Suzanne Devkota, PhD
The gastrointestinal (GI) tract is a highly complex organ governed by tightly coordinated physiological processes that, at the same time, interact with a dense and diverse microbial population that both relies on us and exists independently of us. This gut microbiome is a critical player in our health from the moment we are born throughout life, and its reach extends far beyond our gut, affecting processes as distant as the brain. However, the direct effect of microbial colonization on gut development and physiology, and on conferring a competent mucosal immune system, has been demonstrated, and now established in the literature. Therefore, a deep understanding of external factors that affect colonization, such as diet, antibiotics, and exposure to pathogens, is of critical importance as microbial-based therapies become mainstream. This chapter provides a practical overview of the microbiome for clinicians.
What Is the Microbiome?
The human body is colonized by bacteria, archaea, fungi, viruses, and protozoa that numerically equal or slightly outnumber our own human cells (Table 2-1). However, on a genomic level, these microorganisms house an order of magnitude more genes than our own. And these genes, minute to minute, day to day, and over their lifespan, interact intimately with our human cells, especially in the gut, where the density of microbiota is the highest. These collections of microbes are called the microbiota, and their genes are called the microbiome. However, today, it is common for the term microbiome to be an all-encompassing term to refer to the body of organisms themselves, and often the bacterial fraction. Additional terms, such as mycobiome and fungome, referring to the fungal fraction, and virome, referring to the viral/phage fraction, are emerging to distinguish the study of these microbial compartments as distinct from the bacterial microbiome, despite the fact that the term microbiome technically refers to all of these microorganisms. However, it is indeed the bacterial component of the microbiome that outnumbers all the other life forms in the human gut, excluding bacteriophage, which are the most abundant but cannot survive independently. Although our understanding of the functions of these individual components continues to grow, what we do know is that the gut microbiome interacts closely with the immune, endocrine, and nervous systems. Therefore, changes in an individual’s gut microbiome composition, that are a departure from their natural homeostasis, are correlated with an array of diseases and disorders, including inflammatory bowel diseases, Parkinson’s disease, and cancer (Practical Pearl 2-1).1–3
Practical Pearl 2-1
What Is Alpha Versus Beta Diversity?
When reviewing microbiome data and analysis, the most common findings reported are alpha and beta diversity, but what does this mean, and how are they measured?
Alpha Diversity
- The average number of different species within a sample.
- Common measure: Shannon diversity index; measures species evenness (eg, similar abundance or do some species dominate?); a higher Shannon diversity index value is more diverse than a lower value.
Beta Diversity
- Measures the differences between 2 samples.
- Common measure: Bray-Curtis dissimilarity; measures difference based on abundance (value between 0 and 1); a value of 0 means that both samples share the exact same species at the same abundance, whereas a value of 1 means that the 2 samples have a completely different species abundance.
Note: Although these are the most common ways to characterize microbiome diversity, newer and complementary methods are being developed.
Illustrative Example
Imagine the circles below are 2 stool samples, and the shapes represent different bacteria species. The conceptual alpha diversity of sample A and B is 3 (3 different species). The conceptual beta diversity of samples A and B is 2, because there are 2 distinct species between them.
Historical Origins of Gut Microbiome Research
The field of the gut microbiome is relatively new. Its emergence as a scientific discipline became mainstream around 2005, when the cost of sequencing microbial genomes was significantly reduced. As data from the studies that followed emerged, discussion of manipulating the gut microbiome as a treatment modality in health care became a widespread part of the conversation, particularly in gastroenterology clinics. However, the microbiome field did not emerge spontaneously. It was a thoughtful evolution over time, rooted in fundamental discoveries in bacteriology and microbiology, the history of which provides a fascinating lens through which to appreciate the challenges and opportunities in modern-day microbiome medicine, particularly as it pertains to fecal microbiota transplantation (FMT).
In the early 1800s, disease was largely attributed to metaphysical factors, such as evil spirits, curses, or punishment for wrongdoings.4 Early observations by Redi, Virchow, and Pasteur of sterile laboratory conditions disproved the theory of spontaneous generation, which was the accepted view at the time and stated that life erupted from nonliving material. Pasteur later discovered that fermentative processes were not caused by air, but rather by the yeast in the air. These observations were the beginnings of the germ theory of disease, which suggested that environmental microorganisms were the source of most diseases.5,6
Two subsequent seminal discoveries bridged the gap between environmental bacteriology and human disease. In 1876, Robert Koch, a German physician, proved that a single bacterium could directly cause a disease. He was asked to investigate why humans and livestock were mysteriously dying across Europe, and he discovered rod-shaped structures in the blood. Through a series of sophisticated experiments isolating these structures from blood and reintroducing them into healthy animals, he determined these structures were living bacteria, which he named Bacillus anthracis—or anthrax.7 The experiments he conducted to identify anthrax led to the series of scientific principles known as Koch’s postulates, which one uses to determine whether a particular bacterium is responsible for disease. These postulates have been slightly modified over time; however, they are still an accepted set of experiments for determining a bacterium’s role in disease.8 Yet, it was not until Theodor Escherich’s 1885 discovery of the now-named Escherichia coli, isolated from the colons of healthy children, that evidence for gut-resident bacteria emerged. He determined that some of the E. coli strains were benign, whereas others were responsible for infant gastroenteritis. This was the first description of native gut microbiota, and also of strain variation of a bacterium in different contexts and individuals—a concept heavily investigated in the microbiome field today.9–11
The nearly 100 years that followed was an era of infectious disease control and vaccine development. These important efforts curtailed diseases that once killed by the thousands. This period was followed by a new era, led by Carl Woese and George Fox, that redefined the tree of life based on genetic signature rather than visual observations of morphology.12 In their landmark paper, arguably one of the most influential microbiology publications to date, Woese and Fox showed that the ribosomal RNA (rRNA) of all living things organized all creatures into 3 categories: archaea, bacteria, and eukarya. The bacterial RNA signature was the 16S rRNA, and subsequent work by Norman Pace, Stanley Falkow, and David Relman applied the discovery of the 16S rRNA to show it could be reliably used to identify difficult-to-grow bacteria among mixed cellular communities13 and that these tools could be applied to the identification of bacteria in humans.14 With this, the genomic revolution of microbiology had begun, spawning the field of the modern-day microbiome (Table 2-2).
Microbiome Colonization in the Gut and Host Defenses
The gut harbors the densest community of microbes in the body, and the density of these microbes varies along the length of the GI tract (Figure 2-1), with the colon harboring the most numerous and diverse population. However, nothing happens by chance in the microbial ecology of the gut. The bacteria that colonize each segment of the GI tract are there because they have the ability to optimally survive in that particular niche amongst the daily chaos we subject them to at each meal, and in turn they serve a specialized function for the host. However, it is always a matter of survival of the fittest bacteria, with external and internal selection pressures creating a continually evolved community. For example, it was previously believed that the high acidity of the stomach prevented bacterial colonization, and therefore the stomach was essentially sterile. However, the stomach actually harbors a diverse, albeit not numerically large, community of commensal bacteria ranging from 10 to 100 bacteria/gram.15 This diversity is dramatically reduced if Helicobacter pylori is present. H. pylori is uniquely adapted to the stomach environment such that it can outcompete all the other organisms and overgrow. It is the same phenomenon that governs Clostridioides difficile infection. When the commensal gut community is suppressed by antibiotics, C. difficile thrives. The colonization resistance that is often conferred by having a robust and diverse microbiome is no longer there, leaving an array of available niches exposed. Once C. difficile takes root and thrives, it can be very difficult to eradicate completely through standard-of-care antibiotics, leading to recurrent infection. This is, in large part, why FMT has proven so effective. To restore a microbially barren colon, a thriving, diverse community of organisms enters the GI tract like a SWAT team, occupying the niches and eradicating the offenders (Figure 2-2).
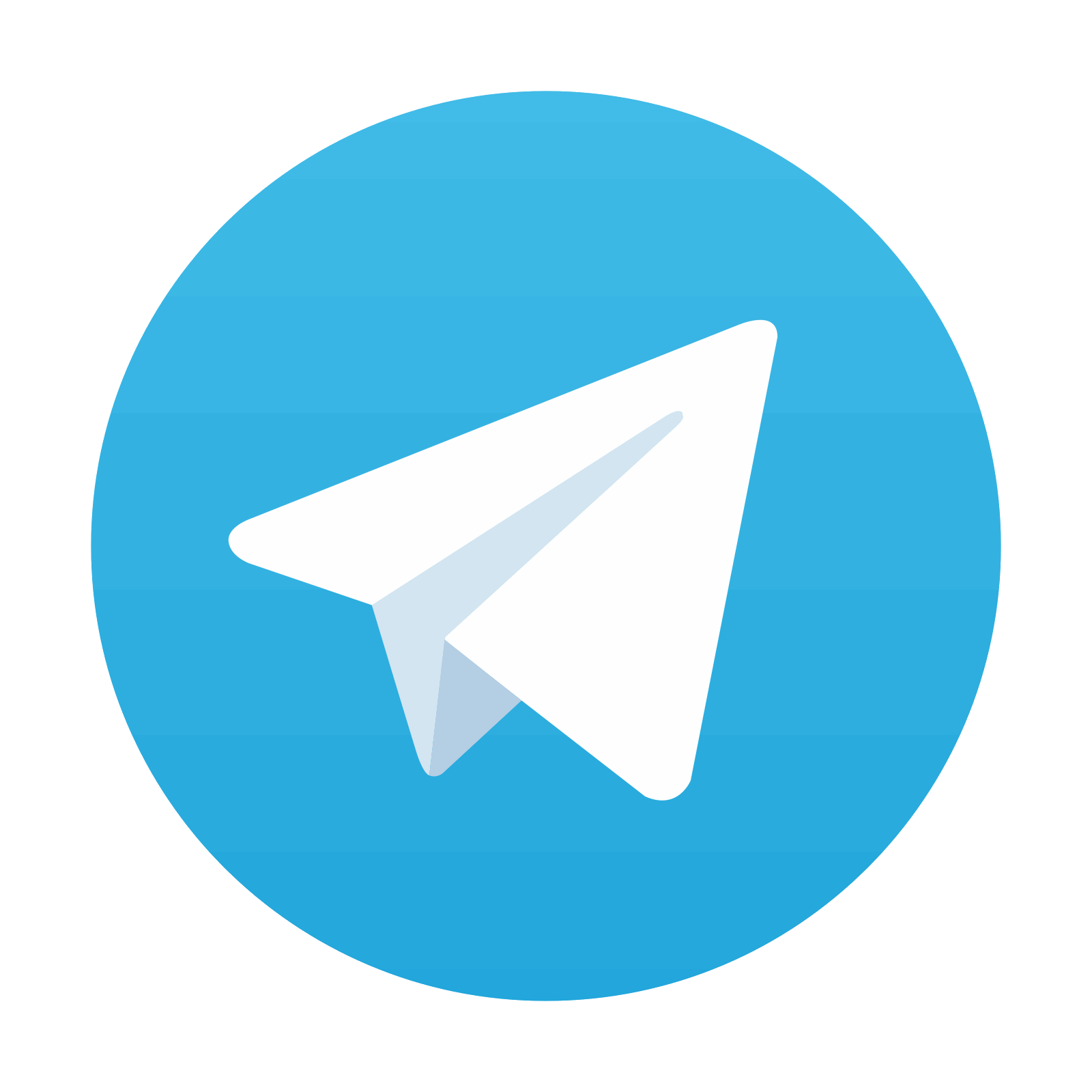
Stay updated, free articles. Join our Telegram channel

Full access? Get Clinical Tree
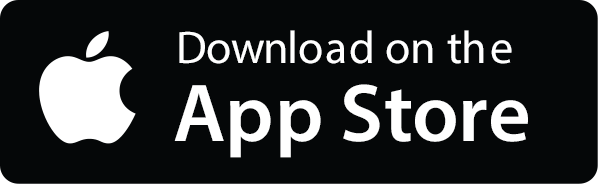
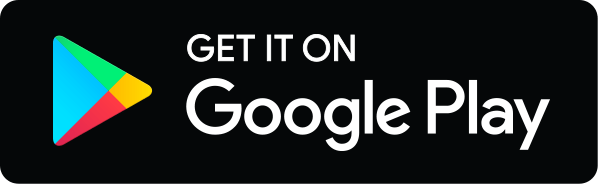