Abstract
Helicobacter pylori induces a persistent inflammatory response within the human stomach, and chronic gastritis is the strongest known risk factor for peptic ulceration and distal gastric cancer. Further, H. pylori colonizes over half of the world’s population. However, only a fraction of persons harboring this organism ever develop clinically apparent disease. H. pylori isolates exhibit remarkable genetic diversity, which engenders differential host inflammatory responses that influence clinical outcome. H. pylori strains that contain the cag pathogenicity island and secrete a functional cytotoxin induce more severe gastric injury and further augment the risk for developing ulcer disease and cancer of the stomach. However, pathologic sequelae are not completely dependent upon bacterial virulence factors, and clinical disease is also influenced by host genetic diversity, particularly within immune response genes, as well as dietary factors. Understanding the mechanisms that regulate H. pylori -induced gastritis is critical, not only to develop more effective treatments for diseases associated with this organism but also because such knowledge may serve as a paradigm for the role of chronic inflammation in the genesis of other pathologic lesions that arise within the gastrointestinal tract.
Keywords
H. pylori , Inflammation, Gastritis, Pathogenesis, Virulence, Gastric disease
63.1
Introduction
Helicobacter pylori is a Gram-negative bacterial species that selectively colonizes gastric mucosa, and virtually all persons colonized by this organism develop coexisting gastritis. A signature feature of the inflammatory response to H. pylori is its capacity to persist for decades, which is in marked contrast to inflammatory reactions induced by other Gram-negative enteric pathogens, such as Salmonella , that either resolve within days or progress to eliminate the host. H. pylori has evolved numerous strategies to facilitate its persistence including limiting the bactericidal effects of pro-inflammatory molecules, varying the antigenic repertoire of surface-exposed proteins, and/or actively suppressing the host-adaptive immune response. However, microbial persistence implies linkage in which signals of the colonizing organism affect signals of the host and during its coevolution with humans, H. pylori has developed the ability to send and receive signals from gastric epithelium, allowing both host and bacteria to participate in a dynamic relationship.
There are biological costs incurred by the long-term relationship between H. pylori and humans in that chronic inflammation confers a significantly increased risk of serious disease, including peptic ulceration (gastric and/or duodenal), gastric adenocarcinoma, and non-Hodgkins lymphoma of the stomach. On the basis of epidemiological studies in humans and experimental studies in rodents, the World Health Organization has designated H. pylori a class I carcinogen for gastric cancer, and since virtually all infected persons have superficial gastritis, it is virtually certain that the organism plays a causative role early in this progression ( Fig. 63.1 ). Eradication of H. pylori significantly decreases the risk of developing gastric adenocarcinoma in colonized individuals, providing additional evidence that this organism influences early stages in gastric carcinogenesis. However, although persistent H. pylori infection is the strongest identified risk factor for peptic ulcer disease or malignancies that arise within the stomach, only a small percentage of colonized persons ever develop clinical sequelae, suggesting that disease risk involves specific and well-choreographed interactions between pathogen and host, which, in turn, are dependent upon strain-specific bacterial factors and/or inflammatory responses governed by host genetic diversity. Further, dietary factors such as high salt intake and low iron states can intensify H. pylori -induced injury. Recent evidence has also indicated that H. pylori -induced chronic gastritis, particularly when localized to the acid-secreting corpus, is associated with a reduced risk for developing gastroesophageal reflux disease, Barrett’s esophagus, Barrett’s-associated esophageal adenocarcinoma, and asthma and atopic diseases. Thus, a comprehensive understanding of how H. pylori initiates ulcer disease or gastric cancer necessitates understanding how H. pylori induces gastritis, and this chapter will focus on specific mechanisms through which H. pylori colonization leads to gastric inflammation and injury via manipulation of the host immune response.

63.1.1
Colonization of the Gastric Mucosa
To successfully colonize the human stomach, H. pylori must initially overcome the barriers of gastric acidity and peristalsis. H. pylori possesses several mechanisms to elude these primary host defenses and establish persistent infection within the gastric niche ( Table 63.1 ). Acute ingestion of H. pylori by humans leads to transient hypochlorhydria, but when left untreated, microbial-dependent inhibition of acid secretion resolves within several months and intraluminal pH decreases to within the normal range. In some individuals, acid production continues to increase, which is likely to result from a compensatory rise in serum gastrin and fall in mucosal somatostatin levels induced by gastric inflammation.
Bacterial Product | Functions | Targeted Host Defense |
---|---|---|
Urease | Neutralize acidity/regulate inflammation | Gastric acid |
Flagellae | Motility/evade TLR5 | Gastric peristalsis/immune response |
Adhesins | Adherence to gastric epithelium | Gastric peristalsis |
Lewis antigens | Adherence to gastric epithelium/molecular mimicry | Host immune response |
Arginase | Decrease nitric oxide | Inflammation |
AhpC, NapA | Antioxidants | Oxidative stress |
LPS | Evade TLR4 | Immune response |
DNA | TLR9 signaling | Immune response |
VacA | Suppresses T-cell activation | Immune response |
CagY | Antigenic variation | Immune recognition |
One pH-altering mechanism within the H. pylori arsenal is urease, a nickel-containing hexadimer composed of two different subunits (60 and 27 kD) ( Table 63.1 ). Urease enzymatic activity, which converts urea to ammonia and carbon dioxide, is conserved among all known Helicobacter species and allows survival to pH as low as 1 in the presence of urea. Further, the primary structure of urease shows little divergence among H. pylori strains suggesting that urease is a necessary factor for the establishment of chronic infection. Indeed, several studies in mice have demonstrated that the activity of H. pylori urease is absolutely required for both successful initial colonization and maintenance of infection. In addition, infection of rhesus macaques with H. pylori producing low levels of urease activity results in expansion of a subpopulation expressing high levels of urease activity.
Seven genes comprise the H. pylori urease gene cluster: ureA and ureB encode the structural subunits of urease, while ureE , ureF , ureG , and ureH encode accessory proteins necessary for assembly and Ni 2 + insertion required to form active urease. Urease activity increases 10- to 20-fold as the pH falls from 6.0 to 5.0 and remains constant thereafter down to a pH of 2.5. Acid activation of cytoplasmic urease is mediated by expression of the third gene in the urease gene cluster, ureI , which encodes a H + -gated urea channel. UreI increases the permeability of the bacterial membrane to urea by at least 300-fold as the pH of the surrounding medium becomes acidic, and the presence of this acid-activated urea channel within the H. pylori inner membrane is necessary for efficient utilization of urea present in gastric juice. UreA/B forms a membrane complex with UreI, and assembly of the urease apoenzyme at the membrane surface facilitates access of urea to urease within the cytoplasm, which permits rapid neutralization of the periplasmic space. These data explain the requirement for both urease and UreI for survival of H. pylori at a medium pH of < 4.0 as well as for successful colonization of animal models. In addition to altering the local pH, urease has a role in the disruption of the tight junctions of gastric epithelial cells. A potential novel function for urease has been identified using H. pylori isogenic deletion mutants and recombinant proteins in H. pylori : macrophage coculture systems. These studies demonstrated that upregulation of inducible nitric oxide synthase and release of nitric oxide, a pro-inflammatory molecule, were dependent upon ureA expression, raising the hypothesis that urease may not only be required for ammonia production but may also regulate inflammation.
H. pylori can further alter the gastric pH by increasing host production of the pro-inflammatory cytokines. One such cytokine is interleukin-1β (IL-1β), which has significant acid-suppressive properties. Studies with H. pylori -infected Mongolian gerbils demonstrated that levels of gastric mucosal IL-1β increase after 6–12 weeks of infection. This increase corresponds to a reciprocal decrease in gastric acid output. Demonstration that administration of recombinant IL-1 receptor agonist normalizes acid output, implicates IL-1β as a key modulator of acid secretion. Host polymorphisms associated with increased expression of IL-1β have been linked with increased risk for atrophic gastritis and gastric adenocarcinoma. High-expression alleles of another pro-inflammatory cytokine with acid-suppressive properties, TNF-α, are also associated with an increased risk for gastric cancer.
To facilitate movement within gastric mucus and to counteract peristalsis, H. pylori possesses multiple unipolar flagella that are comprised of two major structural subunits: a 53-kD FlaA and a 54-kD FlaB. The genes encoding these two flagellins are located at distant sites on the H. pylori chromosome and are transcriptionally regulated by different promoters. Expression of flaB and a gene encoding a component of the flagellar basal body export apparatus ( flhA ) peaks early during bacterial growth phase and precedes expression of flaA. Flagellin-dependent motility is also regulated at a posttranscriptional level by an H. pylori bicistronic operon comprised of neuA , encoding a cytidyl monophosphate- N -acetylneuraminic acid synthetase, and flmD , which encodes a putative glycosyl transferase gene. Inactivation of flmD results in a motility-defective H. pylori mutant that is incapable of glycosylating FlaA.
The essential role for both flaA and flaB in the establishment of persistent colonization has been confirmed by demonstrating that aflagellate H. pylori strains only transiently colonized gnotobiotic piglets. In addition, studies in mice found that not only are the flagellar structures required for chronic colonization, but motility as well. The data further suggest that full motility requires urea such that the pH is increased thereby decreasing the mucin viscoscity. Ultrastructural studies have demonstrated that the H. pylori flagellum is cloaked by a sheath that is an extension of the bacterial outer membrane, which likely protects the acid-labile flagellar structure from gastric contents. Similar to urease production, motility is required for persistent infection, and FlbA, a component of the flagellar secretion apparatus that regulates flagellar biosynthesis, also mediates urease activity ; thus, FlbA may couple urease production and motility, bacterial phenotypes that are necessary for establishment and maintenance of gastric colonization.
In addition to motility, chemotaxis plays an important role in the ability of H. pylori to establish residence within the stomach. H. pylori expresses four chemotaxis receptors to sense external stimuli: TlpA, TlpB, TlpC, and TlpD. TlpA is a receptor for arginine, sodium bicarbonate, and senses acid gradients, TlpB may be involved in pH taxis, TlpC is responsive to media with nickel or zinc, and TlpD senses both acidic as well as basic pH. Unlike FlaA and FlaB, loss of individual Tlp proteins does not prevent colonization in wild-type-infected animals although levels of colonization may be lower. In addition, mutations in tlpB result in significantly less inflammation in infected C57BL/6 mice and gerbils. However, H. pylori that lack expression of TlpA and TlpD are unable to sense acid, resulting in a 100-fold decrease in colonization. Interestingly, when acid production in the stomach is blocked with omeprazole, the ability of tlpAD mutants to colonize is restored. In addition, the coupling proteins CheV1 and CheW, kinase CheY, and response-regulator Y are also critical for chemotaxis.
Though the vast majority of H. pylori in colonized hosts are free-living within the gastric mucous layer, ~ 20% bind to gastric epithelial cells. Adherence is important for colonization likely due to the need for acquisition of nutrients from the host and for resistance to shedding of the mucus gel layer. Adhesion by H. pylori to gastric epithelium is highly specific in vivo and when H. pylori is found in the duodenum, it only overlays islands of gastric metaplasia and not intestinal-type cells. H. pylori expresses greater than 30 outer membrane proteins (OMPs), including the Hop family of proteins, that includes several known and predicted adhesins to facilitate epithelial cell binding. One of these proteins is BabA that binds the host histo-blood group carbohydrate Lewis b and is associated with increased production of IL-8. Bugaytsova et al. have demonstrated that BabA binding is pH sensitive and has a role in H. pylori adaptation to changes in the gastric environment during disease progression and/or decreased adherence to cells shed into the gastric lumen. BabA adaptation to pH occurs via mutation and recombination events with related genes in such a way that changes in adherence are fully reversible.
The host receptor sialyl-LewisX is induced and bound by the H. pylori OMP SabA. The gene-encoding SabA can be in an “on” or “off” configuration, which is regulated by slipped strand mispairing within a group of CT dinucleotide repeats in coding region and a homopolymeric T tract in the promoter region of the gene and transcription of sabA is part of an acid responsive regulon. Recently, the adhesin HopQ has been shown to bind host CEACAM molecules, glycoproteins that regulate numerous cell functions, including adhesion. Additional OMPs with adhesin properties present in most, if not all, H. pylori strains include HopZ, AlpA, and AlpB. All of these H. pylori adhesins have roles in colonization and adherence to the gastric epithelium.
The O-antigen of H. pylori lipopolysaccharide (LPS) contains human Lewis antigens, including Lewis x , Lewis y , Lewis a , and Lewis b . Inactivation of the bacterial genes encoding Lewis x and Lewis y results in an inability of H. pylori to colonize mice, suggesting that H. pylori Lewis antigens may also mediate adhesion ( Table 63.1 ). In vitro, preincubation of H. pylori with anti-Lewis x monoclonal antibodies inhibits bacterial binding to gastric epithelial cells. H. pylori strains that strongly express Lewis x/y are associated with enhanced neutrophilic infiltration and higher colonization densities than strains that only weakly express Lewis x/y Since H. pylori Lewis antigens undergo phase variation (i.e., random and reversible high-frequency alteration of phenotype), a functional role for such changes in Lewis phenotype may be detachment of H. pylori from host epithelial cells that would subsequently facilitate transfer to a new host.
Studies that have focused on adherence of H. pylori to gastric epithelium have also provided mechanistic insights through which this organism colonizes different parts of the stomach. For example, Syder et al. studied bacterial tropism in transgenic mice deficient in acid-secreting parietal cells. Mice were colonized for 8 weeks with an H. pylori strain that expresses adhesins recognized by epithelial NeuAcα2,3Galβ1,4 glycan receptors. In control mice, H. pylori exhibited tropism for gastric mucosa that did not contain parietal cells (e.g., the boundary between the squamous epithelium and the proximal glandular epithelium), and lymphocytic infiltration was found preferentially in this area. In mice having a genetic ablation of parietal cells, epithelial progenitor cells synthesized NeuAcα2,3Galβ1,4 glycans, which was accompanied by an expansion of bacterial colonization and lymphoid aggregates within the glandular epithelium. Histopathologic studies in infected human subjects initially noted that H. pylori binds to particular areas within the gastric epithelium, and that bacterial density is greatest in the upper portion of the gastric glands. This pattern of colonization mirrors the distribution of trefoil factor 1 (TFF1), and Clyne et al. demonstrated that H. pylori binds avidly to TFF1 dimers, suggesting that TFF1 may act as a receptor for this organism in vivo. In contrast, a specific component of gastric mucin (α1,4-linked N -acetylglucosamine O -glycan) that is confined to the deeper glandular regions has been shown to exert antimicrobial effects against H. pylori in vitro by inhibiting the biosynthesis of cholesteryl- d -glucopyranoside, a major H. pylori cell wall component. However, recent studies using 3D reconstructions of confocal in vivo microscopy images have identified microcolonies of replicating H. pylori within the progenitor and stem cell compartments of both human and mouse stomachs. These findings indicate that the host has evolved mechanisms to restrict H. pylori to certain regions of the gastric mucosa.
63.1.2
Evasion of the Host Immune Response by H. pylori
If a bacterial species is to persistently colonize a mammalian host, its most formidable challenge is evasion of immune clearance. One mechanism through which H. pylori may persist is by limiting the bactericidal effects of pro-inflammatory molecules, such as nitric oxide (NO). Inducible NO synthase (iNOS, NOS2) has been shown to be upregulated by H. pylori in macrophages in vitro as well as in vivo . Further, macrophages can kill H. pylori in coculture in an NO-dependent manner even when there is no contact between the macrophages and bacteria. However, H. pylori expresses a functional arginase, RocF , which effectively siphons l -arginine away from the competing host enzyme, iNOS. This, in turn, limits the production of NO by limiting the availability of the l -arginine substrate. H. pylori further limits NO production by inducing expression of the host enzyme ARG2, which also uses l -Arg as its substrate.
H. pylori may also encounter reactive oxygen or nitrogen species generated by activated neutrophils that can induce oxidative DNA damage, which can not only harm the bacteria but also injure host tissue. H. pylori encodes multiple proteins to overcome this environmental stress, including AhpC, a protein that catalyzes the reduction of organic peroxides to alcohols; NapA, an iron-binding neutrophil-activating protein; and SodB, a superoxide dismutase. Bacterioferritin comigratory protein, encoded by bcp , is a thiol peroxidase that utilizes linoleic acid as its substrate. Inactivation of each of these genes resulted in enhanced sensitivity to either paraquat and cumene hydroperoxide ( ahpC − and bcp ) or oxygen ( napA − ), respectively, while an ahpC − / napA − double mutant exhibited the highest sensitivity to growth inhibition by oxygen and organic peroxides. Interestingly, a novel H. pylori protein (HP0630) was upregulated in the ahpC − / napA − mutant, suggesting that it may function as a compensatory oxidative stress-resistance constituent. This group also investigated whether ahpC, bcp, or another gene involved in antioxidant defense, tpx (encoding thiolperoxidase), were required for in vivo colonization. Inactivation of any of these genes led to a severe impairment in the ability of H. pylori to infect mice, suggesting that oxidative resistance is a critical factor for successful colonization. Interestingly, NapA is not required for colonization of mice, although napA − mutants do not survive well in competition experiments with wild-type H. pylori.
Another level of host defense that must be circumvented by H. pylori is innate immunity. Toll-like receptors (TLRs) are an evolutionarily conserved family of eukaryotic receptors that function in innate immunity via recognition of invariant regions in bacterial molecules termed pathogen- or microbe-associated molecular patterns ( Fig. 63.2 ). Thirteen different TLRs have been identified in mammals and although the bacterial ligands for TLRs are distinct, the intracellular signaling pathways activated by these receptors all appear to eventuate in NF-κB activation and pro-inflammatory gene expression ( Fig. 63.2 ). It is becoming increasingly clear; however, that H. pylori has evolved strategies to avoid global activation of this system. For example, TLR4 generally recognizes bacterial LPS, yet H. pylori LPS is relatively anergic compared with that of other enteric bacteria (e.g., Escherichia coli ). This is primarily due to lipid A core modifications as H. pylori LPS contains only four fatty acids compared with the more typical six-component fatty acid structure present in other bioactive LPS molecules. Further, H. pylori can upregulate TLR4 and utilize it as a receptor to adhere to epithelial cells. Bacterial flagellin is typically recognized by TLR5, which is present on the gastric epithelium. However, in contrast to flagellins expressed and secreted by Gram-negative mucosal pathogens such as Salmonella or E. coli , which robustly activate TLR5-mediated pro-inflammatory responses, H. pylori flagellin is not secreted and is noninflammatory. Finally, recent evidence has demonstrated that H. pylori can activate TLR9 via DNA translocation resulting in an antiinflammatory response.

Chronic gastric inflammation induced by H. pylori is considered a T-helper lymphocyte 1 (Th1) response leading to increased production of interferon gamma (IFN-γ). H. pylori has been reported to interfere with IL-4-dependent Stat6 signaling and blunt IFNγ-mediated activation of gastric epithelial cells in vitro , and H. pylori infection is associated with increased mucosal levels of IL-10, an antiinflammatory peptide that inhibits secretion of pro-inflammatory chemokines from macrophages and neutrophils.
Experimental infection of IL-10 − / − mice with either H. pylori or a related Helicobacter species, Helicobacter felis , leads to a significant decrease in colonization in conjunction with a more severe inflammatory response compared to infected IL-10 + / − heterozygotes or wild-type controls, suggesting that microbially induced IL-10 may attenuate an appropriately targeted gastric inflammatory response that has the capacity to eradicate H. pylori from the stomach. Further, ex vivo experiments using H. pylori -infected human biopsies demonstrated that binding of IL-12 to its receptor leads to STAT4 phosphorylation and increased levels of IFN-γ, indicating its role in the H. pylori – associated Th1 phenotype. In addition to production of IL-12 by macrophages and dendritic cells (DCs), studies have indicated that the p40 heavy chain subunit is also expressed in gastric epithelial cells and T cells.
IL-17 levels are also higher in human and mouse gastric tissue compared with uninfected samples. Mice treated with an anti-IL-17 antibody exhibited lower levels of H. pylori colonization as well as inflammation. In H. pylori -infected mice lacking an IL-17 receptor subunit, IL-17RA, transcript levels of IL-17 were higher as was H. pylori colonization density and inflammation. Together, these data suggest that IL-17 induction may be important in both H. pylori colonization and pathologic responses.
H. pylori can also facilitate its own persistence by varying the antigenic repertoire of surface-exposed proteins and by actively suppressing the host-adaptive immune response. Since Lewis antigens are expressed on gastric epithelial cell surfaces, a potential biological role for such antigens is molecular mimicry ( Table 63.1 ). This may allow H. pylori to escape host immunological detection by preventing the formation of antibodies directed against shared bacterial and host epitopes, thus facilitating persistent infection. Initially, human and animal studies supported this paradigm by demonstrating a significant concordance between the Lewis phenotype of the host and the infecting Helicobacter strain. However, two studies challenged these observations by failing to show a significant relationship between the human host Lewis phenotype and that of the corresponding colonizing H. pylori isolates. Moreover, strains that express both Lewis x and Lewis y can be isolated from a single human host. An in vivo experiment with Le b -expressing transgenic mice challenged with Le x and Le y positive strains led to the recovery of H. pylori that expressed only Le b and this change was associated with a putative galactosyltransferase. These data suggest that Lewis antigens may facilitate molecular mimicry and permit H. pylori to evade recognition by host immune defenses.
Finally, H. pylori can survive and replicate within epithelial cells and macrophages, thereby evading host clearance. The ability of H. pylori to invade epithelial cells has been investigated using differential interference contrast video and immunofluorescence microscopy. Using these techniques, H. pylori was found to reside within large cytoplasmic vacuoles, and following vacuole evolution, the reappearance of H. pylori in the extracellular environment was observed in parallel with the disappearance of intravacuolar bacteria, suggesting release from intravacuolar sites. At the ultrastructural level, the entry process was reported to occur via a zipper-like mechanism, as internalized bacteria were bound within phagolysosomes in close association with condensed filamentous acti. Zheng and Jones reported that virulent H. pylori strains can inhibit phagosome development following bacterial ingestion by macrophages, which is associated with retention of coronin 1. However, despite these multiple strategies for evading host clearance, substantial immune activation still occurs as manifested by epithelial pro-inflammatory cytokine release, infiltration of the gastric mucosa by inflammatory cells, and cellular and humoral recognition of H. pylori antigens.
63.1.3
Development of Gastritis
63.1.3.1
H. pylori Contact-Mediated Cytokine Release and the Development of Acute Inflammation
The gastric inflammatory response induced by H. pylori consists of neutrophils, lymphocytes (T and B cells), plasma cells, and macrophages, along with varying degrees of epithelial cell degeneration and injury. One potential mechanism for induction of inflammation is that H. pylori secrete substances that stimulate mucosal inflammation from afar. For example, urease has been detected within the gastric lamina propria, and the urease complex of H. pylori stimulates chemotaxis by both monocytes and neutrophils and activates mononuclear cells as well. Similarly, H. pylori porins and low-molecular-weight molecules, such as Paf-acether, possess chemotactic properties. Finally, H. pylori water extracts promote neutrophil-endothelial cell interactions in vitro and increase leukocyte adherance via CD11a/CD18 and CD11b/CD18 interactions with intercellular adhesion molecule type-1 (ICAM-1).
The presence of acute inflammatory components within H. pylori -infected mucosa suggests that soluble mediators that are capable of attracting polymorphonuclear cells (PMNs) are key regulators in the development of gastritis. Therefore, another mechanism through which H. pylori may induce inflammation is via direct contact with gastric epithelial cells and stimulation of cytokine release. Gastric epithelium from infected persons contains increased levels of IL-1β, IL-2, IL-6, IL-8, and TNF-α compared with gastric mucosa from uninfected persons, and within this group of pro-inflammatory mediators, IL-8 has been studied most intensively as a mediator of H. pylori pathogenesis.
IL-8 is a potent neutrophil-activating chemotactic cytokine (chemokine) that is secreted by gastrointestinal (GI) epithelial cells in response to infection with pathogenic bacteria. IL-8 produced by activated enterocytes binds to the extracellular matrix and establishes a haptotactic gradient that directs inflammatory cell migration toward the epithelial cell surface. Consistent with observations for infected intestinal epithelial cells, expression of IL-8 within H. pylori -colonized gastric mucosa localizes to gastric epithelial cells, and levels of IL-8 are directly related to the severity of gastritis. In vitro, H. pylori stimulates IL-8 expression and release from gastric epithelial cells, and these events are dependent upon an active interplay between viable bacteria and epithelial cells. Thus, a paradigm for the acute component of H. pylori -induced gastric inflammation is that contact between bacteria and epithelial cells stimulates IL-8 secretion, which then regulates neutrophil infiltration into the gastric mucosa.
Pro-inflammatory cytokine expression is often regulated at the mRNA level by soluble transcription factors and the human IL-8 gene contains several motifs within its promoter region including binding sites for NF-κB, NF-IL-6, AP-1 (which is comprised of the binding elements c- fos and c- jun ), as well as an element that is homologous to an interferon-stimulated responsive element (ISRE; Fig. 63.3 ). NF-κB constitutes a family of transcription factors sequestered in the cytoplasm, whose activation is tightly controlled by a class of inhibitory proteins termed IκB’s. Multiple signals, including microbial contact, stimulate phosphorylation of IκB by IκB kinase β (IKKβ), which leads to proteasome-mediated degradation of phospho-IκB, thereby liberating NF-κB to enter the nucleus where it regulates transcription of a variety of genes, including immune response genes ( Fig. 63.3 ). Stimulation and activation of NF-κB does not require protein synthesis, thereby allowing efficient activation of target genes, such as IL-8. This system is particularly utilized in immune and inflammatory responses where rapid activation of defense genes following exposure to pathogens such as bacteria is critical for survival of an organism. Several studies have demonstrated that contact between H. pylori and gastric epithelial cells results in brisk activation of NF-κB, which is followed by increased IL-8 expression ( Fig. 63.3 ). Smith et al. delineated additional upstream mediators of H. pylori -induced NF-κB activation by transfecting HEK293 cells with TLR2 as well as an NF-κB reporter gene. Cotransfection of TLR2 conferred the ability to activate NF-κB and induce IL-8 production in response to different H. pylori strains. The ability of H. pylori to activate NF-κB in vitro has been corroborated in vivo as activated NF-κB is present within gastric epithelial cells of infected, but not uninfected patients, which mirrors the distribution pattern of increased IL-8 protein within colonized mucosa.

Mitogen-activated protein kinases (MAPK) also mediate H. pylori -dependent IL-8 expression. MAPK are signal transduction networks that target transcription factors such as AP-1 and participate in a diverse array of cellular functions, including cytokine expression. MAPK cascades are organized in three-kinase tiers consisting of a MAPK, a MAPK kinase (MKK), and a MKK kinase (MKKK), and transmission of signals occurs by sequential phosphorylation and activation of components specific to a respective cascade. In mammalian systems, at least five MAPK modules have been identified and characterized to date; these include extracellular signal-regulated kinases 1 and 2 (ERK 1/2), p38, and c-Jun N-terminal kinase (JNK). H. pylori activates p38, ERK 1/2, and JNK in gastric epithelial cells in vitro, and activation of ERK1/2 is dependent upon transactivation of the EGF receptor, a receptor tyrosine kinase.
Cell culture studies have revealed that H. pylori -induced IL-8 expression is dependent upon both NF-κB and MAPK activation of AP-1, and cross-talk between NF-κB and MAPK pathways has been demonstrated previously. For example, MEKK1, a hierarchical MAPK kinase, can directly activate the IκB kinase signalsome. However, inhibition of ERK 1/2 attenuates H. pylori -induced IL-8 secretion without affecting NF-κB, raising the possibility that synergistic interactions between AP-1 and NF-κB occur within the IL-8 promoter. Consistent with these data, H. pylori activation of ERK 1/2 results in enhanced c- fos transcription, indicating that ERK 1/2 may exert regulatory effects on IL-8 production that are primarily dependent upon AP-1 ( Fig. 63.3 ). Another layer of complexity exists in that maximal H. pylori -induced IL-8 gene transcription requires activation of NF-κB, AP-1, and ISREs within the IL-8 promoter ( Fig. 63.3 ). Finally, recent exciting data have shown that H. pylori can activate another pathway that mediates IL-8 production, which involves the adaptor protein TIFA. Activation of TIFA is a strain-specific effect that is dependent upon the cag pathogenicity island and translocation of a bacterial metabolite termed heptose-1,7-bisphosphate (HBP), an intermediate of heptose biosynthesis. Mutant H. pylori strains unable to synthesize HBP retain the ability to translocate CagA, but IL-8 induction is reduced by 95%. Collectively, these data indicate that the mucosal inflammatory reaction that develops in response to H. pylori involves multiple different pathways converging on the IL-8 promoter and that H. pylori -mediated host signaling is of central importance for understanding the inflammatory response to this pathogen, which if left untreated over decades, may progress to peptic ulceration or gastric cancer.
63.1.3.2
Humoral Responses to H. pylori
Although H. pylori colonization induces an exuberant systemic and mucosal humoral response directed at multiple antigens, antibody production does not result in eradication even though this organism is susceptible in vitro to antibody-dependent complement-mediated phagocytosis and killing. The ineffective humoral response generated against H. pylori and its components have led some investigators to speculate that this may actually contribute to pathogenesis. For example, a potential consequence of H. pylori Lewis antigen expression is induction of a humoral response that enhances gastric inflammation and injury. H. pylori infection in mice induces anti-Lewis x antibodies with an affinity for parietal cells and immunization of mice leads to the development of anti-Lewis x/y antibodies that cross-react with host Lewis x/y antigens present on the β-chain of the gastric H + K + -ATPase located in parietal cell canaliculi. In humans, H. pylori infection similarly induces autoantibodies directed against the canaliculi of gastric parietal cells. IgM antibodies generated by immortalized B cells obtained from H. pylori -colonized gastric mucosa also recognize gastric epithelium. These findings indicate that the humoral response to H. pylori may contribute to the development of distinct histologic lesions. For example, an autoimmune reaction against parietal cells may lead to gastric atrophy with a concomitant reduction in gastric acidity; conversely, immunoglobulin-mediated destruction of epithelial cells may initiate and/or maintain mucosal inflammation and epithelial cell injury.
63.1.3.3
Cell-Mediated Adaptive Immune Responses to H. pylori
The ability of the GI tract to discern pathogenic bacteria from commensals is regulated through T-cell-dependent responses. CD4 + T cells can be broadly divided into two functional subsets, type 1 (Th1/Th17) and type 2 (Th2) T-helper cells, each of which are defined by distinct patterns of cytokine secretion. Th1 cells produce IL-2 and IFN-γ and promote cell-mediated immune responses while Th2 cells secrete IL-4, IL-5, IL-6, and IL-10 and induce B-cell activation and differentiation. The type of immune response to a particular microbial agent is governed by preferential expansion of one T helper cell subset accompanied by a corresponding and relative downregulation of the other. In general, most intracellular bacteria induce Th1 responses, while extracellular pathogens stimulate Th2 type responses. The importance of CD4 + T cells in H. pylori -induced inflammation is underscored by studies using genetic models of immunodeficiency. Compared to wild-type mice, severe combined immunodeficiency (SCID) mice, T-cell deficient mice, or recombinase activating gene (RAG)-deficient mice infected with Helicobacter develop less severe mucosal injury despite similar levels of bacterial density, while infected B-cell deficient mice are no different than wild-type littermates in the progression to gastric inflammation and injury.
Although the acquired immune response to H. pylori is composed of both Th1- and Th2-type cells, cytokine profiles indicate a Th1/Th17 predominance, as the majority of H. pylori antigen-specific T-cell clones isolated from infected gastric mucosa produce higher levels of IFN-γ than IL-4. Consistent with these observations, H. pylori stimulates production of IL-12 in vitro, a cytokine that promotes Th1 differentiation. This is somewhat counterintuitive because H. pylori is primarily noninvasive and that infection is accompanied by an exuberant humoral response, but additional studies now suggest that this Th1-biased response may actually play an important role in pathogenesis. H. pylori infection of IFN-γ-deficient mice (that fail to mount an appropriate Th1 response) leads to decreased levels of gastric inflammation and atrophy compared to wild-type mice. In vivo neutralization of IFN-γ in mice infected with H. felis similarly reduces the severity of gastritis, while gastric inflammation and atrophy can be correspondingly induced by delivering infusions of IFN-γ, even in the absence of Helicobacter. Certain strains of mice (C57/BL6) infected with H. felis that mount a polarized Th1-type response develop extensive gastric inflammation, while other genetically distinct murine strains (Balb/c) that respond to infection with a Th2-like response, develop only minimal gastritis. Adoptive transfer of Th1-type cells from Helicobacter -infected donor mice into infected recipients increases the severity of gastritis, while transfer of Th2-type lymphocytes reduces colonization density. Consistent with these results, antecedent challenge with a helminth ( Heligmosomoides polygyrus ) that induces a Th2-type mucosal reaction significantly attenuates the development of Th1-mediated gastritis and atrophy in response to H. felis , while induction of a Th1 response in a Th2 responding host (Balb/c mice) enhances gastric injury following infection with H. felis .
Th17 cells represent another subset of T helper cells and are characterized by their production of IL-17, IL-21, and IL-22. Several studies have found that IL-17α is expressed in the stomachs of both humans and mice infected with H. pylori , suggesting that Th17 may also have a role in H. pylori -mediated gastric inflammation.
Regulatory T cells (Treg) have also been implicated in modulating the immune response directed against H. pylori . Increased levels of these CD4 + CD25 high effector cells have been found in both the gastric mucosa and circulation of H. pylori -infected persons as compared with controls. Two studies have demonstrated that CD4 + CD25 + Treg cells may limit the host inflammatory response by decreasing activation of CD4 + T cells within inflamed mucosa. Further, H. pylori -associated gastric adenocarcinoma tissue was found to have more CD4 + CD25 high cells than adjacent tissue. Interestingly patients with peptic ulcer disease had a lower CD4 + CD25 high Treg response, but higher Th1 and Th2 responses when compared to patients without ulcers. Together, these data suggest that an imbalance of the T cell responses may impact the development of H. pylori -associated diseases as well as affecting H. pylori persistence.
DCs represent a critical link between innate and adaptive immune responses and are the primary sensors of bacterial products and also serve as antigen presenting cells. DCs can penetrate the intestinal epithelial barrier in vivo and take up bacteria and disruption of the epithelial apical-junctional complexes by H. pylori may further enhance DC interaction with H. pylori . DCs recognize the H. pylori OMPs HpaA and Omp18, which induce DC maturation and antigen presentation, events that are independent of cag PAI status and vacA genotype. Further, live bacteria are not required for activation of cytokine production.
Surface receptors on DCs (e.g., C-type lectins) recognize carbohydrate structures and, upon binding, internalize pathogens for antigen processing and presentation to T cells. The DC-specific C-type lectin ICAM-3-grabbing nonintegrin (DC-SIGN, CD209) is involved in binding of the HIV-1 envelope glycoprotein to enhance infection of T cells. Common features of pathogens that interact with DC-SIGN include chronicity and the ability to manipulate the Th1/Th2 response, and binding of mannosylated and fucosylated surface glycans of H. pylori to DC-SIGN has been reported by Appelmelk et al. H. pylori also induces the release of Th1-type cytokines (e.g. IL-12) from DCs in vitro, raising the hypothesis that interactions between H. pylori and DCs may influence antigen presentation as well as cytokine secretion, thereby contributing to the persistent inflammatory response induced by this pathogen. Collectively, these data are consistent with a model in which an inappropriate host T-cell response toward H. pylori facilitates the development of gastric inflammation and injury.
63.1.3.4
Additional Host Effectors That Regulate H. pylori -Induced Inflammation and Progression to Disease
Cyclooxygenases catalyze key steps in the conversion of arachidonic acid to endoperoxide (PGH 2 ), a substrate for a variety of prostaglandin synthases that, in turn, catalyze the formation of prostaglandins and other eicosanoids ( Fig. 63.4 ). Prostaglandins regulate a diverse array of physiologic processes including immunity, maintenance of vascular tone and integrity, nerve development, and bone metabolism . Three isoforms of cyclooxygenase have been identified, each possessing similar activities, but differing in expression characteristics and inhibition profiles by NSAIDs. COX-1 and COX-3 (a splice variant of COX-1) are expressed constitutively in many cells and tissues. In contrast, COX-2 expression can be induced by a variety of growth factors and pro-inflammatory cytokines such as TNF-α, IFN-γ, and IL-1 in a number of pathophysiologic conditions. COX-2 expression is increased in epithelial cells cocultured with H. pylori . and within gastric mucosa of H. pylori- infected individuals. COX-2 expression is further increased within H. pylori -induced premalignant (atrophic gastritis and intestinal metaplasia) and malignant (adenocarcinoma) lesions and is dependent on EGFR activation. In addition, COX-inhibitors such as aspirin and other nonsteroidal antiinflammatory drugs have been shown to decrease the risk for distal gastric cancer.

The inflammatory response induced by H. pylori leads to the production of mutagenic substances, such as metabolites of inducible nitric oxide synthase (iNOS), which promote oncogenesis. iNOS-generated nitric oxide can be converted to reactive nitrogen species, which nitrosylates a variety of cellular targets including DNA and proteins. Superoxide anion radicals generated by PMNs also induce DNA damage through the formation of DNA adducts. Mice that are deficient in iNOS exhibit decreased levels of H. pylori -induced gastric cancer and serum levels of vitamin C, a scavenger of reactive oxygen species and nitrates, are inversely proportional to the prevalence of gastric cancer. Eradication of H. pylori has been reported to raise gastric intraluminal ascorbic acid levels, so the presence of H. pylori also affects gastric mucosal antioxidant defenses.
63.1.4
H. pylori Strain Variation, Gastric Inflammation, and Disease
Colonization of humans by H. pylori is relatively common, but the development of ulcer disease or cancer follows in only a fraction of infected persons. Consideration must be given to strain-specific differences that exist among H. pylori isolates, as well as the functions associated with such differences. H. pylori strains from different individuals are extremely diverse due to point mutations, gene insertions, and/or deletions and genetically unique derivatives of a single strain are present simultaneously within an individual human host and can modify their genetic composition over time. Although this extraordinary diversity has hindered characterization of bacterial factors associated with various disease outcomes, different genetic loci have been identified for which persons harboring particular alleles have different risks of disease. These markers are not completely independent of each other and importantly, are not absolutes but instead reflect degrees of risk.
63.1.4.1
The cag Pathogenicity Island
The most well-characterized H. pylori virulence determinant is the cag pathogenicity island, a horizontally acquired 27–31 gene locus that is present in ~ 60% of US strains. Although all H. pylori strains induce gastritis, cag + strains significantly augment the risk for severe gastritis, peptic ulcer disease, atrophic gastritis, and distal gastric cancer compared to that incurred by cag − strains. Several cag genes encode products that bear homology to components of a type IV bacterial secretion system, which functions as a molecular syringe to export proteins, and transmission electron microscopy has permitted detailed visualization of the secretion apparatus. CagL, encoded by the cag island, interacts directly with host cell integrins, leading to local membrane ruffling, the clustering of integrins into focal adhesion complexes, and activation of the host cell kinases Src and focal adhesion kinase (FAK). Additionally, the proteins CagA, CagI, and CagY have been shown to bind β1 integrin and induce conformational changes of integrin heterodimers.
The product of the terminal gene in the island (CagA) is translocated into host epithelial cells by the cag secretion system after bacterial attachment, where it undergoes tyrosine phosphorylation by members of the Src family of kinases ( Fig. 63.5 ). Phospho-CagA subsequently activates a eukaryotic phosphatase (SHP-2) as well as ERK, a member of the MAPK family, leading to morphological changes (e.g. cell scattering) that are reminiscent of unrestrained stimulation by growth factors ( Fig. 63.5 ). These in vitro observations mirror events occurring within inflamed mucosa as CagA is translocated into and phosphorylated within epithelial cells and binds SHP-2 in vivo. Manipulation of the IL-6 family coreceptor gp130 leading to altered SHP-2 signaling similarly culminates in the development of intestinal-type gastric adenocarcinoma in genetically engineered mice. ERK can also be activated within H. pylori -infected cells by other host-signaling pathways. H. pylori cag + strains selectively induce transactivation of the EGF receptor via activation of HB-EGF in vitro, and H. pylori -induced EGF receptor transactivation is required for Ras-mediated activation of ERK and for Akt-dependent activation of β-catenin ( Fig. 63.5 ). CagA has also been shown to bind to the c-Met receptor, which stimulates a mitogenic response in gastric epithelial cells that is dependent upon ERK signaling.

Due to the ability of CagA to commandeer multiple host pathways, investigators have sought to define differences in the biological activity of CagA proteins that are present in different H. pylori strains. Higashi et al. demonstrated that the number of tyrosine phosphorylation motifs (TPMs) within CagA proteins isolated from persons residing in Western countries can vary substantially and that SHP-2 binding affinity and induction of cell scattering are potentiated by an increasing number of such motifs. In contrast, TPMs within CagA proteins from East Asian H. pylori strains are unique and the sequences flanking East Asian-type TPMs perfectly match the consensus binding site for SHP-2. As expected, binding of SHP-2 and cell scattering are induced more potently by CagA proteins containing East Asian-type TPMs compared with Western-type TPMs. Several in vitro studies have indicated that the number of phosphorylation motifs is also associated with the degree of CagA phosphorylation as well as cellular morphological changes and the induction of proinflammatory cytokines. Consistent with these in vitro findings, clinical studies have demonstrated that H. pylori strains with a functional cag island and an increased number of CagA TPMs are more closely associated with gastric atrophy and gastric cancer, which may explain, in part, the strikingly different rates of gastric cancer in these regions.
In addition to the effects of phospho-CagA on signaling pathways that alter cellular morphology, CagA phosphorylation also results in the activation of C-terminal Src kinase, which inhibits the activity of Src, leading to a reciprocal decrease in the level of phosphorylated CagA. Inactivation of c-Src also leads to dephosphorylation of cortactin, an actin-binding protein, which is subsequently redistributed to cellular protrusions. Although this negative feedback loop likely contributes to the long-term equilibrium between H. pylori and its host, unphosphorylated CagA can also exert effects within the cell that contribute to pathogenesis. For example, unmodified CagA binds to growth factor receptor bound 2 (Grb2), which results in activation of the Ras/MEK/ERK MAPK pathway. Translocation, but not phosphorylation, of CagA leads to disruption of apical-junctional complexes in polarized epithelial cells and a loss of cellular polarity, alterations that have previously been shown to play a role in carcinogenesis. The use of an ex vivo gastroid (aka gastric organoid) model has demonstrated that H. pylori induces mislocalization of the tight junction protein claudin-7 in a CagA-β-catenin-dependent manner. Intracellular CagA also binds to E-cadherin at adherens junctions and induces aberrant activation of β-catenin. In addition, it has been shown that CagA interacts directly with PAR1/MARK kinase inhibiting its activity and leading to the disruption of epithelial cell polarity and pertubation of microtubule formation.
Another consequence of cag -mediated epithelial contact is secretion of IL-8. Clinical observations that the cag island represented a disease-associated locus led to subsequent molecular investigations and the first H. pylori strain-specific constituent identified as being required for IL-8 production was a structural component of the cag island, cagE . Inactivation of cagE not only attenuates IL-8 expression but also decreases activation of NF-κB and MAPK in vitro. Numerous cag island genes ( cagG , cagH , cagI , cagL , cagM , and cagY ) have now been demonstrated to be required for NF-κB and MAPK-mediated IL-8 production, , and clinical cag + strains are more potent in stimulating IL-8 production than cag − strains. Consistent with these findings, H. pylori cag + strains induce an enhanced IL-8 and inflammatory response in human tissue, and inactivation of cagE and/or the entire cag locus attenuates the development of gastritis and atrophy in a rodent model of H. pylori -induced gastric adenocarcinoma, Mongolian gerbils. In terms of substrates translocated by the cag island that lead to IL-8 production, recent work has identified that HBP can induce IL-8 secretion in a strain-specific manner.
The effects of cag island components on additional host responses have also been explored. Using eukaryotic microarrays, Guillemin et al. transcriptionally profiled AGS gastric epithelial cells that were cocultured with H. pylori wild-type or isogenic mutant strains. An isogenic cagA − mutant induced expression of fewer cytoskeletal-related genes compared to the parental strain and, as expected, inactivation of cagE resulted in a loss of the ability to upregulate cagA -dependent genes as well as a number of immune response genes. However, the isogenic cagE mutant retained the ability to induce expression of a subset of host genes that function in cellular recognition events including IFN-γ, plasminogen, endothelin-1, and TFF1. These genes were not induced by an H. pylori cag island deletion mutant, indicating that host cells respond differently to strains lacking the entire cag island and those with a defective secretory system.
Some H. pylori strains have been shown to activate NF-κB via CagA, although this property is not universal. Viala et al. demonstrated that H. pylori peptidoglycan can also be delivered by the cag secretion system and is recognized by intracellular Nod1, a member of a family of intracytoplasmic pathogen-recognition molecules. An additional study has also indicated that H. pylori peptidoglycan can also be delivered intracellularly via bacterial outer membrane vesicles through lipid rafts. Nod1 sensing of H. pylori peptidoglycan activates NF-κB, p38, and ERK leading to expression of IL-8 and MIP-2, and Nod1-deficient mice are more susceptible to infection by H. pylori cag + strains compared to wild-type mice. Further, peptidoglycan activation of NOD1 has a role in MAPK and AP-1 activation and translocated peptidoglycan leads to enhanced PI3K-AKT signaling, which results in decreased H. pylori -induced apoptosis and increased cell migration. NOD1 activation also leads to production of type I IFN and may result in aberrant regulation of Th1 cell differentiation. However, NOD1 activation can also activate a negative feedback pathway and result in attenuated inflammation within the context of H. pylori infection. Collectively, these data indicate that cag + strains are disproportionately represented among hosts who develop serious sequelae of H. pylori infection and that genes within the cag island are necessary for induction of pathogenic epithelial responses, which may heighten the risk for disease, particularly over prolonged periods of colonization.
63.1.4.2
The H. pylori Vacuolating Cytotoxin
The aforementioned studies have contributed to the genesis of a molecular portrait through which CagA and the cag island manipulate host signaling pathways that regulate cellular responses and cytokine production. However, most persons colonized by cag + strains remain completely asymptomatic. This paradox has fostered the need for studies to identify other microbial factors that may influence disease.
An independent H. pylori locus linked with pathologic outcomes such as peptic ulcer disease and gastric cancer is vacA , which encodes a secreted bacterial toxin (VacA). When added to epithelial cells in vitro, VacA induces multiple structural and functional alterations in cells, the most prominent of which is the formation of large intracellular vacuoles. vacA encodes a precursor protein of ~ 140 kD, which is processed to yield a mature 87 kD protein (VacA). Mature VacA monomers then assemble into oligomeric structures that are composed of 12–14 subunits, when subjected to acid or alkaline conditions, the VacA oligomer disassembles but retains the capacity to reanneal after pH neutralization. Acid activation also enhances the interaction of VacA with cellular membranes in vitro by increasing its insertion into artificial lipid bilayers where it forms hexameric, anion channels. Cytoplasmic internalization of VacA is mediated by active cellular processes and is required for cytotoxicity and vacuole formation. Vacuolation is dependent upon host factors such as V-ATPase, Rab7, Rac1, dynamin, and PIKfyve previously implicated in endosome formation. VacA also induces alterations in late endocytic compartments leading to inhibition of EGF degradation, clustering of endocytic compartments, and the inhibition of antigen presentation.
There is a strong correlation between vacuolating cytotoxin activity and the presence of cagA . However, vacA and cagA map to two distinct loci on the H. pylori chromosome, and expression of the two proteins is independent. Unlike the cag island, vacA is present in virtually all H. pylori strains examined ; however, strains vary considerably in production of cytotoxin activity due primarily to variations in vacA gene structure. The regions of greatest diversity are localized near the 5′ end of vacA (allele types s1a, s1b, s1c, or s2) and in the mid-region of vacA (allele types m1 or m2). An additional polymorphic region, the intermediate (i) region has also been identified. H. pylori strains that possess a type s1/m1/i1 vacA allele are associated with an increased risk of peptic ulcer disease and gastric cancer. and enhanced gastric epithelial cell injury compared to vacA s2/m2/i2 strains. The relationship between s1/m1 alleles and gastric cancer is consistent with the distribution of vacA genotypes throughout the world. In regions where the background prevalence rate of distal gastric cancer is high, such as Colombia and Japan, most H. pylori strains contain type s1/m1 alleles, whereas the converse is true in regions of the world with low rates of distal gastric adenocarcinoma, underscoring the importance of vacA as a bacterial locus related to high-grade host responses within the gastric niche.
Most type s1 VacA toxins possess detectable cytotoxic activity in vitro, whereas type s2 VacA proteins possess little if any cytotoxic activity. This is attributable to the presence of a 12-amino acid hydrophilic segment at the N-terminus of type s2 toxins, which abolishes cytotoxic activity. The mid-region of VacA contains a cell-binding site; m1-type toxins exhibit higher binding affinities to host cells than do m2-type toxins, and VacA has been demonstrated to bind to a unique receptor-type protein tyrosine phosphatase, PTPζ, a member of a family of receptor-like enzymes that regulate cellular proliferation, differentiation, and adhesion. Oral delivery of purified VacA induces gastric inflammation, hemorrhage, and ulcers, but only in PTPζ + / + mice, and in vitro, VacA treatment of PTPζ + / + , but not PTPζ − / − , cells induces cellular detachment, which may contribute to H. pylori -induced ulcerogenesis in humans.
In addition to vacuolation and cellular detachment, VacA induces gastric epithelial cell apoptosis, a form of programmed cell death that likely contributes to the development of ulcer disease and gastric cancer. Cover et al. demonstrated that VacA-induced apoptosis is dependent upon motifs present within the NH 2 terminus of VacA, suggesting that differences in levels of apoptosis among H. pylori -infected persons may result from strain-dependent variations in VacA structure. The eukaryotic mediators of toxin-induced apoptosis have also been identified, as treatment of cells with purified VacA leads to the release of mitochondrial cytochrome c, implicating the mitochondrial apoptotic pathway in VacA-induced programmed cell death. VacA also functions as transmembrane pore, permeabilizing host epithelial cells to urea, which, in turn, may allow H. pylori to manipulate the pH of its environment by generating ammonia. When added to polarized epithelial cell monolayers, acid-activated VacA increases paracellular permeability to organic molecules, iron, and nickel. Cellular extracts from toxigenic H. pylori strains increase vascular permeability, leukocyte adhesion, platelet aggregation, and vasoconstriction within the gastric mucosal microcirculation of rats.
VacA has also been shown to actively suppress T cell proliferation and activation in vitro, which may contribute to the longevity of H. pylori colonization ( Table 63.1 ). VacA binds integrin β2 (CD18) blocking antigen-dependent proliferation of T cells, in turn inhibiting activation of nuclear factor of activated T cells (NFAT) and its target genes ILR and the high-affinity IL-2 receptor-α. Collectively, these data indicate that VacA can induce multiple physiologic consequences that may contribute to pathogenesis.
63.1.4.3
H. pylori BabA and Additional OMPs
Sequence analysis of the genomes from H. pylori strains 26695, J99, and HPAG1 has revealed that an unusually high proportion (1%) of identified open reading frames are predicted to encode OMPs, which may represent novel H. pylori adhesions, and consequently, recent attention has been directed toward a possible role of these OMPs in H. pylori pathogenesis. BabA is a membrane-bound adhesin encoded by the strain-selective gene babA2 that binds the blood-group antigen Lewis b (also known as MUC5AC) present on gastric epithelial cell membranes, and H. pylori strains possessing babA2 are associated with increased risk for gastric adenocarcinoma. BabA binding has been shown to be acid responsive, such that the binding affinity is dependent on local pH and allows for adaption to changes in the gastric mucosa. The presence of the gene-encoding BabA is associated with cagA and vacA s1; strains that possess all three of these genes incur the highest risk for gastric cancer. Further, data indicate that expression of BabA increases expression of CCL5 and IL-8 in conjunction with the cag secretion system. Focusing on gastric cancer precursor lesions, Yu et al. reported that H. pylori babA + strains are associated with an increased risk of glandular atrophy, intestinal metaplasia, and enhanced epithelial cell proliferation compared to strains that lack BabA, which may explain in part the augmentation in gastric cancer risk associated with these strains.
Another adherence-related OMP is HopQ, encoded by a gene that has been shown to covary with the cag pathogenicity island. Cao and Cover identified two distinct families of hopQ alleles and demonstrated that type I alleles are found significantly more commonly in H. pylori cag + vacA s1 strains isolated from ulcer patients than in cag − vacA s2 strains harvested from patients without ulceration. Two studies have now shown that HopQ can bind to epithelial CEACAM proteins. There is additional evidence, that in some strains, HopQ can attenuate adherence of H. pylori to gastric epithelial cells. At the same time, evidence suggests that HopQ is required for function of the cag secretion system. Together, these data suggest that this locus may have an important role in virulence.
Mahdavi et al. identified sialyl-dimeric-Lewis x glycosphingolipid as a receptor for H. pylori and demonstrated that bacterial colonization induced expression of sialyl-Lewis x antigens in human and monkey gastric mucosa. The H. pylori adhesin required for binding was identified as SabA, and since sialyl-Lewis x antigens are established tumor antigens and markers of gastric dysplasia that is upregulated by chronic gastric inflammation, these results further emphasize the pivotal role of H. pylori adherence in the induction of gastric inflammation and injury. The regulation of SabA expression is complex, but allows for control at the level of bacterial population as well as control at the cellular level.
Another H. pylori OMP that may mediate disease is a 34 kD proinflammatory protein encoded by oipA , also known as HopH. The vast majority of cag + strains isolated from patients in East Asia have an in-frame copy of oipA and when cocultured with gastric epithelial cells in vitro, these strains were found to induce high levels of IL-8. Inactivation of oipA decreased IL-8 levels by ~ 40%, while inactivation of both oipA and cagE in the same strain completely abolished IL-8 production. Analysis of 200 clinical isolates from children and young adults further supports a role for OipA in IL-8 expression. A study of IL-8 promoter activation in gastric epithelial cells revealed that maximal H. pylori -induced IL-8 transcription requires the presence of NF-κB, AP-1, and the ISRE-like element, and that oipA selectively induced STAT1 phosphorylation, which functions as an upstream mediator of ISRE activation ( Fig. 63.3 ). Utilizing the Mongolian gerbil model of H. pylori infection, inactivation of oipA led to attenuation of NF-kB translocation to the nucleus and lowered the rated of carcinoma. H. pylori strains that contain an in-frame, or functional, copy of oipA are linked with more severe gastric inflammation, higher bacterial colonization density, enhanced mucosal levels of IL-8, and duodenal ulcer disease. In a murine model of H. pylori -induced gastritis, the ability of H. pylori to successfully colonize C57Bl/6 mice was shown to be dependent on the in-frame status of not only OipA, but also several other OMPs (HopZ, HopO, and HopP), as strains that possessed > 2 out-of-frame OMPs were completely unable to establish infection. Infection with an isogenic oipA mutant led to a significant decrease in the severity of gastritis as well as a reduction in chemokine production compared to levels induced by the parental wild-type H. pylori strain, indicating a potential role of OipA in H. pylori pathogenesis.
63.1.4.4
FldA and Risk for Gastric Mucosa-Associated Lymphoid Tissue (MALT) Lymphoma
Another H. pylori antigen that is significantly associated with a specific clinical sequelae of long-term colonization is FldA, a putative flavodoxin protein. Chang et al. reported that this 19-kD protein is recognized by sera from H. pylori -infected patients with MALT lymphoma significantly more frequently than by sera from H. pylori -colonized patients without lymphoma. These investigators also found that an 11-amino acid truncation of the FldA protein was more common among H. pylori strains isolated from MALT patients (9/9) than in control strains (6/17). However, FldA antibodies are not universally present among patients with MALT lymphomas nor universally absent among controls, indicating that they are neither necessary nor sufficient for disease to occur. A proteomic study found that strains from patients with chronic gastritis express more FldA than those from patients with gastric ulcer or cancer. It remains to be determined whether FldA plays an important causal role in MALT development or, alternatively, simply may be a marker for strains associated with this neoplasm.
63.1.4.5
Novel H. pylori Virulence Components
Identification of additional strain-specific genes related to pathogenesis has been facilitated by the use of H. pylori whole genome microarrays that contain representations of each known H. pylori gene from two fully sequenced strains. Microarray-based comparisons of genetic content between strains allow each gene within the genome to be sampled simultaneously, and since evolutionary pressures tend to select for the coinheritance of genes involved in common pathways, identification of genes that segregate with known H. pylori virulence-related loci may reveal functional relationships that are not evident from sequence data alone. Indeed, a subset of genes has been identified that covary with the presence of the cag island, including two that encode predicted OMPs: omp27 and babA . Thompson et al. examined H. pylori genes that were transcriptionally altered during transition from log to stationary phase. Expression of napA , flaA , and pfr (encoding an iron storage protein) were significantly increased during late log phase, suggesting that the virulence capacity of H. pylori is highest at this point in the growth phase. Using a similar microarray analysis, Forsyth et al. identified seven genes that were regulated by HP0164, which encodes an H. pylori sensory histidine kinase.
H. pylori arrays have also been used to identify putative virulence components that may regulate inflammation and injury in vivo. In one study, 25 gastric cancer strains and 71 control H. pylori strains were phenotyped based on their ability to bind two different classes of epithelial cell receptors. From this group, 13 binding and 2 nonbinding strains were selected for microarray analysis that identified a panel of variable genes ( n = 370), and then each strain was used to challenge germ-free transgenic mice that had previously been shown to develop enhanced pathology in response to H. pylori infection. Eighteen variable genes were directly related to the severity of the host response, and the proportion of genes encoding HsdS homologs was significantly higher in this pool compared to the pool of variable genes that were not related to pathology, suggesting that HsdS homologs may regulate expression of H. pylori virulence constituents within the gastric niche. Graham et al. used an RNA capture technique in conjunction with microarrays to identify H. pylori genes that were selectively expressed within gastric tissue. A small subset of H. pylori ORFs was consistently present within infected mucosa that was not detected in bacteria grown in vitro, and the majority of these transcripts encoded factors unique to H. pylori . Although many of these in vivo-selective RNAs encode hypothetical proteins, one (HP0228) is predicted to encode a membrane protein involved in the transport of sulfate anion, which is likely to be important for the in vivo survival of H. pylori . As part of a larger study, investigators analyzed the genome data for 63 H. pylori isolates present in the KEGG database and were able to infer gene pathways involved in pathogenesis. Genomic analyses of H. pylori isolates have exposed data indicating the high level of homologous recombination among strains as compared with other bacteria. In populations that are geographically isolated, this can lead to genetic “fingerprints” that indicate the geographic origin of an individual isolate. Comparison of numerous genomes has revealed population-specific patterns of genes under selective pressure, including genes related to pathogenesis such as vacA as well as several of unknown function. As whole-genome sequencing becomes more easily performed, more studies are likely to utilize this type of approach to identify potential virulence factors in H. pylori .
Another method used to identify candidate virulence factors is a global proteomics approach in which DIGE/MS was used to identify 26 differentially abundant proteins when comparing a strain that causes cancer in rodents to its noncarcinogenic progenitor strain. Specifically, a novel missense mutation of the flagellar protein FlaA was identified that affects structure and function of this important protein. These results have identified a group of candidate genes that may be expressed by H. pylori in response to environmental signals encountered in the stomach, many of which can be examined further using appropriate in vitro and rodent models of H. pylori : host interactions. This methodology was also used to identify changes that occur in the proteome of H. pylori within infected gerbils on iron replete or iron depleted diets including an increase in CagA, FlaA, FlaB, and UreB. Another proteomic approach determined that CagA, HopQ, and HP0746 levels increased under conditions of high salt, substantiating their role as virulence factors under these conditions.
The ability of additional pathogenesis-related determinants to influence disease has also been examined. Andermann et al. investigated the role of two predicted chemoreceptors, TlpA and TlpC, in establishing infection in mice. When mice were inoculated with individual strains, the isogenic tlpA − and tlpC − mutants colonized to the same extent as the parental strain. However, coinfection of either mutant with the wild-type strain resulted in an increased recovery fraction of wild-type strains, suggesting that tlpA − or tlpC − -encoded products may partially contribute to in vivo colonization. Numerous investigators have studied the chemoattractants for these receptors. Using both confocal and video microscopy complemented with in vivo models, Huang et al. determined that these chemoreceptors play a critical role in the relationship of H. pylori and acid, affecting the distribution and density of the bacteria that colonize the gastric epithelium.
Other less well-characterized loci have also been identified which, in the future, may become established pathogenic factors. Shibayama et al. identified a novel apoptosis-inducing H. pylori protein that also exhibits gamma-glutamyl transpeptidase (GGT) activity. Recently, the GGT of H. pylori has been shown to suppress the host T-cell response, inhibiting bacterial elimination and to have a role in H 2 O 2 generation as well as increasing DNA damage. Ando et al. identified a novel gene, hrgA , that replaces a previously identified H. pylori restriction endonuclease. Although the function of hrgA remains speculative, its presence is more common among H. pylori strains isolated from gastric cancer patients than from subjects with gastritis alone. Another H. pylori locus, JHP0947, was reported to be more frequently present in duodenal ulcer and gastric cancer strains than in strains harvested from patients without these lesions. The H. pylori locus dupA has been positively associated with duodenal ulcer disease and negatively linked to gastric atrophy and cancer. Hussein et al. reported that the dupA1 allele is associated with higher levels of IL-8 expression in infected gastric mucosa and, further, that this is due to an increase in proinflammatory cytokine production by mononuclear cells. If these genes are consistently found to be related to distinct disease outcomes, they may represent novel molecular epidemiologic markers, which could identify colonized persons at increased risk for clinical sequelae, who then might be considered for eradication therapy.
63.1.5
Human Genetic Polymorphisms That Influence the Propensity Toward Development of Disease
Although H. pylori strain-specific constituents clearly influence disease outcome, these factors are not absolute determinants of virulence. Most persons colonized with disease-associated H. pylori strains remain asymptomatic. Further, H. pylori cag + toxigenic strains are related to both duodenal ulcer disease and distal gastric cancer, two mutually exclusive disease outcomes. The inability of bacterial virulence components to completely account for pathologic outcomes has highlighted the need to explore host factors that may influence disease, particularly gastric cancer.
The critical components that regulate the H. pylori -induced cascade to carcinogenesis are (1) the presence of the bacterium, (2) the concomitant inflammatory response, and (3) a reduction in acid secretion, since inflammation that involves the acid-secreting gastric corpus increases the risk of developing gastric adenocarcinoma by inducing hypochlorhydria and atrophic gastritis. The Th1 cytokine IL-1β, a pleiotropic pro-inflammatory molecule that is increased within the gastric mucosa of H. pylori -infected persons, is a prime candidate that regulates such phenotypes. This molecule both amplifies host inflammatory responses and potently inhibits gastric acid secretion by parietal cells. Further, the IL-1β gene cluster, consisting of IL-1β and IL-1RN (encoding the naturally occurring IL-1β receptor antagonist), contains a number of functionally relevant polymorphisms that are associated with either increased or decreased IL-1β production and this has facilitated case-control studies to be performed that relate host genotypes and disease. Individuals with H. pylori and high-expressing IL-1β polymorphisms have a markedly increased risk for the development of hypochlorhydria, gastric atrophy, and distal gastric adenocarcinoma compared with individuals with alleles associated with low expression of IL-1β. These relationships were only present among H. pylori -colonized persons and not uninfected individuals, emphasizing the importance of host-environment interactions and inflammation in the progression to gastric cancer. These associations mirror alterations in mucosal IL-1β production induced by H. pylori as gastric tissue levels of this cytokine are significantly higher in colonized patients possessing high- versus low-expression IL-1β polymorphisms, and increased IL-1β levels are significantly related to the intensity of gastric inflammation and atrophy.
As described earlier, rodent models also support this paradigm, and IL-1β has been implicated as a pivotal modulator of acid secretion within inflammed mucosa. Since IL-1β is the most powerful known inhibitor of acid secretion, is profoundly pro-inflammatory, is upregulated by H. pylori , and is regulated by a promoter with informative polymorphisms, this molecule is likely to be of substantial importance in regulating the development of gastric cancer. A study in which transgenic mice overexpressed IL-1β in the stomach confirmed the role of IL-1β in H. pylori -associated gastric cancer as these mice progressed through stages from gastritis, followed by gastric atrophy to gastric adenocarcinoma similar to the disease progression in humans. Further, infection with H. pylori accelerated this progression.
Although IL-1β appears to be an ideal host candidate, other molecules are legitimate targets. For example, TNF-α is a pro-inflammatory acid-suppressive cytokine that is increased within H. pylori -colonized mucosa. TNF-α polymorphisms that increase TNF-α expression have now been associated with increased risk of gastric cancer and its precursors. Harris et al. reported that polymorphisms within the IL-8 promoter that increase IL-8 expression also augment the risk for atrophic gastritis among H. pylori -infected subjects. The pattern recognition receptor TLR4 is involved in the recognition of H. pylori and activates pro-inflammatory pathways, and a TLR4+ 896A > G polymorphism has been found to be a risk factor for noncardia gastric carcinoma. Conversely, polymorphisms that reduce the production of antiinflammatory cytokines may similarly increase the risk for gastric cancer, and El-Omar et al. demonstrated that “low-expression” polymorphisms within the locus controlling expression of the antiinflammatory cytokine IL-10 are associated with an enhanced risk for distal gastric cancer. This group also examined the combinatorial effect of polymorphisms within immune response genes on cancer risk and found that the relative risk for distal gastric cancer increased progressively with an increasing number of pro-inflammatory polymorphisms to the point that three polymorphisms increased the risk of cancer 27-fold over baseline. Polymorphisms in Muc1 are also associated with gastric carcinogenesis. In mice, Muc1 deficiency leads to increased IL-1β and Nlrp3 and infected mice begin to die within 6 months, supporting a role of Muc1 in the regulation of inflammation.
An important question raised by studies focused on immune response gene polymorphisms is whether H. pylori strain characteristics can further augment cancer risk exerted by host genotypes. To directly address this, Figueiredo et al. stratified H. pylori -infected subjects on the basis of both high-expression IL-1β polymorphisms and virulence genotype of their infecting H. pylori strains. Odds ratios for distal gastric cancer were greatest in those persons with high-risk host and bacterial genotypes; in persons with high-expression IL-1β alleles that were colonized by H, pylori vacA s1-type strains, the relative risk for gastric cancer was 87-fold over baseline, indicating that interactions between specific host and microbial characteristics are biologically significant for the development of gastric cancer and that combined bacterial/host genotyping determination may identify individuals at extremely high risk for gastric cancer. Importantly, a study of H. pylori -positive patients in Colombia found that those individuals with genetic ancestry (e.g., European) that differed from the ancestry of the H. pylori that they carried (e.g., African) had a greater risk of gastric disease than those with similar host and H. pylori ancestry.
On the basis of case-control studies in human populations, it is apparent that H. pylori is able to send and receive signals from their hosts, allowing host and bacteria to become linked in a dynamic equilibrium. The equilibrium is likely different for each colonized individual, as determined by both host and bacterial characteristics and may explain why certain H. pylori strains augment the risk for inflammation-induced carcinogenesis. For example, H. pylori cag + strains induce an intense inflammatory response that involves production of IL-8. This leads to increased production of IL-8, IL-1β, and TNF-α that cannot only amplify the mucosal inflammatory response, but may also inhibit acid production, especially in hosts with polymorphisms that permit high expression levels of these molecules. Although the host genotype remains stable over time, cag + and cag − strains can coexist and recombine within the same host, and thus the percentage of cag + strains is likely to vary over time, which will affect the amplitude of this equilibrium. An augmented inflammatory response induced by cag + strains in the gastric body leading to decreased acid production can also permit overgrowth of pH-sensitive bacteria, conversion of ingested N -nitrosamines to nitrites, and an increased risk for gastric cancer.
63.1.6
Environmental Conditions That Influence Disease Risk
Numerous studies have demonstrated a relationship between environmental factors such as diet and the risk of gastric cancer. In the context of H. pylori colonization, high salt intake and low iron levels are the most frequently associated with increased risk of gastric adenocarcinoma. Studies have demonstrated that high levels of dietary salt result in increased levels of CagA and OMP HopQ. Data also indicate that a diet deficient in iron leads to increased inflammation and acceleration of the development of gastric dysplasia and cancer in the gerbil model.
In addition to dietary factors, the resident gastric microbiota likely play a role in gastric pathogenesis. The gastric microbiota of H. pylori -negative individuals is highly diverse, harboring 10 1 to 10 3 CFU/g including between 128 and 262 phylotypes. However, the gastric microbiota of H. pylori -positive individuals is dominated by H. pylori , which comprises 93%–97% of all sequence reads. Further, the diversity of microbes detected is decreased. A study comparing the gastric microbiota of subjects from both high-risk and low-risk cancer populations in Colombia found that, despite variation among individuals, there were distinct differences between the microbiota of each risk group. These results provide a basis for future studies utilizing animal models of H. pylori infection to test the effect of altered gastric microbiota on cancer progression.
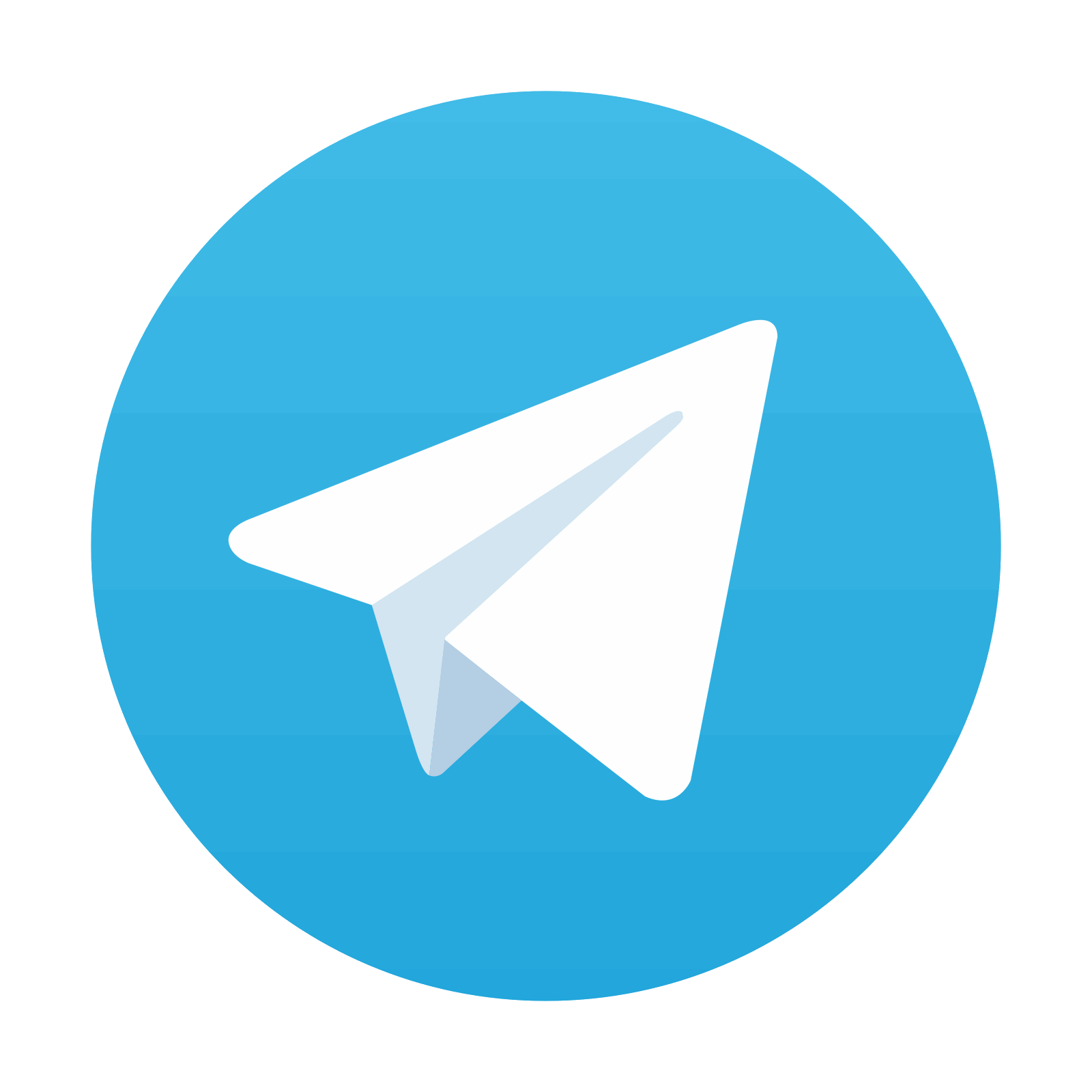
Stay updated, free articles. Join our Telegram channel

Full access? Get Clinical Tree
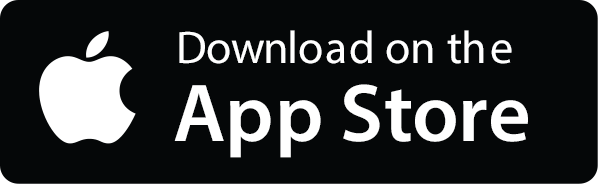
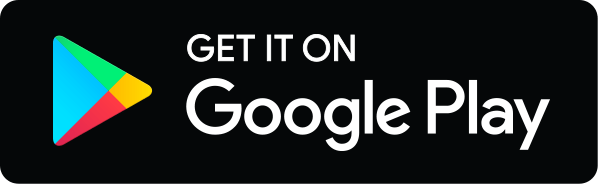