Abstract
Microbes are essential for the health and normal functions of the gastrointestinal tract. However, gut microbes have also been implicated in the pathophysiology of inflammatory bowel diseases (IBD). In this chapter, we discuss the normal microbial colonization of the gastrointestinal tract and the role of microbes in gastrointestinal health. We then address the current evidence and theories for how microbes cause or contribute to IBD, the methods used to determine their roles in these diseases, and the therapies proposed and in use to treat the microbial component of IBD. The goal of this chapter is to provide the context and knowledge needed to understand and evaluate the rapidly accumulating body of research in this area and to identify the opportunities to target microbes to improve diagnosis and treatment of IBD and other complex immune disorders.
Keywords
Bacteria, Colonization, Pathogen, Dysbiosis, Antimicrobial defense, Diagnosis, Therapy, Crohn’s disease, Ulcerative colitis
There is a growing appreciation for the importance of gastrointestinal (GI) microbes in human health and disease. However, which microbes are important and how they contribute to human health and disease are only beginning to be understood. In this chapter, we will discuss how the relationship between host and microbe is established and how a healthy relationship contributes to the health of the GI tract. We will also address current theories for how microbes are involved in inflammatory bowel diseases (IBD) as well as methods to characterize and treat the microbial aspects of disease in IBD patients. Finally, we will identify the emerging opportunities for treatment of IBD in the entire superorganism, human and microbe. Our goal in this chapter is not to provide an exhaustive treatise, but rather to provide the context and knowledge needed to evaluate critically the rapidly accumulating literature, identify unmet needs, and to anticipate future directions in research and discovery relating to the role of gut microbes in IBD.
36.1
The Normal GI Tract Is Composed of Human and Microbial Components
The normal GI tract is a series of organs that perform region-specific functions through cooperative and antagonistic interactions between host cells and microbes. These interactions lead to normal GI tract development, effective and efficient digestion, and defense against nonnative microbes.
36.1.1
Human Component
The human component of the GI tract is composed of tightly attached epithelial cells that line the gut lumen, supporting cells underlying the epithelium, and immune cells resident in the lamina propria. Human, or host, cells are acquired before birth and their physical and functional characteristics are fairly constant throughout life. These characteristics are primarily determined by genetics, which are extremely variable among individuals. The outcome of genetics is influenced by the GI environment, especially the gut microbes in the lumen. Thus, gene and environmental interactions determine host cell gene expression, interactions among host cells, and host cell interactions with microbes.
36.1.2
Microbial Component
In contrast to the human or host component, the microbial component of the GI tract is composed of cells that are more loosely attached to the host epithelium and each other. The microbial community is composed of bacteria, fungi, viruses, archea, and protists. Microbes are primarily acquired after birth and the types of microbes inhabiting the GI tract and their physical and functional characteristics can vary over an individual hosťs lifetime. The trillions of individual microbes in the gut form region- and niche-specific communities that together function as an organ of loosely associated cells. The determinants of microbial community function include microbial genetics, microbe-microbe interactions, host-microbe interactions, and microbial interactions with ingested substances. The colon is the site of the largest and best-characterized microbial population in terms of host-microbe interactions and their role in IBD. Additionally, the majority of microbial studies have focused on bacteria. Therefore, this chapter will primarily focus on bacteria in the colon with the addition of material on other microbes or host sites where it is available.
36.1.3
Organization of Host and Microbial Tissues in the GI Tract
There is a distinct physical separation between host tissues and microbes throughout the GI tract. This barrier serves important functions for both the host and its microbes. First, it limits physical interactions between the host and microbial cells. This prevents direct damage and/or intracellular invasion of intestinal epithelial cells (IEC) by microbes. It also limits microbial access to underlying host tissues. Second, the barrier limits the delivery of microbial components or products to immune sensors in the epithelium to maintain low levels of inflammation and immune tolerance to resident microbes. Third, the barrier protects commensal microbes by minimizing immune targeting and killing of cells that do not express virulence characteristics. Fourth, the barrier allows the host and its microbes to establish relatively stable relationships that prevent incursions of new, potentially pathogenic microbes into the microbial community. Finally, the physical arrangement between the host and microbes allows for stable metabolic interactions between them to provide optimal energy availability for both partners.
Despite the physical separation imposed by the gut antimicrobial barrier, host and microbial cells communicate extensively across the expanse. The barrier is erected and maintained in response to the sensing of microbes in the gut lumen. Likewise, microbes sense and respond to the host components of the barrier. They also make use of them for their own benefit. For example, mucus produced by the host provides attachment sites and carbohydrate sources for microbes. Conversely, the host receives beneficial exports from microbes in the form of metabolites such as short chain fatty acids. Therefore, an intact barrier promotes the health and welfare of both the human and microbial cells in the gut.
The biogeographical organization of the epithelial interface between host and microbes primarily results from acellular physical and chemical defenses produced by the host in response to innate and adaptive immune detection of microbes in the gut lumen. Innate immune mechanisms in IEC and underlying immune cells are activated by structural features of microbes or damage related to microbial attachment or invasion. Toll-like receptors on the cell surface and in endosomes and Nod-like receptors in the cell cytosol are the primary effectors of this detection and their activation leads to inflammation and production of antimicrobial barrier components from the IEC. In the small intestine, IEC produce a thin layer of mucus and copious amounts of antimicrobial peptides near the epithelial surface. Digestive enzymes and bile acids released into the gut lumen also supplement the mucosal defenses to keep the absolute numbers of microbes low relative to the colon. Secretory immunoglobulin A is the primary adaptive immune component of the gut antimicrobial barrier and appears to be primarily produced in the small intestine. It is made by plasma cells of the lamina propria and then translocated into the gut lumen by specialized receptors on IEC. In the colon, the physical space between host and microbe is primarily maintained by cell-associated and secreted mucins. In both the small intestine and colon, epithelial cell turnover also contributes to host defense. While IEC and plasma cells have direct roles in producing the gut antimicrobial barrier, many different cell types feed information regarding the integrity of the barrier to these cells to refine tightly the responses to gut microbes. For example, immune cells in the lamina propria produce cytokines in response to microbial components detected on the host side of the barrier that in turn influence IEC production of barrier components.
Almost all of the data pertaining to the gut antimicrobial barrier has been generated using bacteria as the model microbe. There is some data showing that fungi are also physically separated from the host, although the factors that determine this separation have not been well elucidated. Most of the literature regarding viruses in the GI tract pertains to pathogenic viruses. However, viruses called bacteriophages can infect bacteria and there is a growing appreciation for the role these viruses play in determining survival and behaviors of bacteria in the gut. This suggests that many of the behaviors attributed to individual bacterial species could actually be influenced by or the direct result of bacteriophage infection in those bacteria.
36.2
Microbial Occupation of the GI Tract
Each individual human assembles a unique microbial community in their gut. The determinants of the assemblage include host genetics, environmental contact with microbes able to colonize the gut, dietary resources provided to microbes and host cells, and the niche characteristics available to a given microbe during their transit through the GI tract.
36.2.1
Initial Acquisition of Microbes
The earliest interactions between the GI tract and microbes occur in utero, but the majority of gut microbial colonization occurs after birth. The neonatal GI tract is colonized by vaginal microbes at the time of delivery. These initial microbes are quickly joined by additional microbes derived from the environment to create a more complex community. Over time and with changes in the infant diet from milk to solid foods, the membership undergoes massive upheaval until it resembles the adult microbial community at around 2–5 years of age.
36.2.2
Determinants of Microbial Niche Availability and Occupation
Host and microbial factors interact to determine the nature and availability of macro- and microniches in the GI tract. The host provides a physical space for the microbes to occupy and provides attachment sites and nutrients to the microbes. The mucosal-associated attachment sites consist of the host epithelial tissues themselves, the acellular host proteins and mucins extending from the epithelial surface, and the biofilm formed by microbes that have already colonized the niche. The niche provides macronutrients such as carbohydrates and nitrogen sources along with micronutrients such as oxygen, iron, and salts. The relative concentrations of these nutrients and their availability to incoming microbes is highly dependent on the proximity to the host epithelial surface, host determinants of carbohydrate modification, and the resident microbial community in the niche. This means that the niche encountered by later infiltrating microbes is very different from that encountered by the initial colonizers. It also means that microbes must be able to replicate and recolonize the GI tract, or they will be lost from the community.
The gut lumen is filled with ingested food materials and sloughed host cells that can also be colonized by microbes. The microbes in this niche are thought to be derived from mucosal populations or transiting the GI tract. Material in the gut lumen provides rich sources of biological material for growth and metabolite production by gut microbes and it is likely that the mucosal-associated and luminal populations of microbes influence each other and the host tissues through production of these metabolites. The luminal niche is likely less influenced by host factors and more influenced by diet and other substances ingested by the host.
Both the mucosal-associated and luminal populations of gut microbes are also exposed to host-derived antimicrobial defenses that vary according to the region of the GI tract and determine the nature and availability of microbial niches. As previously discussed, these defenses determine the biogeographical relationship between host and microbial tissues. However, they also determine which microbes colonize the GI tract.
In addition to host factors, microbial factors also influence niche characteristics and the physical availability of space within them. These include structural characteristics of the microbes, metabolic products secreted by microbes, and molecules produced by microbes that influence the behavior or fitness of other microbes. Microbes have both fixed and inducible characteristics, though even relatively fixed characteristics can change to adapt to the environment. The cell surface characteristics of microbes are a product of the type of cell wall that they produce and the physical and chemical properties of that wall. Gram-negative bacteria are covered by a layer of lipopolysaccharide on their surface, Gram-positive bacteria are covered by a thick layer of peptidoglycan, mycobacteria are covered by a thick layer of mycolic acids, and the surface of fungi is coated in mannoproteins. While these surface characteristics are relatively fixed, they can be modified to change interactions with the host or other microbes. For example, modifications to the lipid A component of lipopolysaccharide influence its detection by host TLR4 receptors. Adhesion and motility characteristics are also important determinants of niche occupation. These characteristics are highly influenced by environmental conditions related to the host, other microbes, and diet. Some examples include fimbrae that attach microbes to host tissues or microbial biofilms and flagella that allow them to move into niches or form biofilms. Capsular polysaccharides that modify the microbial cell surface are also inducible and modified depending on environmental conditions. Many of these inducible characteristics are the product of genetic operons that are regulated by invertible genetic elements in the bacterial genome. This allows the entire set of proteins necessary to produce these complex organs to be turned on or off depending on the environmental conditions.
Microbial products can also influence the nature and availability of niches through actions on the host or other microbes. Short chain fatty acids are among the best characterized of the microbial metabolic products. The short chain fatty acids acetate, butyrate, and propionate are produced by bacteria through fiber fermentation. The types and amounts of short chain fatty acids produced by bacteria vary by species and they can be produced by one type of bacteria and utilized by another. Short chain fatty acids also have effects on host tissues that typically promote the health of the IEC. This is an example of a cooperative relationship among microbes and with host tissues, but microbial products can also have direct or indirect effects to exclude other microbes that potentially co-occupy niches. Products with competitive effects include short chain fatty acids produced by commensal bacteria that inhibit the growth of pathogenic bacteria. Likewise, bacteria can produce and deliver antimicrobial agents to competitors in the community through a type VI secretion system. They can also deplete specific substrates necessary for the growth of competitors or secrete waste products that impair the growth of competitors.
The evidence that microbial factors can have a net cooperative effect to encourage colonization by other specific microbes or a net competitive effect that discourages colonization by specific microbes has led to two major concepts related to community organization. The first is the idea that there are keystone microbial species or microbial functions that determine the organizing principles of microbial communities. The second is the idea of pathogenic exclusion. These concepts are the underpinning of the highly embraced theory that healthy microbial communities work to exclude colonization by infiltrating pathogens. Ultimately, community assemblage is the culmination of a huge number of complex interactions among microbes and between microbes and the host. In the end, these interactions establish interconnected, cross-kingdom feedback loops that promote both digestion and defense in the GI tract.
36.3
The Role of Microbes in GI Health and Disease
Colonization of the GI tract by commensal microbes clearly promotes the healthy structure and function of this organ through homeostatic interactions between host and microbial cells. These interactions are necessary for digestion and absorption, prevention of mucosal damage, and promotion of mucosal healing after damage. Emerging evidence also suggests that colonization of the GI tract has important developmental effects on tissues within and outside of the GI tract. In particular, studies from germ-free mice have demonstrated that microbial colonization is essential for development of the normal digestive, absorptive, and propulsive activities of the GI tract. Germ-free mice also have abnormalities of their nervous system, suggesting that microbes influence the development of tissues distant from the GI tract. Finally, the commensal microbial community serves direct and indirect antimicrobial defensive functions in the host through immune system development, induction of host antimicrobial defenses from epithelial tissues, and niche exclusion to prevent colonization by pathogenic microbes.
Though microbes are clearly necessary for the normal functions and health of the GI tract, several lines of evidence have converged on microbes as an important factor in the etiopathophysiology of IBD. The first is the requirement for microbes in murine models of colitis. For example, mice deficient in IL-10 fail to develop colitis under germ-free conditions. The second is the fact that the areas of the GI tract most commonly affected by IBD are the distal ileum and colon, the sites of the highest concentrations of microbes. Third, IBD patients sometimes transiently respond to antibiotics and patients who have undergone colonic resection and ileal pouch-anal anastomosis frequently develop antibiotic-responsive inflammation in the pouch after it is colonized by microbes. Diversion of the fecal stream after ileal resection for Crohn’s disease is also associated with healing in that section of the intestine. Fourth, either single or community-wide changes to gut microbes have been associated with IBD in a large number of studies. These range from studies that have demonstrated increased incidence of single microbes that could function as potential pathogens to those that have shown that the taxonomic composition of the gut microbial community is altered in IBD patients. Fifth, there is evidence that microbes can themselves cause or reinforce phenotypic states of disease. For example, T-bet −/−x RAG2 −/− [TRUC (T-bet −/−x RAG −/− Ulcerative Colitis)] colitis is transmissible by co-housing or cross-fostering. In agreement with this, transferring microbes from mice or patients with colitis to germ-free animal recipients can predispose them to colitis development or lead to development of more severe colitis in naturally occurring or chemically induced models. Finally, a large number of the IBD-associated genetic polymorphisms are in genes involved in microbial sensing and/or responses.
36.4
Methods to Assess Microbial Contributions to IBD
The gut microbial community is made up of trillions of individual microbial cells collectively containing millions of genes. A huge number of different techniques have been developed and applied to this community in attempts to unravel how microbial communities assemble in the gut, which microbial functions are important under homeostatic conditions, and how community structure and function are disrupted in disease. However, there is currently no one technique that can answer all of these questions and data from a number of techniques must be combined to try to assemble models that address these questions.
36.4.1
Microbial Sampling Considerations
The first consideration in assessing the role of gut microbes in disease is which population of microbes is of most interest with respect to the disease process. The majority of human studies have focused on stool microbes since this type of sample is easy to obtain. However, a growing body of literature suggests that mucosal-associated microbes are more relevant to the pathophysiology of IBD. This idea is supported by studies in model organisms showing that the mucosal and luminal microbial communities differ in taxonomic membership, genetics, and functions.
36.4.2
Culture-Based Techniques
Culture-based techniques are the bedrock of microbiology and have provided almost all of the functional data available on gut microbes. The principle behind these techniques is cultivation of one or more microbes in different types of solid or liquid nutrient material under different temperatures and/or gas mixtures. Standard culture conditions have been developed for easily cultivatable and common microbes. However, the precise conditions required to cultivate most microbes in the gut are unknown and some microbes may additionally require specific partners for growth. This means that the majority of gut microbes have never been cultured and are not likely to be amenable to culture.
Most traditional microbiological studies utilize single microbes. This requires isolation of a specific microbe of interest from the microbial community. This microbe is then propagated as a single clone and interrogated in the absence of the contextual clues from the host and the other microbes in the community. Therefore, behaviors demonstrated under these conditions may not faithfully recapitulate in vivo behaviors. Some investigators have attempted to address this limitation by cultivating microbial communities instead. While this may overcome some of the problems associated with studying single cultivars, it presents additional challenges. Many of the microbes in the community do not survive removal from the host, the culture conditions inevitably favor some microbes and disadvantage others, and again the culture conditions skew behaviors in ways that may not mimic in vivo conditions. Despite these challenges and limitations, these types of techniques are still one of the few methods available to perform functional studies with microbes and so they continue to be utilized.
36.4.3
Nucleic Acid-Based Techniques
Nucleic acid-based techniques rely on isolation and sequencing of DNA or RNA recovered from gut microbes. The DNA content of the microbes in the gut lumen is called the microbiome, although this term is often used to more generally to refer to gut microbes. Genomic material can be recovered from live or dead bacteria, which is both a strength and weakness of these techniques. It is a strength because it allows analysis of microbial genetics without requiring the organisms to survive outside of the host, but it also does not discriminate between microbes that are alive and thriving in the gut and those that are not. The DNA must be extracted from the microbes and this presents challenges since many microbes have very tough cell walls while others do not. This means that the amount and quality of DNA recovered from microbes can be influenced by the type of microbe that originally contained it as well as the procedure used to extract it from the cells. It also means that the relative abundance of microbes is a major factor determining whether they will be detected using this type of analysis. It is well accepted that low abundance members of the community are potentially important, but underappreciated using nucleic acid-based techniques.
Nucleic acid-based techniques can be used to detect specific organisms or characterize the entire microbial community. Diagnostic tests for pathogenic microbes typically utilize polymerase chain reaction (PCR) and were initially also applied to IBD. However, the failure to identify specific organisms that are convincingly, consistently, and solely associated with IBD has led to a focus on characterizing the entire gut microbial community in health and disease. There are two major nucleic acid-based techniques currently in use for this purpose. The first is 16S ribosomal RNA (rRNA) for bacteria or 18S rRNA sequencing for fungi. In this technique, PCR primers targeting conserved regions of the ribosomal RNA gene are used to amplify both the conserved region and regions that are highly variable among different microbes. Once the data are generated, bioinformatic analysis is used to assign taxonomy to microbes in the sample. In general, variability in this gene is sufficient to assign taxonomy to microbes at the genus level. However, the actual assignment of taxonomy is somewhat dependent on the initial PCR primer used in sequencing and the software package used for analysis. Each of these can be a source of variability when comparing data between studies. These types of studies generate a taxonomic catalog of the microbes in the sample and their relative abundance. This information has often been used to infer functions based on data reported in the literature for reference organisms interrogated using other, usually culture-based, techniques. However, the validity of these interpretations has to be questioned. It is also common to report community characteristics, such as microbial taxonomic diversity, using this data.
The second nucleic-acid-based technique is shotgun metagenomics. In this technique genetic sequences from many genes, not just ribosomal genes, are obtained from the gut microbes. Short DNA sequences are generated by this sequencing technique, which must be assembled into genes using software. This type of assembly is more complicated than assembly of the rRNA gene and so for the time being, this type of analysis is limited to a relatively small number of scientists with expertise in this area. However, metagenomic analysis is expected to be much more useful than 16S or 18S rRNA sequencing as it will provide data on the actual functional capacity of the microbes in the community. It is well documented that microbes that appear to be the same based on taxonomy can have very different genetic compositions and so have very different functions in vivo. This technique still doesn’t provide direct functional data on gut microbes, but it can be used to determine the functional potential of microbes and polymorphisms in key genes of interest. An additional challenge with this technique is that only a small number of microbial genes are functionally annotated; so, it will need to be combined with other techniques in order to completely understand how the presence or absence of microbial genes contributes to health or disease.
A third nucleic acid-based technique that is not yet in wide use is microbial metatranscriptomics. In this technique, RNA is isolated from gut microbes and sequenced to determine which genes are actually being expressed at any given time in the microbial community. This technique poses substantial technical challenges that must be overcome before it is widely accepted and used. It has been applied primarily to bacteria, which are exquisitely sensitive to changes in the environment and can quickly modify their gene expression to adapt to those changes. In order to accomplish these rapid changes in gene expression, bacterial RNA is highly unstable and has an extremely short half-life of only minutes. This makes it difficult to capture gene expression under the conditions of interest and likely limits this technique, at least for the time being, to use under conditions where bacteria can be rapidly recovered and their RNA quickly isolated. It also means that gene expression is highly context-dependent and that the type of sample used for analysis is very important. For example, studies of single microbes or small communities in model organisms have shown that microbial gene expression in mucosal-associated microbes is very different from that of the same microbes in stool. Another major problem with metagenomics is that there is a huge amount of mammalian host RNA and microbial structural RNA (tRNA and rRNA) in addition to the microbial mRNA of interest in most samples. This means that the starting biomass of the sample has to be substantial and that it must be processed to remove the contaminating RNA species or amplification bias will prevent analysis of the microbial mRNA.
36.4.4
In Situ Imaging Techniques
Another important piece of information about the microbial community is where the microbes are physically located relative to each other and to host tissues. The microbial community is not completely uniform along the gut, even within major anatomic divisions of the tract. In situ imaging techniques provide a way to visualize and analyze individual niches and the biogeography of these niches. Native bacteria can be labeled by using a DNA sequence complementary to a gene of interest [fluorescence in situ hybridization (FISH)] or an antibody targeting a protein of interest. These techniques allow identification of specific microbes or microbial features in fixed samples of GI tissue (immunohistochemistry or immunofluorescence). The probe (DNA or antibody) is attached to the target in conjunction with a fluorescent marker that can then be visualized with fluorescent microscopy. This technique can provide crucial data regarding the location and relative abundance of certain microbes, expression of specific microbial or host genes, or expression of specific microbial or host proteins. However, it also presents some challenges. The fixation process is important to these studies as preservation has the potential to distort in vivo relationships. Also, the information that can be gleaned through these techniques is limited by the ability to generate specific, effective probes. Microbes can also be imaged in situ through fluorescent, bioluminescent, or click chemistry labeling of the bacteria themselves followed by association with a model host organism. In these approaches, the tissues can be fixed or imaged in an unfixed state.
36.4.5
ex vivo Microbe Interrogation Techniques
The techniques described for in situ imaging of bacteria can also be adapted for the ex vivo characterization of microbes. In these techniques, microbes are removed from the host and interrogated without cultivation. Bacterial DNA, RNA, or protein can be labeled as described above and then the bacteria can be imaged using flow cytometry and related techniques. This allows high throughput quantitative or semiquantitative data regarding these parameters, although spatial information regarding relationships between bacteria or with host tissues is not gathered. Additionally, microbial behaviors can be characterized using immunoblots or ELISAs for protein expression or functional assays. These types of approaches allow for collection of more functional data on microbes. However, as previously discussed, removing the microbes from the host can impact their functions, even over short time spans.
36.4.6
Microbial Product Assessment
Microbes secrete a huge number of products in vivo. Some of these products are byproducts of metabolism, some appear designed to promote co-occurrence of partner microbes, some appear to inhibit growth of other microbes, and some have direct effects on host tissues. Characterizing these products is absolutely key to understanding the role of microbes in gut health and disease, but it has proven to be particularly challenging. The first challenge is that production of metabolites is particularly sensitive to the context of the microbes. Therefore, metabolites produced by cultured microbes are likely to be very different from those produced in vivo. Second, many of these metabolites are volatile compounds that require sophisticated techniques and expensive equipment to interrogate. Third, a relatively small number of metabolites are well-characterized, though it is presumed that a very large number of metabolites are produced in vivo. So, any mechanistic data available about microbial products is limited to a small number of products while a large focus of this area of research is the identification of novel microbial products.
36.4.7
Investigating Microbial Functions Using Model Systems
Model systems provide a way to capture some of the context of in vivo microbial conditions. The most common models are in vitro cell cultures and germ-free animals. Cell cultures can involve a single eukaryotic cell type or combinations of cell types from the same species. The conditions can also be varied according to the questions an investigator wishes to pursue. The cells used in these studies have traditionally been cells recovered from human neoplasms, human fetal cells, or human or mouse cells immortalized through viral infection or transformation with genes that allow unrestricted growth. However, the scientific community is increasingly moving toward use of primary cells from humans and mice. Using in vitro cell culture models typically involves isolation and cultivation of single microbes from the gut. The microbes, products, or components derived from microbes can then be used to treat the mammalian cells. If intact microbes are used, the impact of the mammalian cells on the microbes and microbes on the mammalian cells can be analyzed. Products or components are used when only the impact of the microbes on mammalian cells is of interest. It is also possible to use more complex mixtures of microbes or products derived from more complex mixtures, but their use is more difficult in this type of model system.
Germ-free animal models provide an opportunity to study complex microbial communities in vivo. Single organisms or complex communities can be transferred to animals raised under germ-free conditions and then studied. The range of potential germ-free model animals includes zebrafish, mice, rats, rabbits, and pigs with additional models in development. Since many of these models are amenable to genetic manipulation, this type of model also allows investigators to study the impact of microbes on host physiology and disease. Human microbes and microbial communities are often transferred to germ-free animals. This provides a unique opportunity to generate mechanistic data about these microbes, but it comes with limitations that must be considered when interpreting data from these studies. Microbes must survive removal from a host and colonize the new host. This can lead to loss of some community members or changes in relative abundance in the new host. This is an especially important consideration when transferring microbes from one species to another. Many studies have shown that the human microbiome is uniquely human and that transferring microbes across species lines leads to microbial community remodeling toward a community that is more reflective of the recipient than the donor. This is likely due to unique host factors that determine engraftment and survival of the transferred microbes. It also suggests that the functions and products of microbes in this new environment may not fully recapitulate those of the original human host.
36.5
Theories About How Microbes Cause or Contribute to IBD
Microbes appear to be a key component of IBD pathophysiology. However, how they cause or contribute to IBD is a matter of intense debate and may be unique to a given individual. No single causative organism, microbial community characteristic of disease, or consistent microbial biomarker of disease has been identified in IBD. This is likely due to the incredible variability of microbial community composition among individuals and the fact that microbial behavior is highly context-dependent. So, the same microbes are not present in all humans and even when the same two microbes are present, their behavior may differ depending on interactions with other microbes in the gut community, interactions with host tissues, and environmental influences like diet. Thus, it is not clear whether the presence, absence, abundance, or behavior of a single or set of microbes is the strongest determinant of disease and it may vary among individual patients. This has led to the development of a number of theories for how microbes and bacteria in particular could cause or contribute to IBD. Each of these theories is based on evidence derived from IBD patients, though no one theory appears to explain disease in all patients. Furthermore, the same evidence can be used to support different theories, suggesting that they are not mutually exclusive and patients could have a number of different microbial mechanisms contributing to their disease.
36.5.1
True Pathogen
The earliest efforts to understand the role of gut microbes in IBD focused on identification of a causal pathogenic organism. This was primarily due to the ingrained ideas of bacterial infection and disease pathogenesis that were originally developed in the 1800s by Robert Koch. One of the first microbes proposed as a potential pathogen in IBD was Mycobacterium avium subspecies paratuberculosis (MAP). This bacterium is an obligate intracellular organism that causes Johne’s disease in cattle and other ruminants. In cattle, MAP causes chronic granulomatis colitis that shares phenotypic and histologic features with Crohn’s disease in humans. This organism is incredibly difficult to culture due to its obligate intracellular nature and extremely slow growth. It can be identified in tissue with a Ziehl-Nielsen stain, but only if it is producing a cell wall and cell wall-deficient forms (spheroplast) may predominate in human tissues. These features have led to reliance on PCR for MAP DNA or RNA as a diagnostic test. The IS900 PCR is generally considered the most reliable, although it is subject to the same biological and technical strengths and weaknesses as other PCR assays. These are the inability to differentiate between active infection and detection of contaminating or noninfecting DNA/RNA and the reliance on efficient and effective nucleic acid extraction from samples.
An extremely large number of studies have attempted to determine whether MAP is a causative agent in IBD using various combinations of these diagnostic tests on blood or intestinal tissue. In support of MAP as a potential pathogen in IBD, many studies have demonstrated MAP DNA by PCR in the blood of patients with Crohn’s disease and a smaller number have demonstrated MAP DNA in the blood of ulcerative colitis patients. Fewer studies have detected evidence of MAP in intestinal tissues by culture, staining, or PCR. Conversely, many studies have found evidence of MAP in healthy controls or failed to find evidence of MAP in cohorts of patients with disease. In fact, some studies have found a higher incidence of MAP DNA in healthy patients than in controls.
The pathogenic role of MAP has also been investigated using mouse models of colitis. In most cases, MAP does not efficiently colonize a healthy monogastric gut, but it will colonize immunocompromised mice or at very high doses. It has been shown to increase weight loss and delay healing in mice treated with dextran sodium sulfate (DSS) to induce colitis. This suggests that MAP has the potential to colonize the intestine and worsen disease in nonruminants, but that the healthy gut is resistant to colonization. Understanding whether MAP causes or contributes to IBD has been further complicated by the fact that most patients who have been studied are not treatment naive and many of the drugs used as standard therapy for IBD have efficacy against MAP in vitro . However, studies attempting to discern whether antibiotics targeting MAP are beneficial in IBD have been somewhat mixed. This includes a large, prospective, placebo-controlled, double-blind, randomized trial of anti-MAP drug therapy that did not find a sustained benefit of these drugs in Crohn’s disease. However, some researchers have argued that this finding may reflect the fact that the placebo arm of the study received standard therapy. The most convincing argument against MAP as a causative agent in IBD is the beneficial effect of anti-TNF therapy in this disease. Anti-TNF therapy is associated with reactivation of latent tuberculosis, but is often quite effective in Crohn’s disease. Examined together, these factors have led to a general consensus in the field that while there is evidence linking MAP to IBD, the causal relationship is still unclear.
The second bacterium that is frequently discussed as a potential pathogen in IBD is adherent invasive Escherichia coli (AIEC). These are a specific type of E. coli that adhere to and invade epithelial tissues and macrophages. They are more prevalent in ileal Crohn’s disease than in controls, but are only rarely found in colonic tissue from Crohn’s disease patients or ulcerative colitis patients. However, overall increases in adherent E. coli have been reported in both Crohn’s disease and ulcerative colitis. Likewise, E. coli similar to AIEC have been implicated in the granulomatous colitis of boxer dogs.
AIEC have been detected in patients using a number of different techniques and have also been isolated and cultivated for interrogation in mechanistic studies. These mechanistic studies have shown that AIEC are more adherent and lead to increased TNFα production versus commensal E. coli in cell models. AIEC do not readily colonize the normal intestine of wild-type mice. However, DSS treatment leads to increased colonization and persistence of AIEC in the colon. This colonization then leads to more inflammation and tissue damage than DSS alone or colonization with commensal E. coli. The Darfeuille-Michaud group has also demonstrated that the human-specific protein CEACAM6 is increased at the surface of the ileal epithelium of Crohn’s disease patients and mediates attachment of AIEC to the epithelium. Mice transgenic for a group of human CEACAM proteins, including CEACAM6, are efficiently colonized by AIEC and subsequently develop spontaneous colitis. Thus, there are patient-related and mechanistic data supporting a role for AIED in Crohn’s disease of the terminal ileum. However, it is not clear whether this role is dependent on patient-specific factors or if it is broadly applicable in all patients with this disease.
Very few studies have attempted to simultaneously study more than one potential pathogen in IBD patients. However, one study looked at MAP and AIEC in IBD patients. In this study, approximately 40% of patients with Crohn’s disease had evidence of both MAP and AIEC. This suggests that these microbes may be associated with the underlying disease process, rather than acting as specific causative organisms.
Though MAP and AIEC are the best established of the potential pathogens in IBD, a number of other bacteria have been proposed for this same role. These include enterotoxigenic Bacteroides fragilis , Clostridium difficile , Listeria monocytogenes , Chlamydia sp., Salmonella typhi , and Campylobacter jejuni. Several of these bacteria are agents of acute gastroenteritis, suggesting that transient GI infection may act as a trigger for IBD. This idea is supported by studies that showed increased risk for developing IBD after nonspecific infectious gastroenteritis or infection with Salmonella or Campylobacter.
36.5.2
Dysbiosis
Dysbiosis is often defined as an “imbalance” in the gut microbial community that is associated with disease. This imbalance could be due to the gain or loss of community members or changes in relative abundance of microbes. This theory primarily focuses on changes in the taxonomic make-up of the microbial community and functions associated with individual members or subsets of members in the community. Characterizing dysbiosis has traditionally relied on taxonomic catalogs of gut microbes generated in different individuals at single time points using 16S or 18S rRNA sequencing. More recent efforts have attempted to catalog not the microbial species, but rather the microbial genes in the gut using shotgun metagenomics. The taxonomic characterization or genetic make-up of the community is then used to infer its functions based on data in the literature from studies using reference microbial strains. Usually, these model microbes are studied as single organisms, and often in vitro in order to generate these functional data.
A huge number of studies have identified significant differences in the taxonomic composition of gut microbial communities between IBD patients and healthy controls. These differences have been identified in the bacterial, fungal, and viral components of the community. However, the specific microbial taxonomic differences vary widely by study and a definitive disease-associated community structure has not been identified. This may be due to the large variation in gut microbial community composition among humans and/or to technical differences among the studies. Therefore, how to define dysbiosis and diagnose a “dysbiotic community” is still unclear. It is also not clear whether dysbiosis is a cause, effect, or both a cause and effect of disease as inflammation can itself lead to changes in microbial community composition in the gut.
Dysbiosis could cause or contribute to IBD in a number of different ways. It could lead to gain of one or more microbes with functions detrimental to the host or loss of one or more microbes with functions beneficial to the host. Since many of the microbes in the gut community have important functional relationships with each other, changes in a small number of microbes and/or their functions could have broad impacts on the community. Specific functions that are likely to be of interest include changes in niche availability or characteristics that affect colonization acceptance or resistance, changes in microbial products or physical characteristics that change interactions among bacteria or with the host, and changes in the community that activate virulence mechanisms in otherwise commensal microbes. The most consistent characteristic of microbial communities from diseased patients is a loss of taxonomic diversity. However, it is again unclear whether loss of biodiversity in the gut is the cause or effect of disease. It is also currently ambiguous how such a loss of diversity alone could lead to disease, especially since gnotobiotic animals colonized with one or a small number of microbes do not show disease related to colonization with a limited community alone. This suggests that the specific microbes in the community and their functions are of the utmost importance.
Microbial community composition and behavior are both exquisitely sensitive to the environment. The environment of the gut microbial community consists of both host-derived components and ingested substances. Each of these factors can have profound impacts on the composition and behavior of the gut microbial community. The host-derived components are determined by host genetics and potentially by the effects of diet or other ingested substances directly on the host. The best-characterized host determinants of microbial community composition and behavior are the host antimicrobial defense systems. When these systems are disrupted by genetic knockout of individual components in model animals, dysbiosis ensues. The ingested contents of the gut lumen are primarily derived from the diet. The contribution of the diet to microbial dysbiosis is of particular interest to many investigators as IBD is spreading in tandem with the spread of Western lifestyles across the globe and the Western diet is very different from traditional diets in non-Westernized societies. Dietary components consist of macronutrients such as fats and sugars, micronutrients such as vitamins and minerals, and nonnutrient components such as dietary emulsifiers. The Western diet is typically highly processed and contains large amounts of saturated fats and simple sugars. This type of diet has been shown to promote production of metabolites that are harmful to host tissues. Conversely, diets low in substrates that microbes need to produce metabolites that promote health in host tissues can also contribute to disease. For example, nondigestible dietary fiber is used by microbes to produce short chain fatty acids and dietary tryptophan is used to produce aryl hydrocarbon receptor ligands, both of which promote intestinal health. Lastly, dietary emulsifiers are often added to processed foods to improve their texture or stability. In vitro, these compounds activate the production of fimbrae in E. coli , which leads to adherence and tissue damage by these normally commensal bacteria. In vivo, consumption of dietary emulsifiers have been shown to cause dysbiosis and exacerbate colitis in IL-10 −/− mice. Other types of environmental stress, such as antibiotic administration, can also lead to changes in the microbial community through direct microbial killing or horizontal gene transfer. Environmental stress can also determine whether bacteriophages engage in a lysogenic or lytic lifestyle. Phage-induced lysis not only kills bacteria, it also potentially leads to infection of new bacterial targets with changes to how those bacteria behave.
An open question in the field of dysbiosis is the importance of when the dysbiotic changes in the microbial community occur. Cesarean births lead to alterations in early colonization events and early antibiotic administration can significantly disrupt developing communities. Both of these factors have been linked to a number of immune-mediated diseases later in life, but definitive proof of causation or insight into mechanisms is still lacking. Additionally, many patients develop IBD without early life events that would be expected to cause dysbiosis. So additional unknown events may contribute to early, long-standing dysbiosis at the time of IBD diagnosis or more acute dysbiotic events may also contribute to IBD. The type of dysbiotic event is also expected to be a key factor in how dysbiosis contributes to IBD. Whether these are broad changes to the community or small, pivotal changes in particular microbes remains to be determined. This uncertainty is at least partially due to technical challenges that may overestimate the relevance of highly abundant microbes and underestimate the functional importance of less abundant members of the community. Technical limitations of currently available techniques and data are also likely to overestimate the important of known microbes with established functional data over unknown microbes that may be just as important for disease. Finally, how dysbiosis causes or contributes to IBD is still unknown. There is some evidence that specific dysbiotic microbial communities can cause disease in normal hosts. However, there is much more convincing evidence that dysbiosis works in conjunction with additional factors such as underlying genetic predispositions or intestinal damage to cause disease. For example, transfer of microbial communities from IBD patients to germ-free mice does not appear to cause spontaneous colitis. However, it does appear to contribute to disease in mouse models that are predisposed to disease, such as IL-10 −/− mice or with chemical induction of colitis. Additionally, dysbiosis associated with Western-type diets seems to allow colonization with IBD-associated organisms. Germ-free mice colonized by microbes from mice fed a high-fat, high-sugar diet have an increased susceptibility to colonization by AIEC. Therefore, dysbiosis may have different impacts on different hosts depending on the nature of the dysbiotic community and underlying genetic predispositions for disease in the host. Regardless of whether dysbiosis arises first and causes disease or whether it is an outcome of disease, once it arises, it almost certainly contributes to the perpetuation of disease.
36.5.3
Failure of Gut Antimicrobial Defenses
Host antimicrobial defenses determine which microbes colonize the gut and where that colonization occurs. They also promote host and microbial functions necessary for peaceful coexistence between the microbial community and the mammalian host. These antimicrobial defenses take the form of innate immune mechanisms that are primarily deployed by epithelial cells lining the GI tract and adaptive immune mechanisms that are activated when the integrity of the gut antimicrobial barrier is compromised. They include agents of digestion such as digestive enzymes and bile acids that are detrimental to bacteria. They also include more specific defenses such as secretory IgA and antimicrobial peptides. The importance of these host antimicrobial defense mechanisms to the proper assemblage and functions of the gut microbial community has been demonstrated in an enormous number of studies using animal models genetically deficient in these components. Mice that are globally or conditionally deficient in antimicrobial defense genes are predisposed to spontaneous colitis or develop worse colitis in response to intestinal damage. For example, mice deficient in the primary secreted colonic mucin, Muc2, develop spontaneous colitis. This model is also supported by the fact that the majority of IBD-associated genetic polymorphisms occur in microbial sensing or response genes. Finally, changes in host antimicrobial defense systems also lead to dysbiosis, which can then contribute to disease pathophysiology through the previously discussed mechanisms.
36.5.4
Inappropriate Immune Responses to Normal Gut Commensal Bacteria
Inappropriate immune responses to normal gut commensal bacteria could result from changes in how the innate and adaptive immune systems of the gut are exposed to commensal bacteria or how they react once they are exposed to microbes. Though either the innate or adaptive immune systems or both could mount aberrant responses to gut microbes, this theory mainly focuses on adaptive immunity. Patients with IBD have increased levels of pro-inflammatory cytokines that are typically produced by T cells in response to microbes. They also have increased levels of antibodies reactive against gut microbial antigens derived from yeast, E. coli , and bacterial flagellin.
Aberrant immune responses could be triggered by increased immune cell exposure to microbes. This can happen through changes to microbes that increase their expression of antigens or changes to the host and/or microbes that lead to invasion across the barrier into normally protected host tissues. Aberrant immune responses could also result from a failure of immune tolerance toward microbes that are normally resident in the gut. The immune system is constantly exposed to gut commensal microbes and immune counter-regulatory mechanisms limit the responses to these microbes under homeostatic conditions. When these counter-regulatory mechanisms fail, an inappropriate immune response is activated against gut microbes. Normally commensal microbes could also change their surface antigens, potentially through phase-inducible virulence mechanisms leading to activation of immunity directed against these novel antigens. Lastly, immunoreactivity against gut commensals could be accidentally generated during appropriate responses to gut pathogens. This is a particular danger with antigenic elements that are shared between commensals and pathogens.
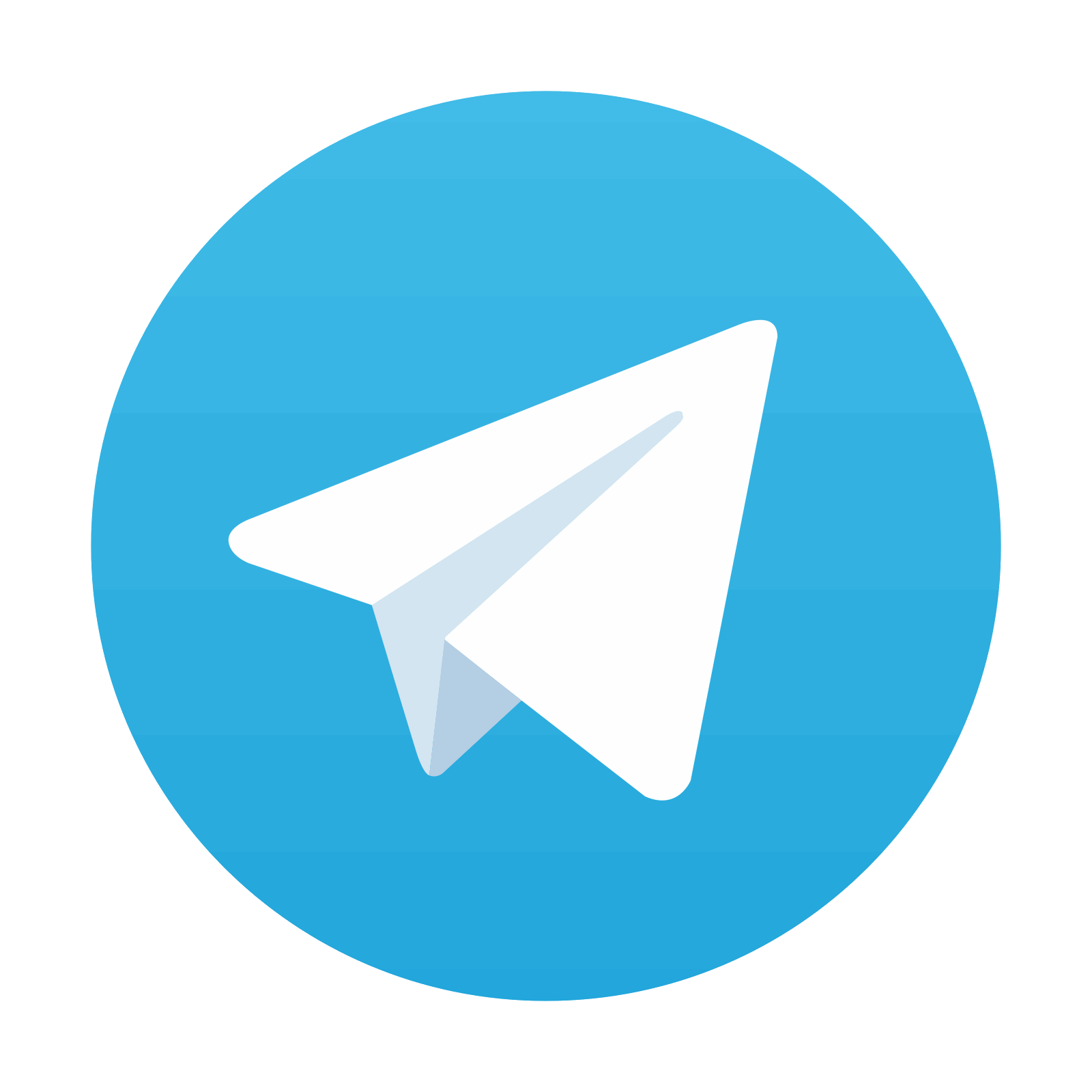
Stay updated, free articles. Join our Telegram channel

Full access? Get Clinical Tree
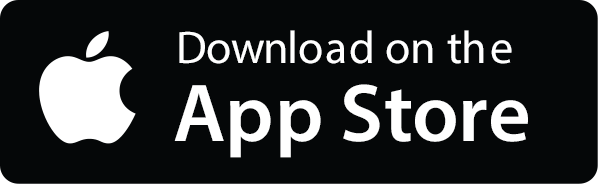
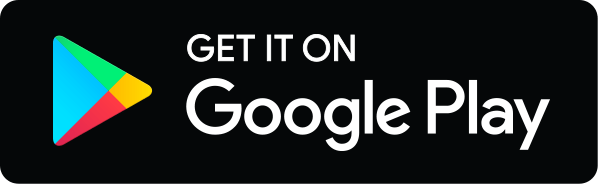