on the immunopathology and their use in preclinical studies.
Mouse Models of Chronic Colitis
One of the best-characterized mouse models of chronic colitis is the CD45RBhigh T cell transfer model (Table 15.1) [41, 42]. Adoptive transfer of antigen inexperienced (i.e., naïve) CD4+CD45RBhigh T cells from healthy wild type mice into syngeneic, lymphopenic recipients results in the generation and expansion of large numbers of Th1 and Th17 effector cells but few, if any, regulatory T cells (Tregs; CD4+Foxp3+) [42]. This unregulated immune response requires the presence of intestinal microbiota and ultimately induces chronic and unrelenting pancolitis at 6–8 weeks following T cell transfer [41–43]. This model illustrates the concept that in the absence of effective immunoregulation (due to the paucity of Tregs), naïve T cells undergo microbial antigen-driven priming, polarization, and expansion to yield large numbers of colitogenic effector cells within the gut-draining mesenteric lymph nodes (MLNs ) and colon [39, 41–43]. Because naïve T cells will, by default, convert to disease-producing effector cells in the absence of Tregs, virtually any T cell deficient recipient (e.g., SCID, RAG-1−/−, RAG-2−/−, nude, CD3−/−, TCRβ−/− × TCRδ−/−) can be used as recipients [39, 42, 44]. We have also observed that the presence of B cells delays modestly the onset but not severity of colitis supporting the concept that B cells may act to suppress colonic inflammation in mice [43, 45, 46]. Histopathological analysis of colons obtained from mice with active disease reveals transmural inflammation, epithelial cell hyperplasia, PMN and mononuclear leukocyte infiltration, occasional crypt abscesses and epithelial cell erosions. Depending upon the strain of the donor and recipient, reconstituted mice may exhibit varying degrees of weight loss, diarrhea/loose stools, and severity of disease. Historically, the T-cell transfer model has been described as a Th1 model of colitis because of the large increase in IFN-γ production within the inflamed tissue [43]. However, we and others have found that the inflammation induced in this model represents a mixed Th1/Th17 inflammation because CD4+ T cells isolated from the spleen, mesenteric lymph nodes, and colonic lamina propria of colitic mice produce IFN-γ, IL-17 or both cytokines.
The original reports describing this model suggested that the inflammation was largely confined to the colon; however, more recent data demonstrates that the transfer of naïve T cells into RAG-1−/− (or TCRβ−/− × δ−/−) mice induces both colitis and small bowel inflammation [43, 47, 48]. We and others have found that the small intestine of these reconstituted RAG-1−/− mice exhibited loss of goblet and Paneth cells along the entire length of small intestine, which was most noticeable in the distal portion [43]. This model may prove useful for investigators who wish to ascertain whether the immunological mechanisms responsible for colitis are similar to those that induce inflammation of the small intestine. The reasons for the relative paucity of reports by other laboratories describing small intestine inflammation in this model are not known; however, most of the original studies used SCID recipients whereas we have used RAG-1−/− mice. It is well known that SCID mice are “leaky” in that they can begin to develop T and B cells with age which may influence the development of intestinal inflammation. In addition to the small intestine, reconstitution of RAG-1−/− mice with naïve T cells induces chronic liver and lung inflammation. We have observed extensive periportal and lobular lymphocytic inflammation, hepatocellular necrosis but no bile duct damage [42]. In addition, we and others have found that the majority of colitic mice develop chronic bronchitis/pulmonary inflammation [1, 49]. In the majority of mice, we observed confluent inflammation merged together between small and medium sized bronchi. Varying degrees of perivascular lymphocytic cuffing were also observed in virtually all of the mice; however, few PMNs were observed and vasculitis was absent.
The major advantages of this model are that one may examine the earliest immunological events associated with the induction of gut inflammation as well as the time-dependent development of disease. In addition, chronic colitis can be attenuated by treatment with a variety of different pharmacologic, immunologic, and biologic treatment protocols (Table 15.2) [8, 10, 50–57]. Furthermore, this model has been proven to be very useful to study the role that Tregs play in suppressing or limiting the onset and/or severity of colonic inflammation [58–61]. Te Velde and coworkers have found, using genome-wide gene expression profiling of inflamed colons, that the pattern of gene expression in the CD45RBhigh T cell transfer model most closely reflects the altered expression profile observed in human IBD when compared to the erosive, self-limiting models of colitis [20, 62, 63]. Disadvantages of this model include the expense and the requirement for cell sorting. In our experience, differences in animal housing, food and water may influence greatly the onset and severity of disease. It has also been suggested that homeostatic proliferation of disease-producing T cells in lymphopenic recipients may not accurately represent the immunopathological mechanisms responsible for human disease [58]. However, a number of features of this model strongly suggest that the development of chronic colitis is not simply the result of homeostatic proliferation of T cells in a lymphopenic environment. For example, colitis does not develop following transfer of naïve T cells into antibiotic-treated or germ-free recipients [58]. These observations together with those demonstrating reduced accumulation of naïve T cells suggest that pathology is driven by intestinal bacterial antigens and not by “space” [58]. In addition, there is a great deal of evidence demonstrating a strong correlation between genes involved in the immunopathogenesis of chronic colitis in the T cell transfer model and those linked to human susceptibility alleles from genome wide association studies performed on patients with CD suggesting that this model may in fact represent a very good model for preclinical studies [58].
Table 15.2
Pharmacological studies using mouse models of chronic gut inflammation
Model | Therapeutic | Efficacy |
---|---|---|
IL-10−/− | TNF/TNFR antisense IL-6/IL-6R mAb IFNγ/IFNγR mAb IL-12/IL-23 mAb | Yes Yes Depends on timing Yes |
CD45RBhigh → SCID/RAG | TNF mAb OX40L mAb B7-H1 mAb CD70 mAb Antiangiogenic peptide FTY720 FASL mAb Dexamethasone/prednisolone Azathioprine Sulfasalazine/5-ASA Cyclosporine Tacrolimus TNF/TNFR mAb Integrins mAb (β7, MAC-1, LFA-1, α4) IL-6/IL-6R mAb IFNγ/IFNγR mAb IL-12/IL-23 mAb IL-17 mAb | Yes Yes Yes Yes Yes Yes No Yes No No No No Yes Yes Yes Yes Yes No |
TNFΔARE | CCR6 mAb Integrins mAb (β7) TNF/TNFR mAb IL-12/IL-23 mAb IFNγ mAb | No Yes Yes Yes Yes |
SAMP1/Yitc | Dexamethasone TNF/TNFR mAb Integrins mAb (β7, MAC-1, LFA-1, α4) IL-6/IL-6R mAb IFNγ/IFNγR mAb IL-12/IL-23 mAb CCR9 mAb | Yes Yes Yes Yes Yes Yes Yes |
A second, widely used model of chronic colitis is the interleukin-10 deficient (IL-10−/−) model (Table 15.1). Mice with targeted disruption (i.e., deletion) of the IL10 gene develop spontaneous pancolitis and cecal inflammation by 2–4 months of age. Again, this model of chronic colitis illustrates the recurrent paradigm that defective immunoregulation, via the loss of IL-10 production, leads to a dysregulated immune response to intestinal microbiota resulting in the development of chronic inflammation [64, 65]. Histopathological analysis of colons obtained from mice with active disease show many of the same characteristics as those observed in human CD including transmural infiltration of mononuclear leukocytes, T cells, and plasma cells. The genetic background of the mouse is a major modifier of disease with disease penetrance and severity occurring to a much greater extent in 129SvEv IL-10−/− and Balb/c IL-10−/− mice when compared to the C57Bl/6 IL-10−/− strain. The advantage of using the IL-10−/− model is that it is a well-established Th1 model of transmural colitis and cecal inflammation that can be attenuated by administration of various pharmacologic, immunologic, and biologic agents (Table 15.2) [8, 10]. A disadvantage of this model is the onset and severity of disease may be quite variable and in some cases requires several months to develop.
The variability in penetrance and severity as well as the delayed onset of disease in genetically engineered models of chronic colitis involving single mutations of specific genes (e.g., IL-10−/− model) limit an investigator’s ability to more fully characterize the time course and immunopathogenesis of disease, as well as evaluate new therapeutic strategies for treatment of chronic colitis. Indeed, investigators have demonstrated that genetic manipulations in two (or more) loci appear to enhance the penetrance of robust colitis as well as accelerate the onset of disease [8, 66, 67]. Recent data derived from genome-wide association studies have identified ~163 different susceptibility loci for CD and/or UC [68]. Of these, alterations in genes involved in IL-10 and TGFβ signaling have been described with polymorphisms in each increasing modestly the risk of developing disease [69]. Furthermore, recent clinical studies have determined that mutations in the IL-10 receptor (IL-10R) results in very early onset IBD in young children [70]. Capitalizing on these observations, Kang et al. generated a novel, “2 hit” mouse model of fulminant and unrelenting colitis [67]. To do this, investigators bred dominant negative TGFβRII (dnTGFβRII) mice with IL-10R2−/− mice to generate dnTGFβRII × IL-10R2−/− offspring referred to as dnKO mice. These offspring have defective IL-10R2 signaling in all tissues and TGFβ signaling only in T cells [67]. Compared to their littermate controls (i.e., IL-10R2−/− or dnTGFβRII), dnKO mice fail to gain weight following birth and develop an accelerated and severe colitis with a 100 % penetrance by 4 weeks of age. Kang et al. show that broad spectrum antibiotics rescued the dnKO offspring, thereby preventing the development of colitis [67]. Additional studies by this same group show that an isolate of Bacteroides but not Enterobacteriaceae induces robust disease in dnKO but not controls [66, 69]. Taken together, these studies clearly demonstrate the need to assess colitogenic potential rather than relying solely on 16S rRNA quantification. Although this model represents a major advancement in mouse models of IBD using IBD-relevant deficiencies in IL-10 and TGFβ signaling, the time commitment and resources necessary to generate sufficient dnKO offspring for studying immunopathogenesis or performing pharmacologic intervention studies are substantially higher than for other mouse models.
Another, well-characterized mouse model of chronic colitis that has been used by different groups of investigators is the multidrug resistance pump 1a-deficient (Mdr1a−/−) mouse (Table 15.1) [71]. Mdr1a is a P-glycoprotein expressed on the surface of several different cell populations including intestinal epithelial cells, T cells, monocytes, macrophages, natural killer cells, dendritic cells, and brain microvascular endothelial cells . This transport protein pumps small amphiphilic and hydrophobic molecules across the cell membrane in an ATP-dependent manner. Of note is that the mdr1a gene is located on chromosome 7q21.1 which is a susceptibility locus for human IBD [71]. Approximately 25 % of the Mdr1a–/– mice develop colitis beginning at 8–12 weeks of age when housed under specific pathogen-free conditions [71, 72]. The colonic inflammation can be delayed for up to 16 weeks of age by the administration of antibiotics [72] or eliminated when raised under germ-free housing conditions demonstrating yet another model of IBD where inflammation is dependent upon the presence of commensal bacteria. Histopathological analysis of colons obtained from these mice reveals an inflammation that is similar to human UC. There is substantial thickening of the mucosa, extensive infiltration of inflammatory cells into the lamina propria and occasional crypt abscesses and ulcerations. The mechanisms by which ablation of the mdr1a gene induces chronic gut inflammation are not known at the present time; however, it is known that the induction of colitis in these mice is dependent upon defective Mdr1a activity on the intestinal epithelial cells and not the lymphocytes [72]. It may be that the lack of intestinal epithelial cell Mdr1 results in increased accumulation of enteric antigens within these cells. Antigen-loaded cells could then process and present these microbial antigens to T cells immediately underlying the epithelium, thereby priming the T cells to become “hyper-reactive” to the enteric microbiota thereby driving colonic inflammation [71, 72]. More recent studies have demonstrated a significant dysregulation of expression of cytokines and chemokines prior to onset of colitis in young Mdr1a−/− mice. These data suggest that the intestinal immune system in these mice may be in a continuous state of immune activation and thus are particularly susceptible to induction of chronic gut inflammation [71].
Collett et al. [73] have shown that older FVB.mdr1a−/− mice (12–16 weeks) have increased colonic mucosal permeability that is associated with tissue inflammation. Tissue from these mice reveal an upregulation in genes involved in bacterial recognition, a downregulation in anti-inflammatory pancreatitis-associated protein (PAP) and RegIIIγ genes and appear to be more responsive to lipopolysaccharide (LPS). Interestingly, although younger Mdr1a−/− mice (4–5 weeks) lack histological evidence of inflammation or increased permeability, they have increased cytokine and chemokine secretion (IFNγ, MIP2, TNFα, and IL1β) that is similar to that of older mice with active colitis [73]. These data could suggest an inappropriate immune response to luminal antigens prior to the induction of chronic inflammation. It has also been shown that FVB.mdr1a−/− mice have decreased amounts of inducible Tregs (iTregs; CD4+Foxp3+) in the intestinal lamina propria and Peyer’s patches prior to and following the onset of colonic inflammation [74]. P-glycoprotein (P-gp) was found to be expressed in these cells and it appears to be restricting the development of iTregs from naïve FVB.mdr1a T cells, as demonstrated both, in vivo and in vitro [74]. P-gp deficiency phenotype conferred by knocking out of the Mdr1a−/− gene has been shown to be modified by the genetic background strain [75]. When the FVB.mdr1a strain is crossed with a C57BL/6 mouse (C57BL/6.mdrla deficient), it no longer develops spontaneous colitis nor is it sensitive to the induction of colitis by piroxicam or Helicobacter bilis. However, this strain shows an increased susceptibility to DSS-induced colitis, which has been attributed to the possible impaired cell cycling associated with P-gp deficiency that then affects wound repair [75, 76].
In order to further investigate the effect of other genes associated with human IBD, Ey et al. produced the Mdr1a−/−TLR2−/− double knockout on the FVB strain. TLR2 is another susceptibility gene identified by GWAS in UC patients and it has been associated with a more severe disease phenotype [37, 77]. The inflammation in this model is spontaneous in nature and is characterized by an excessive release of IL-1β that requires MD2- and MyD88 -mediated signaling. Colonic inflammation is characterized by mucosal thickening and inflammatory cell infiltration beginning at 5 weeks of age, which represents an acceleration in disease onset and severity when compared to Mdr1a−/− animals. Deletion of the MyD88 gene in these mice to create triple knockout mice lacking all three genes (Mdr1a−/−TLR2−/−MyD88−/−) prevents induction of colonic inflammation suggesting that MyD88-dependent signaling is required for induction of disease in Mdr1a−/−TLR2−/− mice [37].
The large majority of mouse models displaying chronic colitis require the participation of the adaptive immune system for expression of disease. However, recent evidence suggests that innate immune cell activation alone is capable of inducing and perpetuating protracted colonic inflammation. For example, Maloy et al. have developed a mouse model of innate immune cell-mediated chronic colitis that is induced by associating lymphopenic RAG-1 or -2−/− animals with the Helicobacter hepaticus (Hp; Table 15.1) [13, 78, 79]. Although Hp does not normally induce chronic gut inflammation in healthy/immunocompetent mice, introduction of this Gram negative, spiral bacterium into RAG-2−/− animals establishes a lifelong colonization that ultimately induces chronic cecal and colonic inflammation (typhlocolitis) [78, 79]. This IL-23-driven inflammation is characterized by epithelial cell hyperplasia and extensive infiltration of PMNs and monocytes which is more severe in the cecum than in the colon [78, 80]. A recent variation of this model has been described in which chronic colitis can be induced in immunocompetent mice by combining the introduction of Hp with systemic administration of a mAb to IL10R [81–83].
Another interesting model of chronic colitis driven by innate immune cells has been described by Garrett and coworkers [84]. These investigators demonstrated that the ablation of T-bet (a T-box transcription factor family member) in RAG-2−/− mice results in the development of spontaneous and communicable colitis that is similar to human UC (Table 15.1). The colonic inflammation in these T-bet−/− × RAG-2−/− ulcerative colitis (TRUC) mice is characterized by remarkable PMN and monocyte inflammation, crypt abscesses, bowel wall thickening, erosions, and ulcerations. These investigators also demonstrated colonic epithelial barrier disruption prior to the onset of active colitis. The fact that colitis is communicable and will develop in wild type mice when housed with TRUC mice suggest the selection of a pathogenic microbiota that develops in response to immune defects and/or inflammation [84]. Indeed, these investigators characterized the intestinal microbiota in TRUC mice and found that the overabundance of Klebsiella pneumoniae and Proteus mirabilis correlated with the onset of disease. Furthermore, TRUC-associated bacterial strains in association with maternally transmitted microbiota have the ability to induce disease in WT and RAG−/− mice [85]. Not surprisingly, these two organisms, as well as other members of the Enterobacteriaceae family, have been shown to be increased in human IBD [85–87]. Certain species within Enterobacteriaceae are thought to thrive in the inflamed gut due to the ability of these facultative anaerobes to proliferate in the presence of small, but significant amounts of oxygen adjacent to the inflamed tissue [85, 88]. In addition, the relative overabundance of some members of the Enterobacteriaceae family may be due to their ability to utilize certain electron acceptors (e.g., tetrathionate and nitrate) that are present in the inflamed lumen for anaerobic respiration, thereby outcompeting obligate anaerobes [88–90]. Not only does the microbial structure differ during active colitis and treatment-induced remission, but so do the major metabolic functions of the microbiota [88]. Some of the major differences that are seen in microbial function during active colitis are their decreased ability to harvest energy (i.e., lipid metabolism, xenobiotics biodegradation, and metabolism) and dysregulated pathways of microbial signaling and processing [88].
In addition to chronic colitis, TRUC mice develop colitis-associated colorectal cancer (caCRC) that resembles human disease. Virtually all TRUC mice develop colonic dysplasia and adenocarcinoma (ADA) by 6 weeks of age [91]. Selective depletion of dendritic cells (DCs ) prevents inflammation and cancer progression in these mice, demonstrating the critical interplay between the innate immune system and intestinal microbiota in the induction of inflammation and associated malignant disease. As with other models of chronic colitis, disease incidence and severity in the TRUC model depends upon background strain. Ermann et al. showed that the severity of colitis in TRUC mice bred onto the C57BL/6 background (B6.TRUC) was less severe when compared to mice bred onto the BALB/c background (BALB/c.TRUC) [92]. These investigators found that when the suspected susceptibility locus Cdcs1 (cytokine deficiency-induced colitis susceptibility-1) was introduced into the B6.TRUC mice, severity of colitis was found to be similar to that observed in BALB/c.TRUC animals. The Cdcs1 locus, among others, has been described in the IL-10−/− and Gαi2−/− mouse models of chronic colitis and it is thought to control disease severity via its effects on innate immune cell function and maintenance of mucosal homeostasis [92, 93]. Although this model represents an important addition to the toolbox for IBD investigators who wish to examine the relationship among the innate immune system and chronic gut inflammation, it should be noted that currently, little or no evidence exists suggesting that human CD or UC can be transferred to healthy children or adults.
Models of Chronic Small Bowel Inflammation
In addition to the well-characterized models of chronic colitis described above, two models of spontaneous, small bowel inflammation are gaining increasing interest for studying the immunopathology of Crohn’s ileitis as well as for evaluating new drug therapies. One model is the homozygous TNF-overexpressing TNFΔARE/ΔARE mouse (Table 15.1). These mice were developed by targeted deletion of a 69 base-pair region of the AU-rich elements in the 3′ UTR region of the TNF-α gene [94]. The deletion results in increased TNF-α mRNA stability and enhanced TNF-α protein production. Mice possessing the deletion in the homozygous state display severe and rapid wasting disease and die within 1–3 months of age. These mice are runted in appearance, exhibit alopecia-like lesions, develop chronic and degenerative inflammatory arthritis and express severe inflammatory changes in the distal small bowel similar to CD by 4 weeks of age [94]. Interestingly, there appears to be a gene dosage effect as heterozygote littermates (HET; TNFΔARE/+) have a gradual development of inflammation and live at least 7–8 months, showing severe intestinal inflammation at 8 weeks of age [94]. HET mice models are useful because as in human disease, they show a malabsorption phenotype characterized by weight loss, increase fecal energy loss, fecal fat excretion and bone alterations possibly due to calcium malabsorption [95, 96]. Lesions consist of villous blunting (and broadening) associated with the transmural infiltration of acute and chronic inflammatory cells, including mononuclear leukocytes, plasma cells, and PMNs. This transmural inflammation extends deep into the muscular layers of the bowel wall, displaying characteristics of Crohn’s ileitis. The severity and location of the inflammation (ileum) in this model seems to be dependent on the dysbiotic microbiota, according to Schaubeck et al.[97]. When transferred into germ–free TNFΔARE/ΔARE mice, this dysbiotic microbiota induced Crohn’s-like ileitis and decreased antimicrobial lysozymes and defensins. This is yet another mouse model of IBD that demonstrates the role of intestinal microbiota in disease as antibiotic treatment or raising mice under germ-free conditions eliminates intestinal inflammation [97]. In addition, different environmental and dietary factors may alter the microbiota thereby altering the onset and/or severity of disease. For example, Wernet et al. have shown depletion of luminal iron alters the gut microbiota and prevents Crohn’s disease-like ileitis suggesting that luminal (oral) supplementation with iron for patients with IBD may be problematic [98]. The inflammation observed in this model can be treated with several biologics and immune-modifying drugs used in human medicine (Table 15.2). This model also offers the unique opportunity to examine the immunopathological mechanisms underlying TNF-induced ileitis and arthritis with the possibility of identifying new therapeutic strategies to treat patients with CD.
The SAMP1/Yit model of chronic ileitis is another model that is gaining increased attention and is readily available to interested investigators (Table 15.1). As with TNFΔARE/ΔARE mice, these animals are useful for studying the underlying mechanisms involved in the pathogenesis of Crohn’s ileitis and preclinical evaluation of new drug therapies. This model of spontaneous ileitis was originally generated by >20 generations of brother–sister mating of a senescence-accelerated mouse line [99]. SAMP1/Yit mice are unique in that 100 % of these animals develop spontaneous ileitis at ~30 weeks of age without any genetic, immunological or environmental manipulation. Histopathological analysis of these mice reveals robust and chronic ileitis as well as extraintestinal manifestations similar to human CD. The segmental inflammation is localized to the terminal ileum and displays transmural involvement and occasional granulomas. Chronic ileitis can be adoptively transferred by CD4+ T cells to SCID or RAG−/− recipients suggesting that activated CD4+ T cells home specifically to and recognize antigens within the terminal ileum [100]. Over the past few years, it has been found that with further brother–sister matings for an additional 20 generations, a new phenotype emerged in which chronic ileitis developed by 10 weeks of age with the occurrence of perianal disease, ulceration and fistula in a small subset of these mice as well as periodontal disease [101]. This substrain has been renamed SAMP1/YitFc and has been propagated through continued brother–sister mating [102]. Given its well-defined progression of disease in the SAMP1/YitFc model, it has shown to be very useful in studying the immunological changes before and during inflammation [103]. Pizzarro et al. demonstrated two clear phases of disease [103]: The inductive phase occurs between 4–7 weeks of age that is characterized by intense upregulation of Th1 and proinflammatory cytokines that precede histological evidence of overt inflammation. The chronic phase occurs at 9–10 weeks of age and is characterized by a Th1/Th2 response that correlates with robust intestinal inflammation [103]. It should be noted that the Tregs in this mouse model have been shown to be dysfunctional in vivo, whereas, these cells seem to have normal suppressive activity in vitro [104]. This paradoxical observation has also been made in human IBD in which investigators have noted an expansion of Tregs in the lamina propria of inflamed intestinal tissue obtained from CD patients [105]. Although these data imply Treg dysfunction, Ishikawa et al. and Fantini et al. have demonstrated normal suppressive activity of Tregs obtained from inflamed tissue in vitro [104] [106]. Fantini et al. further showed that the apparent lack of effect of Tregs in chronic gut inflammation is most likely due to resistance of colitogenic effector T cells to Treg-mediated suppression [106].
This model of chronic ileitis has also been helpful in defining the role of the innate immune system in chronic inflammation. As described above, it is widely believed the adaptive immune system plays a major role in induction and perpetuation of chronic intestinal inflammation. However, Corridoni et al. hypothesize that: “CD may occur as a deficit in innate immunity as opposed to an overly aggressive immune response” [107, 108]. It is also important to point out that although the microbiota seem to play a role in the SAMP/Yit model, it is not required for induction of disease as in other models given that some germ-free animals still develop small bowel inflammation [103]. Studies using the SAMP1/YitFc model have shown that immune-blockade of Th1- polarizing cytokines or certain T-cell adhesion molecules attenuate the severity of ileitis (Table 15.2). Thus, both the TNFΔARE/ΔARE and the SAMP1/YitFc mice provide the investigator with the unique ability to study the underlying immunopathological mechanisms responsible for the development of ileal inflammation.
Humanized Mouse Models of Autoimmune and Chronic Inflammatory Diseases
There is no question that mouse models have provided investigators with valuable information on the immunopathogenesis of chronic intestinal inflammation as well as other autoimmune and chronic inflammatory diseases [109–112]. Despite revealing a number of different targets and therapeutic strategies from preclinical studies, few new therapies have been demonstrated to be effective in well-controlled clinical trials. This is not entirely surprising given the fact that the structure and function of the mouse immune system differs substantially from humans [113, 114]. It has been estimated that >80 major differences exist between the mouse and human immune systems. [35, 114]. Thus, even with the use of standardized and reproducible mouse models of chronic gut inflammation, it may be difficult to accurately model human disease. In response to these concerns, investigators have been actively working to develop mice with a functional human immune system [35]. By utilizing long-term engraftment of the human hemato-lymphoid cells (e.g., hematopoietic stem cells or lymphocytes) in severely immunodeficient recipients, investigators have been successful in producing humanized mouse models of some acute and chronic inflammatory diseases. Furthermore, some of these models have been used to evaluate certain human-specific therapies in vivo. This approach has been particularly successful using humanized mouse models of infectious diseases (e.g., HIV) [29, 110, 112, 115]. In addition, great strides have been made in using humanized mice to study the pathogenesis and human-specific therapies in different chronic diseases such as diabetes, arthritis, graft-vs.-host disease, transplant rejection and cancer [109–112, 116–118].
A major breakthrough came in the humanization of the murine immune system with the discovery that targeted mutation of the IL-2 receptor common gamma chain (IL-2γ) in NOD/scid mice (termed NOD/scid-IL2rγ−/− or NSG mice) greatly enhanced the long-term engraftment of human hemato-lymphoid cells in these severely immunodeficient mice [35, 119–122]. It was well known that IL-2rγ was a required component for receptor complexes specific for IL-2, IL-4, IL-7, IL-9, IL-15, and IL-21 (Fig. 15.1) [123, 124]. In addition, studies had demonstrated that these cytokines are critical for generation of T, B and NK cells. Thus, deletion or inactivation of IL-2rγ created mice devoid of T, B and NK cells [125–127]. Several laboratories have developed different stocks of immunodeficient mice devoid of IL-2rγ that differ with respect to strain background and the type of IL-2rγ mutation (reviewed in 35). These novel platforms have been used to engraft different populations of human hemato-lymphoid cells, thereby producing three major models of humanized mice (Table 15.3). The reader is referred to recent reviews for more detailed discussions of these models [35, 128, 129]. It is theoretically possible to model most, if not all of the human autoimmune and chronic inflammatory diseases using one or more of the immunodeficient IL-2rγ−/− strains. Below is a brief overview of the generation, uses and limitations of the 3 major humanized mouse models.


Fig. 15.1
The IL-2 receptor common γ chain plays a critical role in the development of immune cells. The common γ chain is associated with the high affinity receptors for IL-2, IL-4, IL-7, IL-9, IL-15, and IL-21. These cytokines are produced by different immune cells, stromal cells, and epithelial cells. Cytokine-receptor signaling is critically important for the generation of different populations of immune cells (Modified from Ref. 127 with permission)
Table 15.3
Models of human hemato-lymphoid cell engraftment into immunodeficient IL-2rγ−/− mice
Model name | Description | Advantages | Applications | Limitations |
---|---|---|---|---|
Hu-PBMC-IL-2rγ −/− (Human Peripheral Blood Mononuclear Cells engrafted into lymphopenic IL-2rγ−/− mice) | Lymphopenic IL-2rγ−/− mice are injected with human peripheral mononuclear cells | • Technically simple model • Good engraftment of effector and memory T cells | • Induced models of inflammation (e.g., sepsis, arthritis, colitis) • Model of xenogeneic GVHD • Assess effector functions of T cells obtained from patients with RA, lupus, MS, diabetes or IBD • Human-specific infectious diseases (HIV) • Allograft tissue rejection | • Only activated/memory T cells are present • Lack of mature human B cells, myeloid cells, DCs, platelets and erythrocytes • Xenogeneic GVHD develops after 4–5 weeks due to human T cell reactivity against mouse MHC molecules • Limited primary immune responses |
Hu-HSC-IL-2rγ −/− (Human Hematopoietic Stem Cells engrafted into lymphopenic IL-2rγ−/− mice) | Lymphopenic IL-2rγ−/− mice are injected with CD34+ HSCs derived from fetal liver, cord blood, bone marrow, or from peripheral blood following G-CSF-mediated mobilization | • Generates a naïve human immune system • Development of T and B cells, APCs, myeloid cells, and NK cells | • Human hematopoiesis • Induced models of inflammation (e.g., sepsis, arthritis, colitis) • Engraft human HSCs from patients with autoimmune or chronic inflammatory diseases • Human-specific infectious diseases • Transplantation biology | • Low rate of T cell engraftment • Impaired T and B functions • No mucosal immune system • Lack of expression of human HLA within the thymus prevents education and development of HLA-restricted CD4+ and CD8+ T cells (DTH response is suboptimal) • Only small numbers of PMNs, RBCs, and megakaryocytes are present in the blood |
BLT-IL-2rγ −/− (Bone marrow, Liver, Thymus engrafted into lymphopenic IL-2rγ−/− mice) | Lymphopenic IL-2rγ−/− mice are implanted with small pieces of human fetal liver and autologous thymus under the renal capsule; the mice are then injected with human CD34+ HSCs purified from the same fetal liver sample | • Human immune system engraftment is much more robust than in the Hu-SRC-NSG model • Sustained high level of T cell development; T cells are educated by the human thymus and are HLA restricted • Produces human mucosal immune system | • Human hematopoiesis • Engraft human HSCs from patients with autoimmune or chronic inflammatory diseases • Human-specific infectious diseases • Transplantation biology | • Surgical expertise and fetal tissue are required • Responses to vaccination protocols are limited to IgM antibody production • A delayed xenogeneic GVHD (>4 months) occurs in these mice that results from the lack of negative selection against murine antigens in human thymus and/or to lack of peripheral regulation |
Engraftment of Human Peripheral Blood Mononuclear Cells into Immunodeficient Mice Devoid of IL-2rγ
There is a long history of attempts to engraft (via adoptive transfer) human peripheral mononuclear cells (PBMCs) into immunodeficient mice such as SCID or RAG-1−/− or -2−/− mice [35, 130]. However, very small numbers of T cells and few if any B cells engraft in this model due to the presence of a fully functional innate immune system that destroys xenogeneic (human) cells [131–133]. As pointed out above, the development and use of immunodeficient mice that lack IL-2rγ greatly enhanced the engraftment of T cells in these novel lymphopenic recipients devoid of T, B, and NK cells [112, 134–136]. This model, which we have termed the Hu-PBMC-IL-2rγ −/− model, has been used to investigate immunological mechanisms involved in allograft rejection, cancer, autoimmunity, regenerative medicine, and infectious diseases [29, 112, 116, 134, 137] (Table 15.3). This model has also been used to evaluate novel, human-specific therapies including cell-based therapies [138]. It should be noted that this model is particularly useful for investigating T cell-mediated immune responses following engraftment of PBMCs obtained from patients with autoimmune or chronic inflammation diseases; however, the usefulness of this model is limited by the development of lethal, xenogeneic graft versus host disease (GVHD) within 3–4 weeks post T cell engraftment [139]. King and coworkers discovered that GVHD developed as a result of aggressive immune responses of human T cells towards murine MHC class I and II [139]. A delay in GVHD in NSG mice devoid of murine MHC class I and II may prove useful in extending the observation period in the Hu-PBMC-IL-2rγ −/− model in future studies [139].
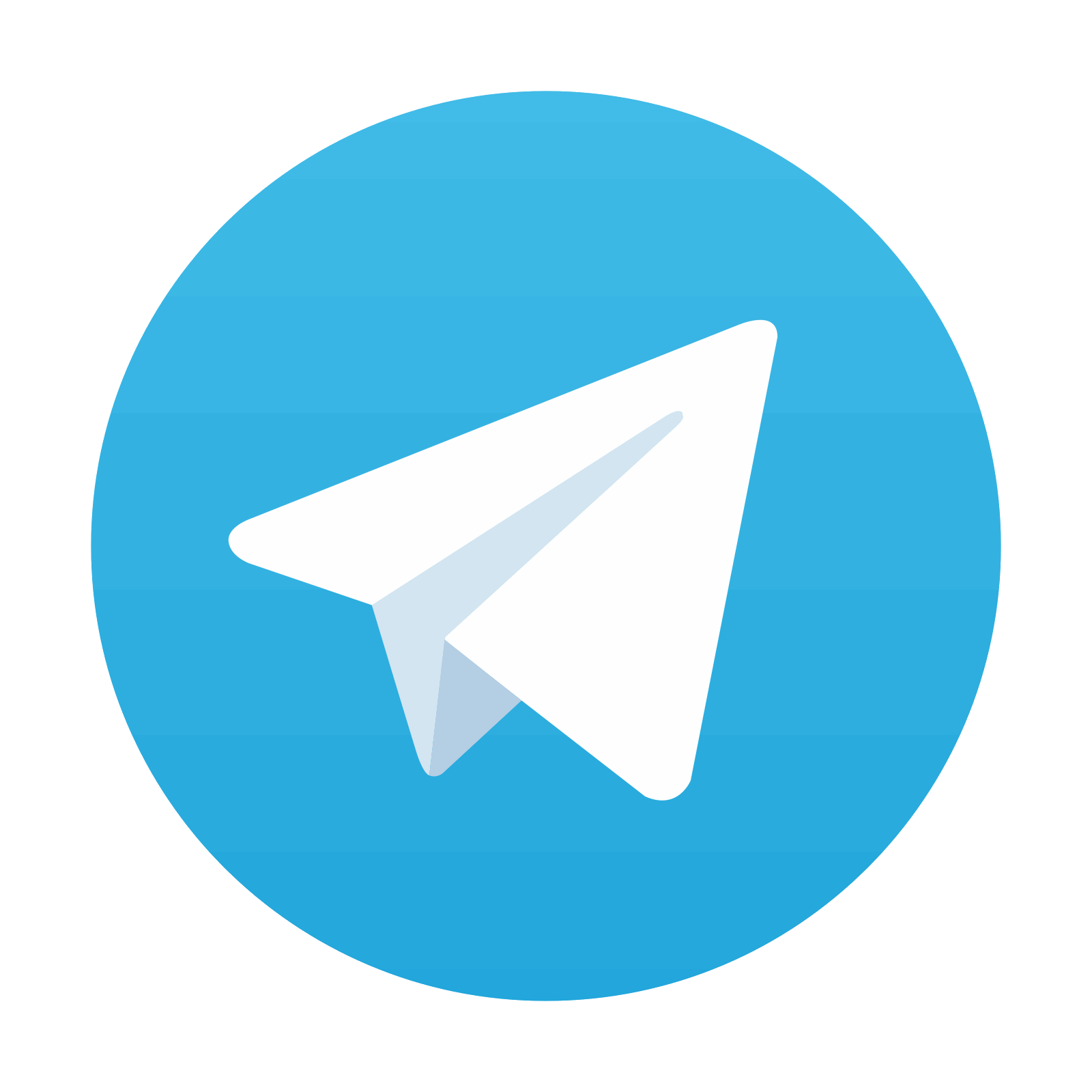
Stay updated, free articles. Join our Telegram channel

Full access? Get Clinical Tree
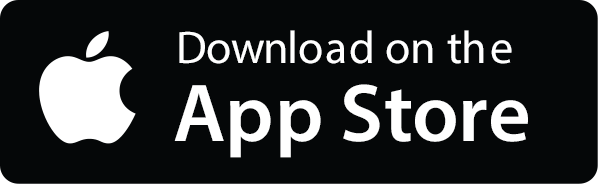
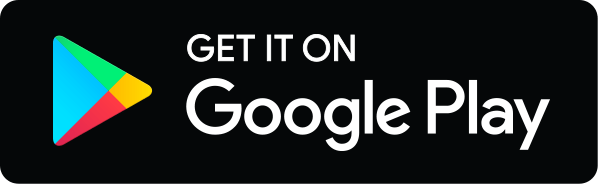