Fig. 1
The concept of esophageal high-resolution manometry (HRM). In contrast to conventional manometry, HRM requires multiple sensors 1 cm apart on an esophageal motility catheter (a) that generate multiple recordings of pressure phenomena from each individual sensor throughout the esophagus (b). The space between recording sensors is filled with the best-fit data using dedicated computer software, which also color codes amplitude levels (c). Finally, the image is smoothed out electronically, and displayed as a topographic contour plot representing the peristaltic sequence when viewed from above (d). The contour plots are termed Clouse plots in honor of Ray Clouse, who conceived and developed HRM. (Reproduced with permission from [8])
Conventional Esophageal Manometry
In 1977, Dodds and Arndorfer created the first high-fidelity manometry system. This system consisted of a bundle of thin flexible tubes, each with a single small “side-hole” perpendicular to the esophageal lumen, spaced 3–5 cm apart along the catheter. A pneumo-hydraulic pump connected to the catheter pumped gas-free water into each tube and out each side-hole. This column of water transmitted esophageal luminal pressure to a transducer, which converted the measured pressure into an electrical signal displayed as a wave form [4]. This low-compliance water-perfused catheter system increased accuracy of pressure measurements while relatively durable and inexpensive.
An ideal manometry system should collect pressure measurements continuously and concentrically, accounting for all esophageal motor activity and pressure fluctuations along the asymmetrical esophageal lumen and sphincters. In the earliest conventional manometry system, however, the pressure sensors were spaced several centimeters apart and were unidirectional, leading to inaccuracies in recorded pressure data [1, 2, 4]. For instance, the radial asymmetry of the sphincters led to inaccurate pressure measurements from the unidirectional pressure sensors. Further, the tubular esophagus shortens relative to the catheter during swallowing, and the sensor designated to record the lower esophageal sphincter (LES) pressure may no longer remain within this sphincter. To better assess LES pressure measurements, a 6 cm perfused sleeve sensor (known as the “Dent sleeve”) was devised, positioned at the distal end of the catheter to provide continuous pressure within the LES [5]. Finally, the localization of the sphincters, especially the LES, required a “stationary pull through maneuver,” which consisted of pulling the catheter back 1 cm at a time, observing typical sphincteric pressure profiles, and localizing the LES relative to the nostril so that the catheter could be maneuvered into place for the actual manometry study. This process was cumbersome for the operator and unpleasant for the patient.
Since water-perfused manometry was labor intensive, fraught with artifacts, and required technical expertise for data acquisition and interpretation, water-perfused sensors were subsequently replaced with solid-state pressure transducers [1, 2, 6]. Solid-state manometry used strain gauge transducers placed along the catheter at regular intervals and measured pressure in a unidirectional fashion or averaged pressure around the circumference of the transducer. The development of a solid-state manometry system improved the accuracy of assessment of esophageal peristalsis and sphincter function, but the systems were fragile and expensive [1]. Further, the sensors remained widely spaced, and the problems with incomplete assessment of the pressure continuum persisted.
Esophageal High-Resolution Manometry (HRM)
In the early 1990s, Ray Clouse and colleagues began investigating how best to circumvent the limitations of conventional manometry. Clouse believed that pressure events between the widely spaced conventional manometry sensors could provide additional clues to esophageal motor function in health and disease. Innovative and pioneering research addressing these limitations led to the concept and development of HRM and esophageal pressure topography (EPT) [3, 7]. Clouse first performed pull-through maneuvers 1 cm at a time with water-perfused catheters, charting pressure events along the esophagus. He then digitized the pressure recordings, filled space between the 1 cm recordings with best-fit data, and assigned colors to different pressure levels. This allowed 3-D display of the peristaltic sequence, colors indicating pressure amplitudes, and the x- and y-axes indicating distance along the esophagus and time, respectively—images generated are now termed Clouse plots [8]. Using these concepts, Clouse initially created a system with 21 water-perfused pressure sensors located every centimeter along the catheter [7]. Using data collected from these sensors, the EPT plots were created with dedicated computer software. This representation of continuous esophageal peristalsis, sphincter location, and pressure is similar to that of geographic topographic maps. Today, the HRM can be performed using water-perfused or solid-state systems. The commercially available systems utilize 32–36 circumferential solid-state sensors spaced at 1 cm intervals (Fig. 1).
The HRM using EPT has considerable theoretical and practical advantages over conventional manometry , allowing for more consistent and accurate assessment of esophageal function and dysfunction [2, 9]. In practice, compared to conventional manometry, the HRM is relatively simple and fast for both the operator and the patient [10, 11]. The stationary pull-through maneuver is obsolete as the pressure profile of the esophagus can be visualized in real time immediately upon catheter placement; this ensures that the catheter is placed correctly and that it traverses both the upper esophageal sphincter (UES) and the LES. The close spacing between pressure sensors ensures that the HRM generates dynamic and detailed depiction of pressure phenomena along the tubular esophagus and sphincters [2]. Many esophageal motor abnormalities and disorders create distinct and recognizable HRM and EPT patterns, easily identified even by novice and trainee esophagologists in many instances [12]. Pattern recognition with HRM and EPT plots has allowed for improved inter-observer agreement amongst interpreters, providing a user-friendly interface for teaching trainees with better diagnostic accuracy compared to the conventional line tracings [12−14].
The advent and use of HRM and EPT plots has contributed to better understanding of the esophageal phase of deglutition. The accuracy of assessment of abnormal LES function has been impacted the most, as the LES can now be followed proximally with esophageal shortening during swallows—with conventional manometry, this could have created an appearance of “pseudo-relaxation” of the LES as the sphincter shortened proximal to the LES sensor [15]. Prior to HRM, the concept of two smooth muscle contraction segments in the esophageal body was not established [7]. The behavior of the smooth muscle contraction segments on EPT defines motor abnormalities at both ends of the motor disorders spectrum; hypermotility and hypomotility. Finally, HRM has allowed uniformity in data reporting and interpretation, as software tools can be utilized to assess and report motor phenomenon [16].
Indications for Esophageal HRM
Esophageal manometry has multiple indications in the evaluation of esophageal motor function. While some of the same indications as for conventional manometry apply for HRM, additional detail can be obtained with this technique, providing for gains in diagnostic accuracy. The traditional indications for manometry include diagnosis of obstructive motor disorders (e.g., achalasia) in the setting of dysphagia without a structural etiology on endoscopy or barium esophagogram, localizing the proximal margin of the LES for placement of pH probes, and assessing the adequacy of esophageal body peristalsis [1, 2, 16]. While HRM fulfills these indications efficiently, additional detail is obtained regarding the structure, integrity, and function of the esophageal sphincter. Further, esophageal body motor patterns can be better defined, and motor patterns hitherto unknown have been described (e.g., jackhammer esophagus, premature contraction sequences, fragmented contraction sequences) that may explain both obstructive transit symptoms as well as perceptive symptoms from esophageal hypervigilance associated with certain motor patterns [17−20]. Evaluation of unexplained noncardiac chest pain and post-fundoplication dysphagia has been further refined with HRM. While evaluation of the UES was suboptimal with conventional manometry, HRM has shown potential in identifying the UES dysfunction. The superimposition of impedance with HRM (high-resolution impedance manometry , HRIM) allows assessment of bolus transit with liquid, viscous, and solid boluses—while this has potential for additive benefit, outcome studies are unavailable to date and research continues [21]. Finally, a newer HRM system with a short segment of even closer sensor distribution (3-D manometry) may provide further sphincteric detail [22].
Abnormal or dysfunctional esophageal motility can cause esophageal dysphagia. Therefore, HRM is indicated in the diagnostic workup of esophageal dysphagia after endoscopy or barium esophagography has excluded more common structural defects (such as a stricture, ring, mucosal inflammation, eosinophilic esophagitis), or when a motor disorder is suspected after initial testing. Clinical utility of HRM is better when the pretest probability of a motor disorder is high, as nonspecific abnormalities on manometry studies may not correlate well with symptoms [23, 24]. Achalasia and advanced spastic disorders (such as diffuse esophageal spasm), on the other hand, correlate with symptoms such as dysphagia and regurgitation from abnormal bolus transit and are easily recognized on HRM [25]. HRM may also have the utility in situations where multisystemic disease processes affecting the smooth muscle or the enteric nervous system, such as scleroderma where striated muscle function is normal in the proximal esophagus , but peristalsis is diminished or absent in the smooth muscle esophagus with decreased or absent LES pressure [26, 27]. Finally, there is evidence that HRM findings may help stratify patients being considered for antireflux surgery (i.e., fundoplication) [28]. In this setting, important outcomes from HRM are the identification of esophageal outflow obstruction, which contraindicates fundoplication and esophageal aperistalsis without outflow obstruction, which necessitates a partial rather than a standard fundoplication [28, 29]. Provocative maneuvers such as multiple rapid swallows (MRS) may help assess esophageal peristaltic reserve in settings where peristalsis is hypomotile [29].
Acquisition and Interpretation of HRM
Patients scheduled for HRM should be fasting for at least 6 h prior to the procedure, and medications that affect gut motility are typically withheld [1, 2]. Once the HRM catheter is disinfected and calibrated, it is inserted transnasally with the operator directly visualizing pressure patterns to ensure proper catheter placement. Two bands of pressure generated by the UES and LES respectively anchor the HRM Clouse plot at either extent, and the operator learns to recognize these to confirm that the catheter tip is in the stomach [2]. The point of pressure inversion with respiration can ascertain that the diaphragmatic crura have been traversed in most instances—this is distal to the LES high-pressure zone when a hiatus hernia is present. Once catheter placement is appropriate, the patient undergoes a standard manometry protocol in the supine position, consisting of a 30 s baseline recording period without swallowing, followed by a series of ten 5 ml water swallows (delivered by a syringe into the patient’s mouth) every 20–30 s. The interval between swallows allows for the esophageal motor function to return to the baseline [2]. Viscous and solid boluses can be administered if desired. Finally, provocative maneuvers can be performed. The simplest of these consists of administering five small (2 mL) water boluses in rapid succession 2–4 s apart, termed multiple rapid swallows (MRS) [2]. This provocative maneuver tests esophageal neural connections responsible for both deglutitive inhibition (absence of esophageal smooth muscle peristalsis and profound relaxation of LES during MRS), and esophageal contractile reserve (robust esophageal body contraction and reestablishment of LES tone following deglutitive inhibition) [29, 30]. Deglutitive inhibition is often impaired in disorders with inhibitory nerve dysfunction such as achalasia and esophageal spasm [31, 32], while post-MRS contraction is impaired in hypomotility disorders [29].
Interpretation of EPT plots starts with identification of esophageal sphincters. The UES and LES are easily identified on EPT plots as bands of abrupt pressure increase at proximal and distal extents of the esophagus (Fig. 2) . The esophagogastric junction (EGJ) is superimposed on the LES high-pressure zone under normal circumstances [2, 16]. The location of diaphragmatic crura can be further ascertained by asking the patient to take a deep breath when inspiratory contraction of the crural diaphragm can be visualized. However, separation of the LES from the crural diaphragm defines an axial hiatus hernia.
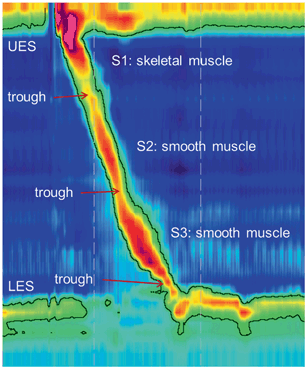
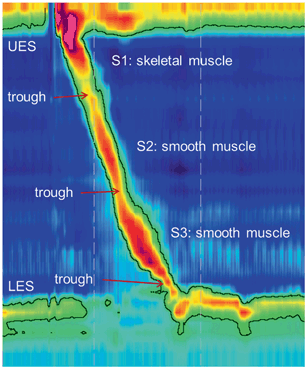
Fig. 2
Normal high-resolution manometry Clouse plot. The plot is anchored by two pressure bands, the upper esophageal sphincter (UES) and the lower esophageal sphincter (LES). The pressure in the sphincters dissipates to designate relaxation in conjunction with a peristaltic sequence. Smooth muscle contraction segments consist of a proximal striated muscle segment (S1), followed by two smooth muscle segments (S2, S3). Sphincter relaxations and contractions of the three peristaltic segments occur in smooth continuity with normal esophageal peristalsis
Integrity of esophageal peristalsis is then assessed. During a normal wet swallow, the EPT plot will display pharyngeal contraction and UES relaxation, followed by the progressive and sequential esophageal body contraction in the three esophageal segments (Fig. 2). Software tools such as the isobaric pressure contour can be used to assess peristaltic integrity, typically with the tool set at a threshold of 20 mmHg [2, 16]. A distinct pressure trough between the first and the second segments, the transition zone, identifies transition of peristalsis from striated to smooth muscle, and consequently, from central nervous system control to the enteric nervous system [33, 34]. The LES relaxes promptly upon initiation of the swallow as visualized by UES relaxation, and demonstrates after-contraction while regaining resting tone as the peristaltic wave terminates in the distal esophagus .
The most important aspect of interpretation of HRM Clouse plots is the determination of adequate LES relaxation with swallows. Integrated relaxation pressure (IRP) is the universally accepted metric for assessing this, and represents four continuous or discontinuous seconds of nadir pressure during LES relaxation [2, 16]. The IRP, therefore, interprets resistance to bolus flow through the EGJ and is determined by both hydrostatic pressure generated from the esophageal peristalsis and outflow resistance at the EGJ. The threshold of normal has been determined to be 15 mmHg. The classic condition with an elevated IRP, typically in the face of absent or nonpropulsive esophageal peristalsis, is achalasia (Fig. 3). The IRP may also be elevated by any process that increases outflow resistance (such as infiltration or inflammation of the EGJ, or an anatomic process such as a stricture). Determination of the presence or absence of esophageal outflow obstruction using the IRP is the first interpretative element of HRM analysis, since outflow obstruction is the most treatable of esophageal motor disorders [2, 16].
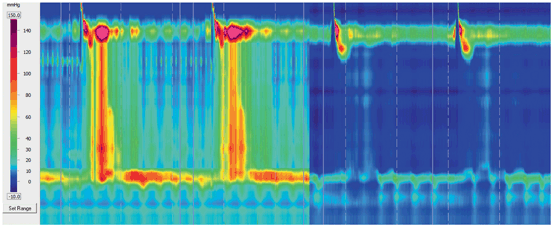
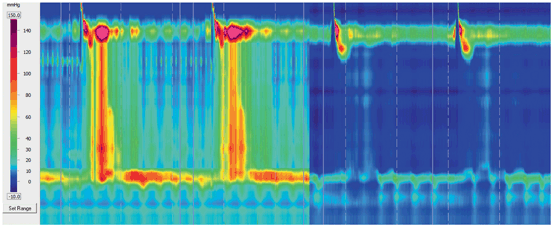
Fig. 3
Examples of HRM Clouse plots in achalasia and scleroderma esophagus. The left panel demonstrates aperistalsis and panesophageal compartmentalization of intrabolus pressure between the UES and the nonrelaxing LES with each swallow, features characteristic of achalasia. Because of the panesophageal compartmentalization of pressure, this pattern designates the achalasia as type II. The right panel also demonstrates aperistalsis, but in this instance, the skeletal muscle contraction segment is intact, the LES is hypotensive, and relaxes with each swallow. This pattern, if consistent throughout the study, suggests esophageal smooth muscle dysfunction. The combination of aperistalsis and a hypotensive but relaxing LES is termed scleroderma esophagus , despite the fact that scleroderma may not be the etiology in the majority of instances. These examples demonstrate the intuitive and visually descriptive nature of HRM. HRM high-resolution manometry, UES upper esophageal sphincter, LES lower esophageal sphincter
The HRM Clouse plots allow better characterization of esophageal body motor function. Characteristics evaluated include the proportion of wet swallows that result in peristaltic sequences, the velocity of transmission, and certain characteristics of the contraction sequence. Bolus transport is abnormal when a wet swallow does not result in a peristaltic sequence, either with partial or complete failure of esophageal contraction, or with simultaneous nonpropulsive contraction [35]. The velocity of the peristaltic transmission varies throughout the esophagus . Pressure waves travel about 3 cm/s in the upper-esophageal body, 5 cm/s in the mid-esophageal body, and 2.5 cm/s in the distal-esophageal body [36]. In general, a velocity greater than 6 cm/s is abnormal and may cause ineffective bolus transit [37]. The amplitude of the pressure wave is defined as the difference between the baseline resting pressure and the highest pressure during peristalsis [1]. Normal pressure wave amplitude ranges between 30 and 180 mmHg. Pressure waves with amplitudes < 20 mmHg are not classified as being produced by a contraction; amplitudes consistently < 30 mmHg are generally described as hypotensive; while averaged amplitudes > 180 mmHg are generally described as hypertensive [35, 38, 39].
Software tools are also available for interrogation of esophageal body peristalsis. The vigor of peristalsis in the smooth muscle esophagus is addressed by the distal contractile integral (DCI), which takes into account the length, amplitude, and duration of smooth muscle contraction, expressed as mmHg/cm/sec [2]. Single-swallow DCI values above 8000 mmHg/cm/sec, and averaged values above 5000 mmHg/cm/sec are almost never encountered in asymptomatic adults; these thresholds define hypercontractile disorders. However, the DCI provides a broad assessment of smooth muscle contraction rather than specific details, and further inspection of contraction segments may provide additional contraction detail that may explain esophageal symptoms [2, 16]. For instance, contraction wave abnormalities (such as multiple peaked waves, elevated distal contraction amplitudes > 180 mmHg, and intermittently prolonged wave duration) may not necessarily result in an abnormal DCI [40]. Distal latency (DL) is another HRM metric that defines timing of esophageal peristalsis, measured as the time from UES relaxation to the transition point between fast proximal and slower distal peristaltic propagation speeds (termed contractile deceleration point or CDP). Values < 4.5 s define the premature arrival of esophageal peristalsis in the distal esophagus, segregating sequences that may not propel boluses [2, 16]. The speed of esophageal body peristalsis is further assessed as contraction front velocity, and values > 9 cm/s identify simultaneous esophageal peristalsis, and diffuse esophageal spasm.
For establishing uniformity in interpretation of HRM and EPT plots for nonobstructive dysphagia, the Chicago Classification scheme was created [16]. This employs a 3-step approach to classify HRM and EPT findings that consist of: (1) assessing the EGJ anatomy and function by examining the EGJ morphology and basal pressure, and calculating the IRP; (2) assessing esophageal body function by evaluating the DCI and peristaltic integrity; and (3) assessing pressurization patterns. This diagnostic algorithm classifies HRM and EPT findings into four groups: (1) achalasia (further subdivided into subgroups as described below), (2) EGJ outflow obstruction, (3) abnormal motility disorders not observed in normal subjects (i.e., distal esophageal spasm, hypercontractile esophagus , and absent peristalsis), and (4) borderline motility with abnormalities not within the range of normal values (i.e., weak peristalsis, frequently failed peristalsis, rapid contractions with normal latency, and hypertensive peristalsis) [16].
Achalasia, defined as aperistalsis and incomplete LES relaxation, creates a distinct pattern that is easily identified on EPT plots (Fig. 3). Achalasia results from death or significant dysfunction of the inhibitory nerves, typically from idiopathic inflammation of inhibitory neurons in susceptible individuals. The Chicago Classification categorizes achalasia into three subgroups, types I–III, with each type representing a distinct phenotype and response to the treatment [41]. In this scheme, the threshold for the diagnosis includes esophageal outflow obstruction with IRP > 15 mmHg. Achalasia subtypes are defined as follows: Type I achalasia (i.e., “classic achalasia”) with 100 % failed peristalsis; type II achalasia (i.e., “achalasia with esophageal compression”) has no normal peristalsis and panesophageal pressurization with at least 20 % of swallows; and type III achalasia has no normal peristalsis with preserved spastic fragments of distal peristalsis or premature contractions with at least 20 % of swallows [16]. Available first-line treatments for achalasia include pneumatic dilation or surgical myotomy, which disrupt the nonrelaxing LES and thereby resolve esophageal outflow obstruction [42]. Type I achalasia patients are reported to respond better to Heller myotomy compared to pneumatic dilation, type II patients respond fairly well to all acceptable treatments, and type III patients respond incompletely to therapy mainly from a persistent perceptive element related to partially retained spastic contraction [11, 43, 41]. Peroral endoscopy myotomy (POEM) is a relatively new minimally invasive option that is reported to adequately resolve esophageal outflow obstruction in achalasia [44].
Esophageal body motor dysfunction generally falls along the lines of hypomotility (poor contraction, breaks in peristaltic integrity, fragmentation of peristalsis, absent peristalsis) and hypermotility (exaggerated contraction, simultaneous peristalsis) based on pathophysiological mechanisms that correspond with easily recognizable EPT patterns [18, 45]. Hypomotility processes can affect the LES and/or the esophageal body, and can result from either poor excitatory mechanisms or failure of the smooth muscle to respond to excitatory stimuli. Hypomotility patterns are frequently associated with poor esophageal clearance and suboptimal barrier function at the EGJ, associated mainly with gastroesophageal reflux disease (GERD) patterns. Hypermotility patterns manifest aberrant inhibitory nerve function, exaggerated excitatory function, or combinations thereof, and can be associated with esophageal hypervigilance and perceptive “hypersensitivity” symptoms [19].
pH- and pH-Impedance Monitoring
A trial of empiric proton-pump inhibitor (PPI) therapy is considered the standard of care for initial evaluation and management of patients with suspected GERD symptoms, reserving endoscopic evaluation for alarm situations or lack of response to this empiric approach. However, certain situations necessitate further diagnostic evaluation for either establishing the presence of abnormal esophageal acid exposure in explaining esophageal symptoms, or for ruling out a reflux mechanism for symptoms [46, 47]. Evaluation is typically performed using ambulatory pH monitoring, with or without concurrent impedance monitoring. Indications for pH testing include symptomatic states that could be consistent with GERD, but refractory to seemingly adequate PPI therapy, and when aggressive treatment options such as antireflux surgery are being considered. Currently, the ambulatory pH monitoring options include conventional catheter-based pH monitoring with two pH sensors 15 cm apart, and wireless pH monitoring using a single sensor pH probe that can be attached to the esophageal mucosa [46, 47]. Multichannel intraluminal impedance monitoring can assess bolus movement along the tubular esophagus and can complement pH monitoring. This consists of the measurement of resistance (impedance) to flow of a tiny electrical current, generated by esophageal tissues or intraluminal bolus within the esophagus. Resistance (impedance) decreases below the resting baseline with liquid content adjacent electrode pairs along the impedance catheter, and increases with air in the lumen. The multiple electrode pairs placed along the catheter allow assessment of directionality of impedance change, and hence direction of bolus movement along the esophagus. This technology can be combined with ambulatory pH monitoring to better characterize reflux events (Fig. 4), or with stationary HRM to define the relationship between esophageal peristaltic patterns and bolus clearance [46−48].
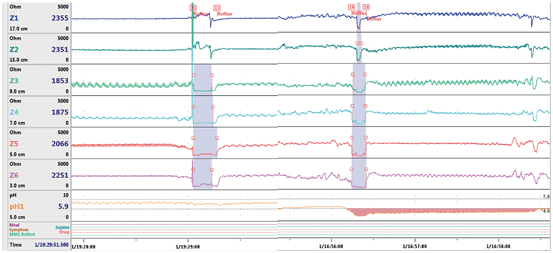
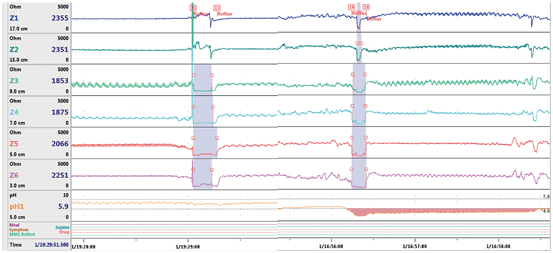
Fig. 4
Esophageal pH and impedance tracing showing a reflux event. The first event does not demonstrate a drop in pH, and therefore, represents a weakly acidic or nonacidic reflux event. The drop in pH associated with the second event indicates that the reflux event is acidic. Decrease in esophageal impedance is seen moving retrograde up the esophagus, indicating that these episodes represent reflux and not swallowed boluses
Data Acquisition and Analysis
Ambulatory pH monitoring documents the exposure to acid mainly in the distal esophagus . This can be performed using a transnasal catheter-based system consisting of two recording sensors 15 cm apart or a wireless system with a single sensor; both systems require a data recorder worn by the patient. With the catheter-based systems, the reflux events are monitored over a 24 h period while the patient keeps a diary of activities and meals. By convention, the distal pH recording sensor on catheter-based devices is placed 5 cm above the proximal LES margin defined by manometry [46−48]. Wireless pH probes record acid exposure for 48–96 h, and are placed 6 cm above the measured squamocolumnar junction, which corresponds to the location of the distal pH sensor on catheter-based systems [49]. pH drops below a threshold of 4.0 define reflux events. The duration for which pH is < 4.0 can be quantified, typically reported as the percentage of time this threshold is crossed over a day (acid exposure time, AET). Normal thresholds are typically 4 % for total AET, 6 % while upright, and 2 % while supine. Correlation of symptom episodes to reflux events can be assessed. Patients record symptoms electronically using buttons on their recording device; symptoms occurring within 2 min of reflux events are extracted and considered correlated to reflux events [46]. A simple proportion of correlated symptoms to all symptoms define the symptom index (SI). A more sophisticated measure is the symptom association probability (SAP), which statistically assesses the probability of chance association between symptoms and reflux events—chance association < 5 % defines strong confidence in symptom reflux association, which corresponds to a p value of < 0.05 on this test, or SAP > 95 % [50]. Increasing ambulatory pH monitoring from 24 to 48 h has been shown to increase the diagnostic yield of the study, especially in bringing out day to day variation in acid exposure times, and assessing symptom reflux association for infrequent symptoms, particularly atypical symptoms [51−54]. Limited data exist on even longer pH monitoring, up to 96 h, with further augmentation of diagnostic yield. The 96 h study can sometimes be used to assess response to acid suppression, when the first half of the study is performed off antisecretory therapy and the second half on therapy [55].
< div class='tao-gold-member'>
Only gold members can continue reading. Log In or Register a > to continue
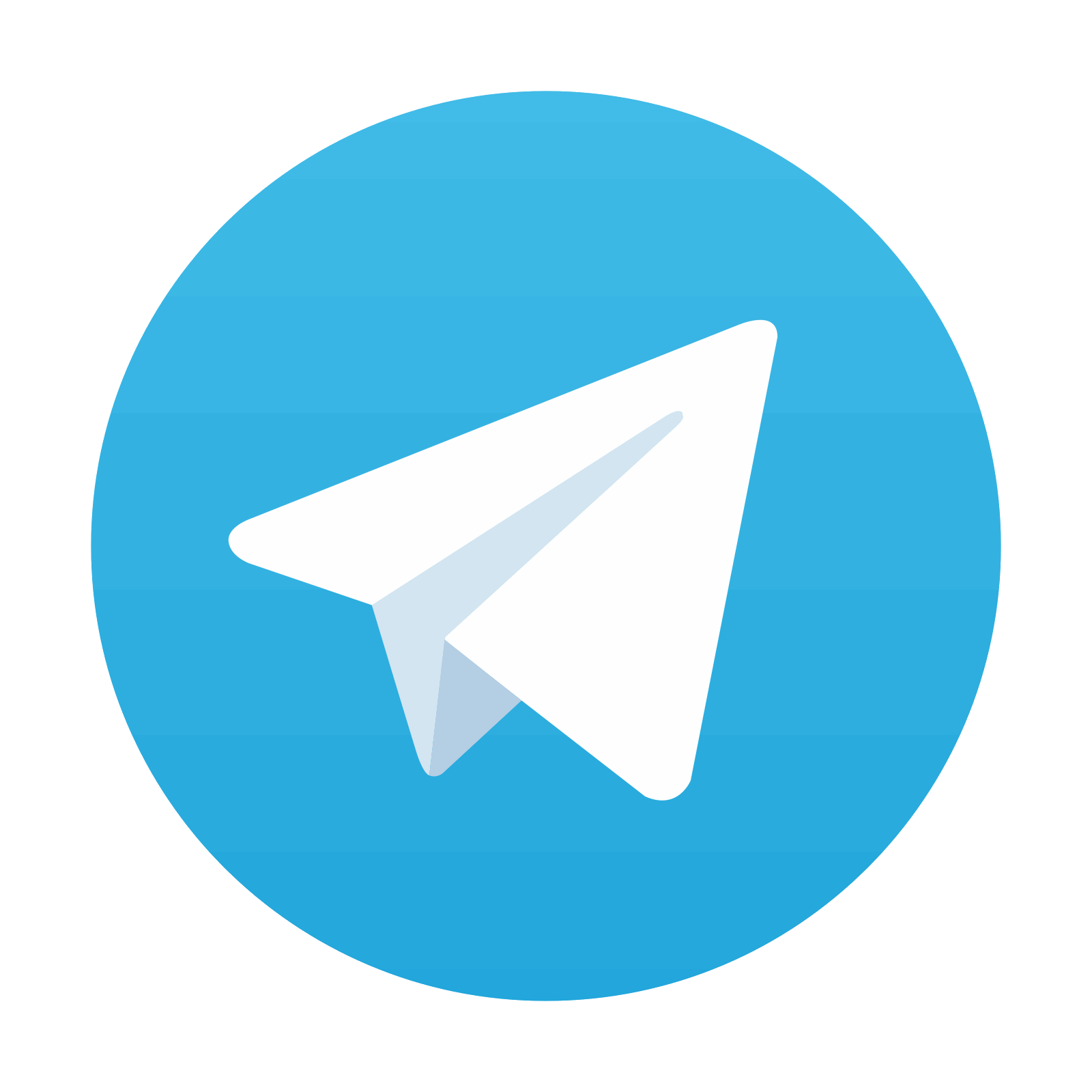
Stay updated, free articles. Join our Telegram channel

Full access? Get Clinical Tree
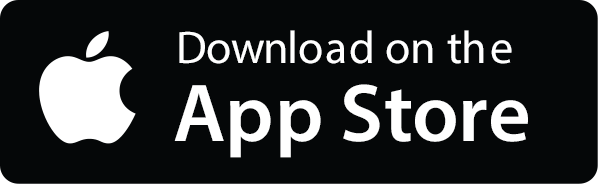
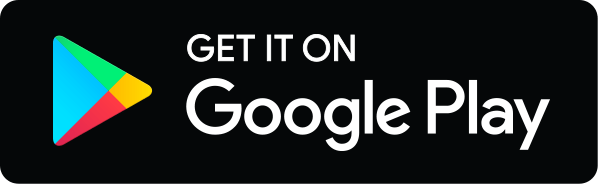