(5)
Division of Nephrology, Department of Medicine, National University Hospital, Singapore, Singapore
(6)
Department of Intensive Care, Austin Hospital, 145 Studley Road, Level 2 Austin Tower, Heidelberg, VIC, 3084, Australia
16.1.1 Summary
Acidosis is common in patients with AKI in the ICU and often associated with acidemia. It is typically secondary to the accumulation of lactate, chloride and unmeasured anions. Its correction appears desirable and can be more reliably and safely achieved with CRRT. Use of bicarbonate-based fluids is safest as the initial approach. However, lactate- and citrate-buffered fluids can also correct acidosis if appropriately metabolized by the liver and other key organs. CRRT can also be used to correct extreme acidosis in the absence of a major degree of renal impairment. As CRRT controls volume status easily, it would additionally enable bicarbonate infusion to occur for more rapid correction of acidemia.
In patients with acute kidney injury (AKI), metabolic acidosis is especially common [1]. Although the exact mechanisms of metabolic acidosis in AKI are complex, excess of retained metabolic acids is likely to contribute, together with other general acid–base disorders of critical illness (hyperlactatemia and/or hyperchloremia) [2]. Depending on its severity, correction may require different levels on intervention including renal replacement therapy (RRT) [3].
Both intermittent hemodialysis (IHD) and continuous RRT (CRRT) correct metabolic acidosis. However, the rate and degree of correction differ significantly. CRRT normalizes metabolic acidosis more rapidly and more effectively in the first 24 h. Moreover, IHD is associated with a higher incidence of metabolic acidosis than CRRT during the subsequent treatment period. Accordingly, CRRT is physiologically superior in terms of correction of metabolic acidosis [4]. The plasma concentration of solutes available for ultrafiltration, the composition of the dialysis or replacement fluid, and the rate of ultrafiltration all appear to determine the effect of RRT on acid–base status.
Once CRRT is commenced, even acidemia is typically corrected within 24 h. CRRT appears to correct metabolic acidosis in acute renal failure through its effect on unmeasured anions, phosphate and chloride. Once CRRT is established, it becomes the dominant force in controlling metabolic acid–base status and, in stable patients, it typically results in a degree of metabolic alkalosis [5].
During CRRT, according to conventional acid–base thinking, there is a substantial loss of “buffers.” Lactate and bicarbonate have been used as “buffers” during RRT [6, 7]. Citrate has been used as “buffer” and anticoagulation. These “buffers” affect acid–base balance. Bicarbonate has the major advantage of being the physiological buffer and is now the dominant or sole buffer used in CRRT in developed countries. Moreover, lactate, if not metabolized and still present in blood, acts as a strong anion, which would have the same acidifying effect of chloride. Accordingly, iatrogenic hyperlactatemia can cause a metabolic acidosis. The administration of lactate-buffered fluids can induce significant hyperlactatemia and acidosis in patients with liver failure because the metabolic rate is insufficient to meet the additional lactate load.
Citrate has been used for regional anticoagulation. During this procedure, citrate is administered to the circuit before the filter and chelates calcium, thus impeding coagulation. Once citrate enters the circulation, it is metabolized to CO2 and then bicarbonate on a 1:3 basis; thus, 1 mmol of citrate yields 3 mmol of CO2 and then bicarbonate. Under these circumstances, citrate acts as the “buffer” as well as the anticoagulant [8, 9]. Its administration can lead to metabolic alkalosis (up to 25 % of cases). Attention must be paid in patients with liver disease who may not be able to metabolize citrate. In these patients, citrate may accumulate and result in severe ionized hypocalcemia and metabolic acidosis because the citrate anion acts as an unmeasured anion and increases the SIG, which has acidifying effects.
When oxidizable anions are used in the replacement fluids, the anion must be completely oxidized to CO2 and H2O in order to generate bicarbonate. If the metabolic conversion of non-bicarbonate anions proceeds without accumulation, their buffering capacity is equal to that of bicarbonate. Thus, under most circumstances, the effect on acid–base status depends on the “buffer” concentration rather than on the kind of “buffer” used. The nature and extent of acid–base changes during CRRT is therefore governed by the intensity of plasma water exchange/dialysis, by the “buffer” content of the replacement fluid/dialysate and by the metabolic rate for these anions.
CRRT can be also used to correct severe metabolic acidosis even in the absence of severe acute kidney injury. If the dose of treatment is titrated to achieve such a goal, essentially even the most dramatic metabolic acidosis can be corrected. In addition, because volume can be controlled, if deemed necessary, a bicarbonate infusion can be started at 100–200 mmol/h and the volume removed simultaneously by CRRT. Such an approach can expedite the correction of even the most severe level of acidemia.
Key Messages
Acidosis and/or acidemia are common in critically ill patients with AKI.
The acidosis of AKI in ICU is complex and involves the accumulation of lactate, chloride and unmeasured anions.
Correction of acidosis appears desirable and can be achieved with renal replacement therapy.
CRRT is superior to IHD in correcting metabolic acidosis in critically ill patients with AKI.
Bicarbonate is the safest buffer to achieve correction of acidosis, but citrate- and lactate-based fluids can also achieve such correction, provided they are correctly and rapidly metabolized.
16.2 Electrolyte Management During CRRT
(7)
Service d’Anesthésie-Réanimation 2, Centre Hospitalier Universitaire de Bordeaux, Bordeaux, France
(8)
Department of Intensive Care, VU University Medical Center, Amsterdam, The Netherlands
16.2.1 Summary
Electrolyte balance of CRRT depends on baseline plasma concentrations, the composition of the dialysate or replacement fluids, CRRT dose and type of anticoagulation. Hypokalemia and hypophosphatemia are the most faced electrolyte disturbances during RRT. During citrate CRRT, calcium balance depends on the target plasma ionized calcium concentration of the protocol, but is often negative. Some algorithms provide a zero calcium balance option. During heparin CRRT, calcium balance is generally slightly positive. Magnesium balance is generally negative, more so during citrate than during other anticoagulation, due to the low magnesium concentration of commercial fluids. During CRRT, plasma concentrations of potassium, phosphate, calcium and magnesium should be monitored. Choose replacement fluids with concentrations close to target plasma concentrations or supplement these electrolytes separately.
16.2.2 Introduction
Continuous renal replacement therapy (CRRT) is used to support renal function but also to recover homeostasis; electrolyte management is actually a challenge in that case. The electrolyte balance of CRRT depends on baseline plasma concentrations, the composition of the dialysate or replacement fluids, CRRT dose and type of anticoagulation.
16.2.3 Potassium and Phosphate
The most common electrolyte disorders observed during CRRT are hypokalemia and hypophosphatemia [10–12]. Due to frequent hyperkalemia in patients with acute kidney injury before start of CRRT, solutions with a low potassium concentration (0–2 mmol/L) are developed. Then, hyperkalemia is rapidly normalized by CRRT. However, after some hours of treatment, hypokalemia occurs. This is more so for phosphate, because most replacement fluids are phosphate-free. Hypophosphatemia can occur early during hemofiltration treatment, especially with higher CRRT dose. Although few disturbances are registered in some studies (in particular with pediatrics patients [11, 13], other electrolytes are correctly regulated, because concentrations in the substitution fluids are close to normal [14, 15].
16.2.4 Role of Effluent Volume
There are clearly some differences between the different modalities of RRT treatment in terms of electrolytes removal and acid–base equilibrium. The diffusion principle used in the dialysis mode is more effective for small molecule removal (as electrolytes) than hemofiltration at standard dose (25 ml/kg/h). However, the convection principle used in the hemofiltration mode can remove much more electrolytes when used at high volume (more than 35 ml/kg/h) [16]. However, the most important parameter for electrolyte removal remains the dose of renal replacement (effluent volume). Electrolyte deficiencies are more frequent when high effluent volumes are used [17]. In two large randomized controlled trials comparing low- and high-intensity RRT, more electrolyte imbalances were found in the high-intensity group [2, 3].
16.2.5 Intermittent Versus Continuous
Furthermore, continuous techniques are more able to normalize hyperkalemia or hyperphosphatemia than intermittent hemodialysis, but are also more likely to induce electrolyte deficits like hypophosphatemia [9]. Accordingly, CVVH achieves better acid–base balance and electrolyte removal than extended daily dialysis, but can also induce severe hypophosphatemia [18].
16.2.6 Calcium
The typical calcium concentration of the bicarbonate- and lactate-buffered fluids is about 1.75 mmol/L, substantially higher than the plasma ionized calcium concentration, which is the amount accessible for filtration or dialysis. Thus, calcium balance during conventional CRRT is generally slightly positive (about 5–12 mmol/day) [19, 20]. However, during citrate CRRT, calcium handling is different and calcium balance of many protocols is negative [19, 20]. Most fluids used for citrate CRRT are calcium-free and calcium is generally replaced by separate infusion. For calcium replacement, each protocol has its own ionized plasma calcium target, ranging between 1.0 and 1.35 mmol/L. Especially with lower plasma calcium targets, calcium balance is negative, 12–96 mmol/day is reported [19, 20]. Of note, a negative calcium balance does not necessarily cause systemic hypocalcemia, due to a rapid parathormone response, which leads to unwanted calcium release from bone [21]. Targeting a higher plasma calcium concentration seems to suppress PTH release, which may limit calcium mobilization from bone. However, PTH measurements in critically ill patients should be interpreted with caution, because the normal assay measures oxidized PTH as well, which is biologically inactive [22]. Some modern CRRT devices incorporate an algorithm with a 100 % calcium compensation option, which seems attractive. Up to now, optimal calcium targets are not known. Hypocalcemia is common in patients with sepsis, and it is not known whether correction is beneficial [23]. However, a prolonged negative calcium balance for days should likely be avoided.
16.2.7 Magnesium
Magnesium balance during CRRT has gained little attention. The typical magnesium concentration of commercial CRRT fluids is about 0.5 mmol/L, while high normal or increased magnesium concentrations are targeted in ICU patients to prevent arrhythmias, promote vasodilatation and anti-inflammatory and anti-oxidant defense [24]. When using these fluids, mean magnesium balance during CVVHDF was −17 mmol/day in heparin circuits and −26 mmol/day in circuits anticoagulated with citrate [25]. Apart from calcium, citrate chelates magnesium, explaining the higher magnesium loss during citrate CRRT in this study. Hypomagnesemia is reported by others as well [26] and is even associated with non-recovery of renal function [27]. Given the important role of magnesium during critical illness, additional magnesium should be supplemented, the amount depending on the fluids in use, the local CRRT protocol and the plasma magnesium concentration.
16.2.8 Monitoring of Electrolytes During CRRT
Standard electrolyte monitoring should include potassium (two to four times daily), phosphate and magnesium (twice daily), total calcium (once daily) and ionized calcium (four times daily during citrate anticoagulation). See also the chapter on citrate anticoagulation.
Key Messages
Electrolyte balance of CRRT depends on baseline plasma concentrations, the composition of the dialysate or replacement fluids, CRRT dose and type of anticoagulation.
Hypokalemia and hypophosphatemia are the most faced electrolyte disturbances during RRT.
During citrate CRRT, calcium balance depends on the target plasma ionized calcium concentration of the protocol, but is often negative. Some algorithms provide a zero calcium balance option.
During heparin CRRT, calcium balance is generally slightly positive.
Magnesium balance is generally negative, more so during citrate than during other anticoagulation, due to the low magnesium concentration of commercial fluids.
Choose replacement fluids with concentrations close to target plasma concentrations or supplement these electrolytes separately.
16.3 Nutrition and Micronutrients
(9)
Division of Nephrology, Department of Medicine, National University Hospital, Singapore, Singapore
(10)
Department of Intensive Care, Austin Hospital, 145 Studley Road, Level 2 Austin Tower, Heidelberg, VIC, 3084, Australia
16.3.1 Summary
In patients receiving renal replacement therapy, enteral nutrition is preferred. Specific so-called renal feeding preparations are not needed. The dose of protein should be between 1 and 1.5 g/kg/day. Energy provision should likely be between 20 and 25 kcal/kg/day. Amino acid loss through the filter accounts for 10–20 % of administered protein. Water soluble vitamins are lost and should be replaced. Lipid soluble vitamins are not lost and do not require regular replacement. Some trace elements are lost and regular trace element administration seems prudent. However, all recommendations regarding nutrition in these patients are based on weak evidence.
16.3.2 General Recommendations
The preferred mode of nutritional support in critical illness is early enteral nutrition (EN) [28, 29]. In the absence of more definite evidence, CRRT patients should receive EN within 24–48 h following admission titrated to gut tolerance. EN is preferred to parenteral nutrition (PN) because of its safety, low cost, and its physiological route and hormonal signalling. The role of supplemental PN in CRRT patients is unknown.
The target goal of nutrition remains ill defined. Providing 20–35 kcal/kg/day of calories with 1.5–1.8 g/kg/day of protein in patients with AKI on CRRT appears to allow a reasonable balance between protein catabolism and nitrogen balance [30]. This empirical caloric amount seems consistent with calculated requirements [31]. Due to water soluble vitamin losses, it seems prudent to administer water soluble vitamins daily or second daily. As some trace elements are also lost, it seems prudent to administer trace elements daily or second daily.
These recommendations for CRRT patients are based on clinical observational studies, small studies with physiological outcomes and physiological reasoning. They are low-level recommendations (level C) and open to challenge. Such lack of evidence suggests the need for level 1 studies in this field.
16.3.3 Protein
The optimal protein intake is unclear, and the flexibility of adjusting intake is limited by the composition in standard enteral formulas. For example, Isosource® 1.5 cal (Nestle) contains 1.5 kcal/ml of calories and 67.6 g of protein per litre. Intake of 30 kcal/kg/day only delivers 1.4 g/kg/day of protein. Higher protein intake of 2.5 g/kg/day results in a near positive or positive nitrogen balance and offers the only active measure to reduce the negative nitrogen balance so commonly seen in these patients [32, 33]. A positive nitrogen balance is associated with improved patient survival in critical illness, but improved survival is not a direct effect of increased protein intake [31]. However, there may be a dose-related association between increased protein intake and clinically significant improvement in renal function in critically ill patients [34]. Supplemental PN does not lead to improved clinical outcomes [35] and, if given early, may lead to clinical problems [36]. There is no evidence that specific administration of essential AA in preference to general AA preparations leads to any improved clinical outcomes.
16.3.4 Amino Acids (AA)
The molecular weights of proteins range from 55 to 220 kDa, well above the cut-off of standard CRRT membranes, while that of AA is only 110 Da (75–204 Da). AA loss during CRRT has been studied mostly in the context of total parenteral nutrition (PN).
Most AA have a high sieving coefficient (easily filtered) of near 1.0, such as cysteine, arginine, alanine and glutamine, except for glutamic acid (0.25–0.5) [37–39]. AA clearances range from 20 to 45 ml/min in patients on CRRT [40], as compared to about 200 ml/min in patients with AKI on intermittent hemodialysis [41]. In general AA loss represents about 11–12 % of total (parenteral) protein intake. The amount of AA lost in each patient depends on the intensity of CRRT but also in great part on AA blood levels, which in turn depend on the amount administered and route of administration.
Protein intake of more than 1.5 g/kg/day is associated with AA loss of about 12 g/day [39], and AA loss seems less with lower protein intake [41]. AA losses can account for 5 % to a substantial 20 % of daily AA intake [37–39, 41]. Individual blood AA levels correlate well to corresponding losses in CRRT [41], and overall AA loss is higher with higher intensity of treatment [37]. In addition, there is selectivity in individual AA losses, with glutamine and alanine being lost in greater absolute amounts [39, 41], and tyrosine having the highest fractional loss per intake [37]. All three are non-essential AA under normal physiological states.
Total nitrogen loss in CRRT is about 25 g/day [38, 39]—the vast majority of which is due to urea nitrogen with 10–20 % (2–5 g/day) contributed by AA nitrogen loss [37–39]—while the rest is likely from protein catabolism; this results in a negative nitrogen balance. Intermittent hemodialysis has been reported to lead to losses of 6–8 g/session [17].
< div class='tao-gold-member'>
Only gold members can continue reading. Log In or Register a > to continue
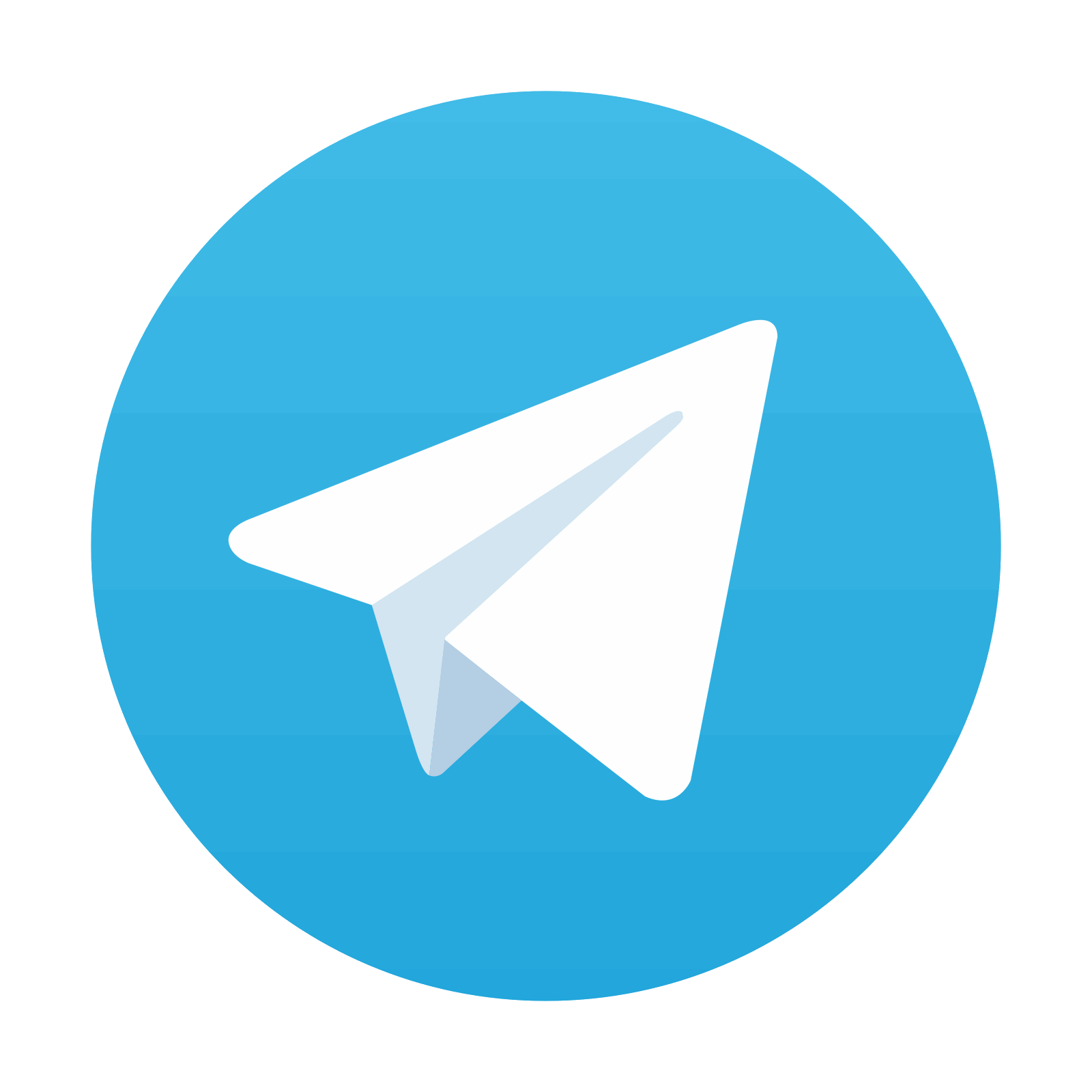
Stay updated, free articles. Join our Telegram channel

Full access? Get Clinical Tree
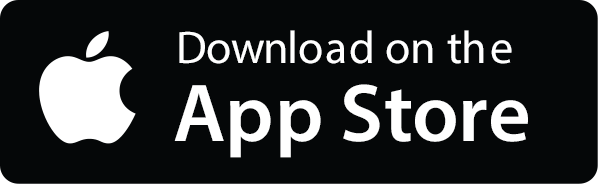
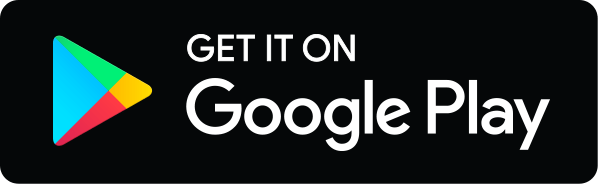