The normal mitral valve annulus is a saddle-shaped structure with 3:4 ratio of the anterior–posterior to commissure–commissure distance.
Mitral stenosis is usually a result of rheumatic fever, and valvular pathology represents antigen cross-reactivity from Group A streptococcus resulting in valvulitis, myocarditis, or pancarditis.
Fibroelastic deficiency and Barlow disease are the predominant forms of degenerative mitral valve disease leading to mitral regurgitation. Fibroelastic deficiency is found in older patients whereas congenitally excessive valve tissue seen in younger patients is observed with Barlow valve.
Chordal rupture is the most common etiology of mitral regurgitation in degenerative mitral valve disease.
Ischemic mitral regurgitation (IMR) is associated with a localized left ventricular infarct with subsequent chamber remodeling and perturbation in the subvalvular apparatus. Functional mitral regurgitation (FMR) is associated with end-stage dilated cardiomyopathy.
Both FMR and IMR are characterized by subvalvular remodeling and annular dilation.
Leaflet remodeling may contribute to the pathophysiology and severity of both IMR and FMR.
Annular dilation and perturbed leaflet coaptation tend to be symmetric in FMR and asymmetric in IMR.
The mitral valve comprises two leaflets with the anterior leaflet being larger and sail-like traversing approximately one-third of the annular circumference. The posterior leaflet is smaller, more rectangular-shaped, and typically has three scallops denoted as P1, P2, and P3 from the anterolateral toward the posteromedial commissure. Corresponding coapting regions of the anterior leaflet are labeled as A1, A2, and A3 according to the Carpentier nomenclature, although no anatomic landmarks, such as distinct interscallop clefts found in the posterior leaflet, separating these regions exist (Fig. 34-1). The free edge of the leaflets is called the bare or membranous zone, with the remainder of the leaflets termed the rough zone. The histologic structure of the leaflets includes the fibrosa, a solid collagenous core that is continuous with the chordae tendineae, and the spongiosa that consists of few collagen fibers but abundant proteoglycans, elastin, and mixed connective tissue cells, which is on the atrial surface and forms the leaflet leading edge. On the atrial aspect of both leaflets, this surface (the atrialis) is rich in elastin whereas the ventricular side (the ventricularis) is much thicker, is confined mostly to the anterior leaflet, and is densely packed with elastin. Nutrients to leaflet tissue are supplied by annular and ascending chordal vessels. The leaflets have long been thought to be passive, inert structures at the hemodynamic mercy of the ebb and flow of ventricular contraction, but recent data refute this view. Leaflet tissue is richly innervated, has intrinsic contractile properties, complex heterogeneous ultrastructure, and may be important in modulating timely and efficient valve closure.1,2
The mitral annulus is a less well-defined structure (particularly the posterior component) without a clear “ring” configuration that anchors the base of the leaflets. It has two major fibrous components, termed the trigones. The right fibrous trigone is part of the central fibrous body of the heart, being in continuity with the aortic valve, the tricuspid valve, and the membranous septum. The portion of the annulus that anchors the anterior mitral leaflet is in direct continuity with portions of the left and noncoronary aortic valve leaflets. The intertrigonal distance defines the fibrous or anterior portion of the mitral annulus and, like the height of the anterior mitral leaflet, is an important clinical marker for surgical annuloplasty sizing as the fibrous annulus has been thought to remain unchanged across the spectrum of mitral disease; however, this region may remodel in ischemic mitral regurgitation (IMR) and functional mitral regurgitation (FMR). The posterior, or lateral, annulus is mostly muscular and manifests most of the dynamic shape and area change of the mitral opening during the cardiac cycle. The normal mitral valve area ranges between 4 and 6 cm2 whereas the normal annular diameter ranges between 5 and 11 cm2 and maintains a 3:4 ratio of the anterior–posterior to the commissure–commissure distance. The annulus has a saddle-shaped configuration with the highest points located anteriorly and posteriorly and the lowest points located at the commissures. This three-dimensional annular structure may be important for proper leaflet stress distribution and effective valve closure. The annulus increases in size during early diastole and decreases rapidly during late diastole and early systole with most of size reduction achieved before ventricular contraction.3 It becomes less planar during systole as the anterior portion of the “saddle” becomes more accentuated simultaneously optimizing mitral valve closure and left ventricular (LV) outflow tract size during the period of ejection. In addition, the mitral annulus descends toward the LV apex during systole; the duration, average rate, and magnitude of annular displacement correlate with the rate of left atrial filling and emptying.
There are two major papillary muscles that support the mitral valve. Both papillary muscles provide chordae tendinae to both mitral leaflets. The anterolateral papillary muscle usually has one major head and is larger; the posteromedial papillary muscle is flatter and can have two or more heads. Vascular supply to the anterolateral papillary muscle is usually from both the left anterior descending and the circumflex arteries, while the posteromedial papillary muscle is often supplied only by the posterior descending artery. First-order chordae, designed to prevent leaflet prolapse, arise near the tips of the papillary muscles and insert into the leading edge of the leaflets while second-order chordae insert farther back into the leaflet, either on the rough zone or at the junction between the rough zone and the clear zone. These chordae are larger and thicker and serve to stabilize the valve, maintain valvular–ventricular continuity, and aid ventricular systolic performance. Third-order chordae arise from ventricular wall trabeculations and insert near the annulus. The chordae also serve as scaffolding for nutrient vessels to supply leaflet tissue while their ultrastructural composition is related to their function.4
Mitral valve stenosis is usually the result of rheumatic fever suffered in childhood or early adulthood. A definite history of rheumatic fever can be obtained in only about 50 to 60 percent of patients; women are affected more often than men by a 2:1 to 3:1 ratio.5 Nearly always acquired before age 20, rheumatic valvular disease becomes clinically evident one to three decades later. Rheumatic disease remains a significant problem throughout the world, although its incidence has fallen sharply in industrialized countries. Rheumatic heart disease is a result of antigen cross-reactivity from group A β-hemolytic Streptococcus culminating in pancarditis, affecting valve leaflets, endocardium, and myocardium to varying degrees. Mimicry between streptococcal antigens and cardiac tissue proteins, combined with high inflammatory cytokine and low interleukin-4 production, leads to the development of autoimmune reactions and cardiac tissue damage.6 Mitral valve involvement is the most common, followed by combined mitral and aortic involvement. Commissural fusion, chordal fusion and shortening, and leaflet fibrosis and retraction as well as significant calcifications are most commonly seen in rheumatic mitral valve disease. Grossly, the mitral opening takes on the classically described “fish mouth” appearance (Fig. 34-2).
Physiologically, mitral stenosis elevates the transvalvular gradient during diastole between the left atrium and the left ventricle, leading to restricted ventricular filling and increased left atrial pressure. Mean valve area of less than 1.0 cm2 and a mean transvalvular gradient greater than 10 mm Hg is considered to be severe mitral stenosis. The transvalvular pressure gradient is a function of the square of the transvalvular flow rate; thus, high transvalvular gradient may be associated with a normal cardiac output, but if cardiac output is low, only a modest transvalvular gradient may be present. In addition, mitral transvalvular flow depends on cardiac output and heart rate. An increase in heart rate decreases the duration of LV filling during diastole and consequently increases the mean transvalvular gradient and left atrial pressure. The dynamic nature of the transvalvular gradient may therefore become significant and hence cause symptoms only with exercise; however, chronic and progressive valvular stenoses are usually accompanied by elevated left atrial pressures and increased pulmonary vascular resistance and pulmonary hypertension. Maintenance of sinus rhythm becomes paramount as atrial contraction augments flow through the stenotic valve, thereby helping to sustain adequate forward cardiac output. However, the dilated atrium becomes substrate for development of atrial fibrillation, which significantly limits ventricular filling. The development of atrial fibrillation decreases cardiac output by 20 percent or more; atrial fibrillation with a rapid ventricular response can lead to acute dyspnea and pulmonary edema. Major determinants of atrial fibrillation in patients with rheumatic heart disease include older age and larger LA diameter.7 The risk of intra-atrial thrombus from stagnant flow within the enlarged chamber increases concurrently.
Isolated mitral stenosis is associated with LV chamber size that is normal or smaller than normal with typically low end-diastolic pressure. Cardiac output is diminished due to underfilling of the ventricle from inflow obstruction rather than LV myocardial dysfunction. During exercise, LV ejection fraction may increase, but this response is blunted by the shorter diastolic filling periods at higher heart rates; therefore, stroke volume and cardiac output do not increase proportionally to physiologic demand. In approximately 25 to 50 percent of patients with severe mitral stenosis, LV systolic dysfunction is present as a consequence of associated problems (e.g., mitral regurgitation, aortic valve disease, ischemic heart disease, rheumatic myocarditis or pancarditis, and myocardial fibrosis), and in these patients, LV end-systolic and end-diastolic volumes may be larger than normal. In severe chronic mitral stenosis with elevated pulmonary vascular resistance, pulmonary arterial pressure is elevated at rest and can approach systemic pressure with exercise. A pulmonary arterial systolic pressure greater than 60 mm Hg significantly increases impedance to right ventricular emptying and produces high right ventricular end-diastolic and right atrial pressures as well as eventual tricuspid insufficiency.8 In time, right ventricular systolic performance deteriorates.
Structural changes of the mitral valve may produce valvular insufficiency by adversely impacting one or more components of the mitral valve complex: mitral annulus, mitral leaflets, chordae tendinae, or LV geometry. Carpentier classified mitral regurgitation into three main pathoanatomic types based on the leaflet motion: normal leaflet motion (type I), leaflet prolapse or excessive motion (type II), and restricted leaflet motion (type III).9 Type III is further subdivided into types IIIa and IIIb based on leaflet restriction during diastole (type IIIa) or during systole (type IIIb) (Fig. 34-3). Mitral regurgitation with normal leaflet motion can be the result of annular dilatation usually due to LV remodeling. Leaflet prolapse typically results from a floppy mitral valve with chordal elongation and/or rupture, but rarely can also be seen in patients with coronary artery disease who have papillary muscle elongation or rupture. Mitral regurgitation due to restricted leaflet motion is associated with rheumatic valve disease (types IIIa and IIIb), ischemic heart disease (IMR with type IIIb restricted systolic leaflet motion secondary to apical tethering or tenting), and dilated cardiomyopathy (type IIIb). This elegant classification system allows for standardized nomenclature while reflecting the underlying pathophysiology of mitral insufficiency across a wide spectrum of structural deformities of the mitral valve complex.
Degenerative mitral valve disease is a major cause of mitral insufficiency requiring surgical evaluation in the United States. Included in this category are myxomatous degeneration, floppy mitral valve, and mitral valve prolapse. The etiology of mitral valve prolapse is both acquired (fibroelastic deficiency in older patients) and congenital or heritable, with excess spongy, weak fibroelastic connective tissue constituting the leaflets and chordae tendineae (Barlow valve, in younger patients) (Fig. 34-4). Mitral valve prolapse is most common in young women and can have a familial component; the more severe forms of insufficiency are more common in men.
In myxomatous disease, the mitral leaflets become thickened and opaque and the degenerative process may extend down the chordae and out onto the annulus. Histologically, elastic fiber and collagen fragmentation and disorganization are present, and acid mucopolysaccharide material accumulates in the leaflets.10 Myxomatous degeneration commonly involves the annulus, resulting in annular thickening and dilatation. All these changes are pronounced in young patients with Barlow valves but can be minimal in older subjects with fibroelastic deficiency, in whom the noninvolved posterior leaflet scallops and anterior leaflet are normal and thin. A minority of patients (5–10 percent) with mitral valve prolapse progress to symptomatic mitral insufficiency; these patients can be placed into three categories: isolated chordal rupture, isolated annular dilatation, and combined chordal rupture and annular dilatation (most common). Chordal rupture can result in acute mitral insufficiency and should be suspected in patients with no previous clinical history of coronary artery disease or acute myocardial infarction. Chordal rupture is typically found in older patients with prolapse due to fibroelastic degeneration without much accompanying leaflet pathology. Posterior chordal rupture, usually subtending just the middle scallop, is the most frequent finding, followed by anterior chordal rupture and then combined anterior and posterior chordal rupture.
FMR is the result of incomplete mitral leaflet coaptation in the setting of LV dysfunction and dilatation with or without annular dilatation (e.g., dilated cardiomyopathy or ischemic cardiomyopathy). IMR, a subset of FMR, is usually associated with a localized LV insult due to myocardial infarction with subsequent remodeling and geometric perturbations in the subvalvular apparatus. Historically, FMR and IMR occur in the setting of what has been thought as structurally normal mitral valve leaflets and are thus considered “functional.” These clinical entities frequently complicate myocardial infarction and carry a poor prognosis in the setting of both coronary disease and idiopathic dilated cardiomyopathy.
“Functional mitral regurgitation” has been used interchangeably to refer to mitral insufficiency due to end-stage ischemic dilated cardiomyopathy and that due to idiopathic dilated cardiomyopathy, yet the mechanisms of mitral regurgitation in these clinical conditions differ significantly.11 The lack of standardized application of “functional” and “ischemic” etiologies of mitral insufficiency represents a considerable hindrance in the appropriate interpretation and comparison of clinical studies, especially since coronary artery disease and LV dysfunction can span a wide range of clinical presentations. Despite these limitations, many investigators today use “functional” to refer to mitral regurgitation in the setting of severe LV dysfunction and dilation usually defined by an ejection fraction of less that 30 percent regardless of etiology.12 IMR, in contrast, refers to patients with coronary artery disease and mitral regurgitation with lesser degree of LV dilation, more preserved ventricular function, and more asymmetric ventricular remodeling usually affecting the posterolateral ventricular wall.13 Not surprisingly, these entities lie on a clinical continuum and cannot always be precisely defined due to the heterogeneity of ischemic heart disease. Animal models of both local and global LV systolic dysfunction and remodeling associated with mitral regurgitation have been developed and permit identification of the contributing geometric factors that result in FMR and IMR in controlled settings.14,15
IMR encompasses a difficult patient population that is characterized by high operative mortality, poor long-term outcomes, and frequent recurrent regurgitation after standard surgical repair.16 Optimal surgical repair and improved clinical outcomes can only be achieved with better knowledge of the pathophysiology of IMR, which is currently incompletely understood. Clinical studies estimate the incidence of postinfarction mitral regurgitation detected on echocardiography to be approximately 20 percent with up to 12 percent of patients having moderate or severe regurgitation.17,18 In patients who progress to clinically symptomatic heart failure, the incidence of mitral regurgitation reaches 56 percent with approximately 16 percent having moderate or severe regurgitation.19 By these estimates, approximately 860,000 patients have clinically significant IMR (moderate-to-severe grade) in the United States with the prevalence bound to increase with the aging population and new therapies to improve postinfarct survival. Echocardiographic studies have shown that mitral regurgitation is an independent predictor of heart failure and death in infarct survivors beyond 30 days. The degree of mitral regurgitation shows a graded relation to 1-year postinfarct mortality, and even mild degree of IMR has been shown to have prognostic significance. Similarly, the presence of mitral regurgitation in dilated cardiomyopathy (or FMR) portends a poor prognosis and is associated with high cardiovascular morbidity and mortality in both ischemic and idiopathic etiologies.
The causative mechanism of IMR appears to lie in the annular and ventricular levels rather than leaflet or chordal structure leading to such labels as “ischemic,” “functional,” and “nonorganic” being clinically applied. Although IMR is a prevailing clinical entity, it has not been consistently defined in the literature adding to considerable confusion and conflicting results. Rankin and colleagues defined IMR as “valvular insufficiency arising from coronary disease without primary leaflet or chordal pathology.”20 Yet this definition appears overly broad as the spectrum of coronary disease and LV dysfunction varies tremendously in IMR ranging from preserved ventricular function to moderate dysfunction to end-stage dilated ischemic and idiopathic cardiomyopathy. A useful definition of IMR was suggested by Replogle who subclassified patients in this group into three entities: (1) sudden-onset mitral regurgitation due to acute myocardial infarction and papillary muscle rupture and/or LV wall dysfunction, (2) mitral regurgitation associated with coronary artery disease with normal mitral leaflets, and (3) mitral regurgitation caused by end-stage ischemic dilated cardiomyopathy.21 The first of these three etiologies usually represents a catastrophic event with very high mortality but fortunately low incidence. Thus, it is the latter two types of IMR that represent the bulk of the clinical disease.
Mitral regurgitation, whether functional or ischemic in origin, is usually associated with LV dilation; however, the degree of chamber remodeling may differ significantly. In a canine model of dilated cardiomyopathy induced with coronary microembolization, Kono and colleagues found increased LV sphericity, or ventricular globularization, to be closely associated with FMR.22 Echocardiographic studies in patients with nonischemic dilated cardiomyopathy confirm LV diameter and sphericity to be major predictors of mitral regurgitation; sphericity has also been shown to accompany progression of mitral regurgitation in end-stage ischemic heart disease and recurrent regurgitation after ventricular reduction surgery.23 IMR, on the other hand, appears not to be solely dependent on global LV dilation but rather on a localized LV insult.24 Nonetheless, recurrent regurgitation after ring annuloplasty for IMR is associated with a persistently spherical ventricle underscoring the spectrum of clinical disease and blurring of boundaries between IMR and FMR as LV dysfunction and dilation progress. Experimentally in an ovine model, a small posterior infarct increases cavity size by 48 percent, yet results in no mitral regurgitation after 8 weeks, whereas large infarcts with lesser degree of LV dilation result in immediate severe regurgitation.25 In a chronic model of ovine posterolateral ischemia, the end-systolic volumes did not differ between animals that did or did not develop significant mitral regurgitation.24
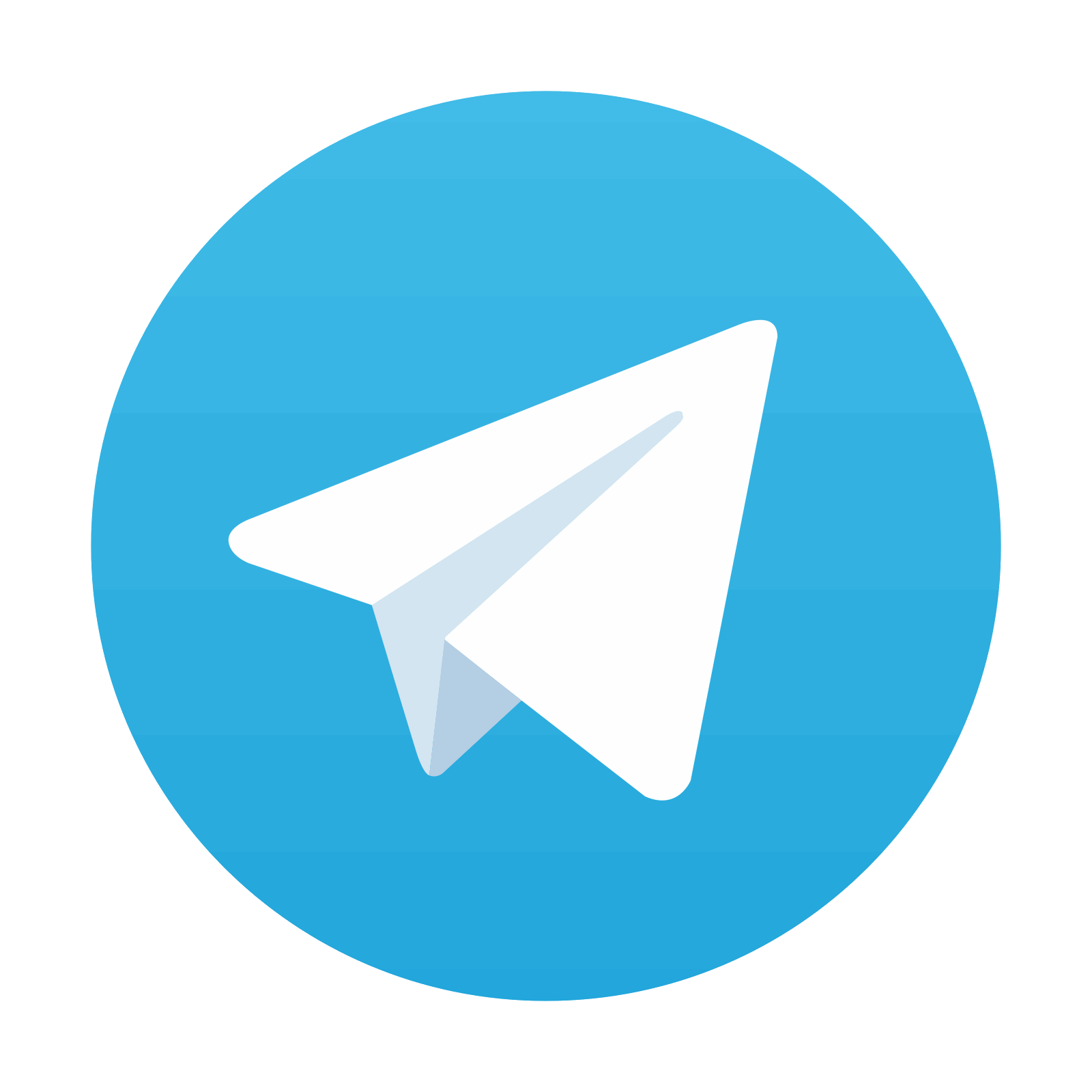
Stay updated, free articles. Join our Telegram channel

Full access? Get Clinical Tree
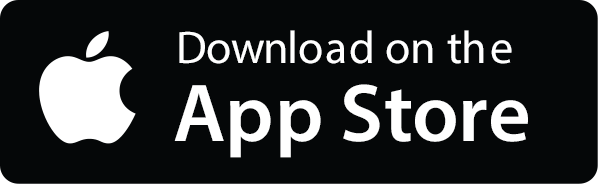
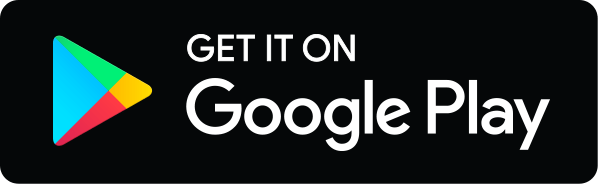