Understand the complexity and lack of standardization related to the diagnosis and treatment of posttraumatic long bone osteomyelitis
Provide guidance and treatment options when faced with a patient who requires workup and management of segmental defect
Highlight this chapter with a case example with a special emphasis on pearls and pitfalls.
Of note, the case example used in this chapter was previously published and utilized in an open access article: Chadayammuri et al. Patient Safety in Surgery (2015) 9:32 DOI 10.1186/s13037-015-0079-0 © 2015 Chadayammuri et al.
Osteomyelitis, or infection of the bone, represents a challenging clinical entity in the field of orthopedics. In 1951, Gallie et al reported a case of recurring osteomyelitis following a period of 80 years since onset of initial infection. The patient was a 90-year-old woman with a Brodie abscess localized to the distal femur. Given a largely asymptomatic presentation throughout the patient’s lifetime, diagnosis and treatment were exceedingly delayed. This case is but one of many that illustrates the complex nature of osteomyelitis.1
Two primary variants of osteomyelitis have been previously characterized: hematogenous and long bone posttraumatic osteomyelitis (PTOM). Classification may be further divided according to duration (Waldvogel et al) or disease stage (Cierny-Mader et al).2 In the Waldvogel classification, osteomyelitis is classified on the basis of being hematogenous, contiguous, or chronic in nature. In the Cierny-Mader classification, osteomyelitis is categorized by anatomic location into stage 1 (medullary), stage 2 (superficial), stage 3 (localized), and stage 4 (diffuse). The latter classification scheme also incorporates consideration of the host’s health status, divided into local factors (chronic lymphedema, venous stasis, arteritis, etc) or systemic factors (malnutrition, renal failure, diabetes mellitus, immunodeficiency status, etc). While these classification systems can be useful for diagnosis and treatment planning, they have shortcomings. The location within the bone (periarticular versus diaphyseal) is neglected in the Cierny-Mader classification, which has an impact on treatment options and outcome. Classifying the anatomical stage can also be difficult when hardware is present because MRI is often not useful due to interference.
The focus of the remainder of this chapter will be on long bone posttraumatic osteomyelitis (PTOM), defined as infection of the bone in conjunction with recent fracture or traumatic insult. Long bone PTOM is a frequent occurrence and may be involved in as many as 10% of all open fractures and 1% of all closed fractures.3 Several etiological factors have been previously described, including direct inoculation at time of injury, macrovascular or microvascular damage, surgical contamination, host immunodeficiency, and/or postoperative wound contamination.4-6 Importantly, untreated long bone PTOM may progress to fracture nonunion, sepsis, and ultimately, limb amputation.5
The clinical diagnosis of long bone PTOM is challenging, in large measure, owing to the nonspecific nature of its initial presentation. In addition to findings of localized pain, long bone PTOM classically presents with signs and symptoms of infection including low-grade fever, erythema, edema, and/or draining sinus tracts.6,7 In pediatric patients, presentation may also include systemic manifestations such as fever, chills, and night sweats.6 Clinical examination should reveal localized tenderness to palpation overlying an aspect of bone with prior or current fracture.
Diagnostic workup of long bone PTOM traditionally involves a combination of imaging, tissue culture, and laboratory studies.8 Acute inflammatory markers such as erythrocyte sedimentation rate (ESR), C-reactive protein (CRP), and leukocyte count (WBC) have low specificity for diagnosis, particularly in the setting of a profound systemic inflammatory response (eg, rheumatoid arthritis, Crohn’s disease, systemic lupus erythematosus). Therefore, trending levels of acute inflammatory markers are more appropriately reserved for monitoring infection status following initiation of treatment.6,7 The “gold standard” for the diagnosis of PTOM is a bone culture obtained in the operating room; however, this should always be complemented by histopathological analysis to reduce the incidence of false positives.8,9 Currently ongoing clinical studies are investigating newer technologies such as polymerase chain reaction (PCR) and fluorescence in situ hybridization (FISH) that may improve diagnostic sensitivity; however, additional research is required to inform the feasibility and validity of such modalities.9,10
Following positive findings on culture and pathology suggestive of long bone PTOM, preoperative workup must include an imaging series consisting of anteroposterior (AP) and lateral radiography, magnetic resonance imaging (MRI), and/or computed tomography (CT). A multimodal imaging approach is employed to surmount the independent limitations of each imaging modality. In patients with long bone PTOM, AP and lateral radiographs traditionally demonstrate regional osteopenia, periosteal reaction (“Codman’s triangle”), and sequestrum (segments of necrotic bone with interspersed viable granulation tissue). However, sensitivity of plain radiography to detect infection is rather poor in the initial 2 weeks of infection.6 CT may enable earlier detection of infection through visualization of devitalized cortical bone, sequestrum, and/or involucrum (periosteal bone formation) on multiplanar reconstructions but suffers poor capacity to delineate soft-tissue involvement.11 In contrast, MRI enables excellent visualization of soft-tissue pathology in as early as 3 to 5 days following initial onset of long bone PTOM.11 For this reason, MRI is considered the “gold standard” for confirmation of osteomyelitis infection via imaging studies. Exudate, edema, or sequestrum appears as hypodense lesions on T1-weighted MRI images and short-tau inversion recovery (STIR) sequences, while surrounding granulation tissue appears as a low-intensity signal on T1-weighted images and high-intensity signal on STIR sequences or T2-weighted images.11
The management of long bone PTOM is complex and challenging. Therefore, patients must be prepared for a long course of multiple surgeries and counseled on the risks for postoperative complications that include nonunion, hardware failure, and infection recurrence. Moreover, initial stages of preoperative planning must involve correction of modifiable comorbidities and/or risk factors that portend poor postoperative wound healing. All patients with long bone PTOM should be evaluated for poor glycemic control (in diabetic patients), tobacco or illicit drug use, malnutrition, and vascular insufficiency of the affected limb.12 Low socioeconomic status (SES) is also a prognostic indicator of worse treatment outcome,13 a fact that should not deter the provision of care but rather alert the treating surgeon to the increased potential for postoperative complications.
TIP: Order Nutritional and Metabolic Labs in the Preoperative Workup of Patients with Long Bone PTOM
A landmark study demonstrated that 31 of 37 patients (83%) presenting with unexplained fracture nonunion in spite of adequate reduction and stabilization were found to have one or more underlying metabolic or endocrine abnormalities such as vitamin D deficiency, calcium imbalance, central hypogonadism, thyroid disorders, and parathyroid hormone disorders. Eight of these patients (25%) achieved bony union in an average of 7.6 months (range, 3 to 12 months) following treatment of their metabolic or endocrine abnormalities without further operative treatment.14
In severe cases of long bone PTOM, debridement of the infected tissue results in extended diaphyseal loss of bone that cannot be adequately managed by conventional methods of reconstruction. In particular, conventional methods often fail to satisfy at least one of the following goals of therapy:
control of the local infection with radical debridement and antibiotic therapy
fracture stabilization when instability occurs due to debridement or nonunion
provision of adequate soft-tissue coverage to ensure wound healing3,6
Radical debridement may further precipitate widening of the osseous defect in cases where segmental bone defects exceed 5 cm in size.15 Conventional techniques such as vascularized fibula autograft and Ilizarov bone transport also yield poor long-term outcome, often due to graft resorption and revascularization by creeping substitution.16,17 Bone regeneration may also be impeded secondary to inadequate vascularization or soft-tissue coverage.18
The Masquelet technique represents a 2-staged reconstructive procedure that overcomes several of the shortcomings in the treatment of osteomyelitis defects, particularly those located to the long bone and associated with infected and/or nonviable soft tissue.19 First developed in the late 1970s by AC Masquelet but only recently popularized, the chief advantages of this strategy include control of the local infection with radical debridement, placement of a polymethyl methacrylate (PMMA) cement spacer for maintenance of dead space, and induction of a periosteal membrane that protects against graft resorption. Furthermore, the Masquelet technique is length-independent and is therefore a viable option for the treatment of larger osseous defects. A detailed description of the Masquelet technique with “Tips and Tricks” and an illustrative case example are provided in the following sections.
Clinical Example: Two-Staged Reconstruction of Extended Diaphyseal Bone Defects Using the Masquelet Technique
The Masquelet technique is performed in 2 stages. In the setting of an unstable long bone with infected and/or nonviable soft tissue, the first stage involves radical debridement of all infected or nonviable bone and interposed fibrous tissue. Given that devitalized tissue serves as a nidus for recurrent infection and predisposes to increased risk of postoperative complications such as delayed union, nonunion, and vascular thrombosis, reconstruction of the bone defect (conducted in stage 2) is only possible once complete eradication of infected and nonviable tissues has been achieved.
TIP: Margins of Debridement
The margins of debridement should extend until viable bony edges are encountered, determined intraoperatively using the “paprika sign” (punctate bleeding upon drilling with a 2.5 mm drill bit). Once the margins of debridement have been determined, an osteotome can be utilized to perform a corticotomy in order to prevent destruction of healthy surrounding tissues.
Following debridement, stabilization must be achieved to maintain length, alignment, and rotation prior to insertion of the cement spacer. Options include unilateral or ring external fixation, plate osteosynthesis, or IM nailing. The choice of stabilization is based on the location of the defect. For bone loss in the middiaphysis, an IM nail offers stable fixation that allows early weight bearing and avoids the complications associated with external fixation, such as pin site infection. A narrow-diameter nail is used and coated with antibiotic-impregnated cement. For defects close to an articular surface, external fixation is preferred. Ring fixators offer stable fixation and the ability to modify the bony alignment postoperatively. When placing external fixation, care must be taken to keep pins away from the site of definitive fixation so that the external fixator can be left in place until healing has been achieved (during stage 2 of the procedure).18
In our experience, the preparation of an antibiotic IM interlocking carbon-fiber nail can be achieved using a simple and reproducible technique.20 Depending on the clinical situation and bone involved, plastic tubing of various internal diameters including chest tubes, D&C tubing, and D&E tubing can be used as a mold. We recommend creating a cement mantle of at least 2 mm to prevent delamination of the cement from the nail if removal becomes necessary. The tubing is cut to a length such that the proximal threaded portion of the nail is left free of cement. The inner portion of the tube is coated with sterile mineral oil to facilitate extraction of the nail. One end of the tubing is then clamped with a Kocher while the other end is loaded with the viscous cement-antibiotic preparation using a cement gun. We traditionally use 3 g of vancomycin powder combined with 40 g of Palacos-R (Zimmer Biomet; Warsaw, Indiana) PMMA cement. When polymicrobial of gram-negative infection is suspected or demonstrated by bone cultures, 3.6 g of tobramycin can be added to the mix as well. An extra 10 to 20 cc of monomer should be used to obtain injectable cement, and mixing should be performed under a vacuum to improve the antibiotic elution profile.21 A standard 8 or 9 mm IM nail is then inserted centrally into the chest tube to produce a wide cement mantle with a consistent thickness. The entire construct should then be placed into a cool sterile saline bath during the exothermic polymerization process to prevent melting of the plastic tube and facilitate removal of the nail.22 We allow the interlocking holes to be covered with cement. Once hardened, the chest tube is cut longitudinally and peeled off the cement-coated nail. The distal end of the cement mantle can be contoured with a rasp to allow for easier insertion. The intramedullary canal is then prepared in a standard fashion for nail insertion. The cement-coated nail is wider than standard nails so aggressive reaming is often necessary. If there is concern for proximal or distal spread of the infection in the canal, we use the reamer/irrigator/aspirator (RIA) system as a means of further debridement. The IM nail is inserted and statically locked under fluoroscopic guidance, with proper alignment confirmed by plain radiography.
TIP: Care When Drilling
Care should be taken when drilling through the cement mantle, as high force is generated that can potentially damage or break the drill bit.
The next step following stabilization is to fashion a cement spacer to fill the segmental bone defect, traditionally composed of PMMA cement. The mixing of antibiotic with the cement spacer is controversial. Masquelet does not typically use antibiotics in his spacers because this may suppress a low-grade residual infection and increase the risk of recurrence after stage 2 is complete. Instead, he relies on a thorough debridement and systemic antibiotics to clear the infection. Antibiotic-impregnated cement creates a local environment with a very high concentration of antibiotics that is higher than that possible with systemic treatment. This also provides a convenient and controlled dosing scheme that relinquishes issues related to poor patient compliance.23,24 A cement spacer is preferable over other modalities of local antibiotic delivery primarily because it assumes the conjoint functions of inhibiting fibrous tissue ingrowth and maintaining dead space volume until time of reconstruction.15
TIP: Cement Spacers
For optimal outcome, the cement spacer should fill the intramedullary canal and edges of surrounding viable bone.18 Furthermore, we recommend irrigating with cool saline during the exothermic polymerization process to prevent local tissue necrosis (see detailed description below).
The appropriate choice of antibiotic therapy is predicated on the results of culture and pathology testing performed on direct wound and bone samples. Additionally, the chosen antibiotic must be thermostable to the exothermic polymerization (solidification) process of the PMMA cement. There are many option for this purpose with the most common being vancomycin, gentamicin, and tobramycin.25
TIP: Antibiotic-Cement Preparation
High-dose antibiotic-cement preparation is advocated for treatment of long bone PTOM. We typically use 3 g of vancomycin and/or 3.6 g of tobramycin. The largest permissible ratio is 8 g of antibiotic per 40 g of cement, as higher doses of antibiotic may impede cement molding.26
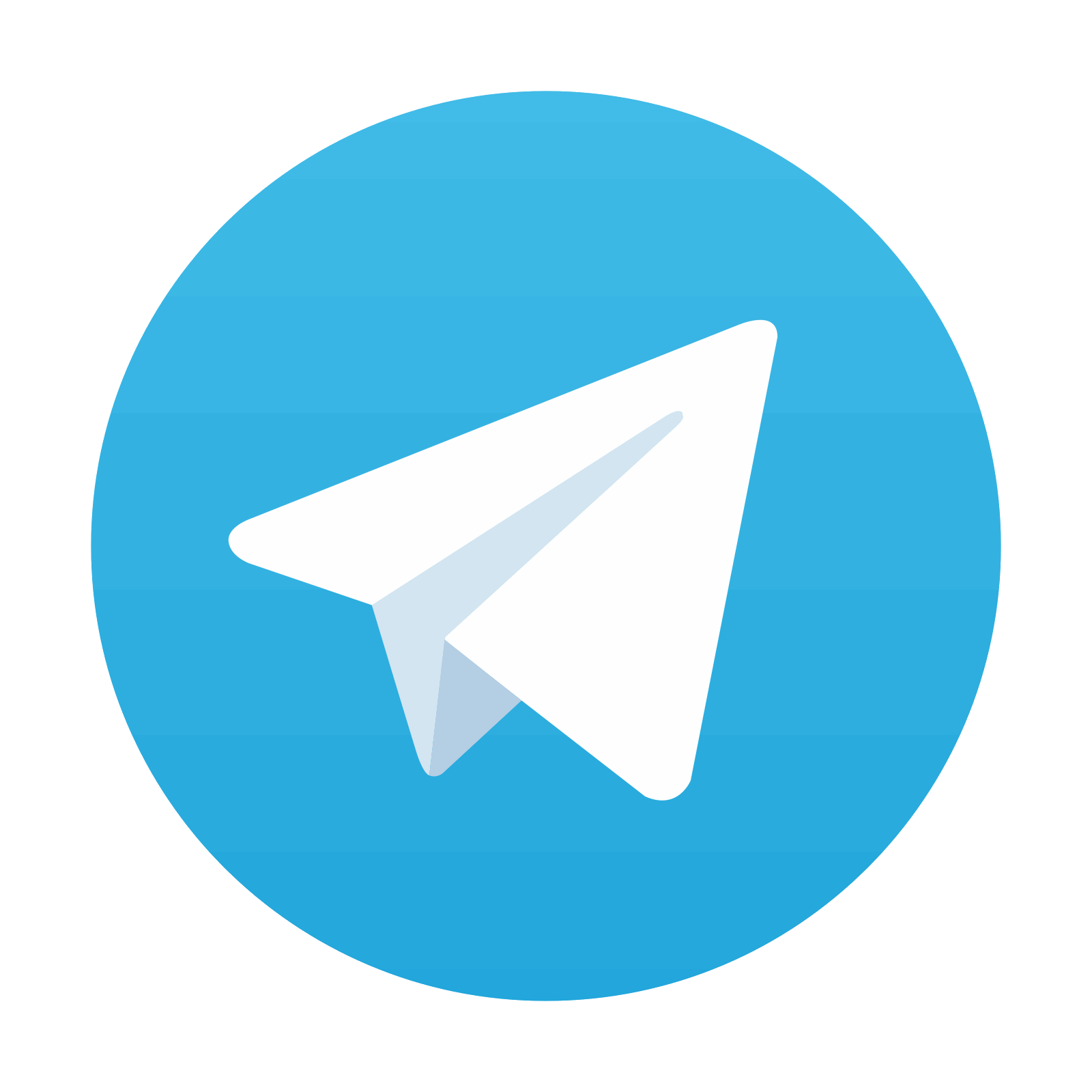
Stay updated, free articles. Join our Telegram channel

Full access? Get Clinical Tree
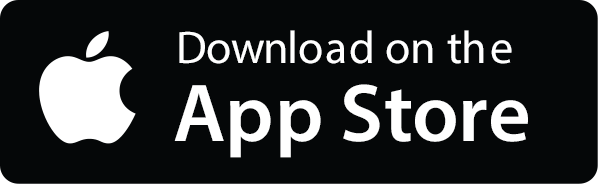
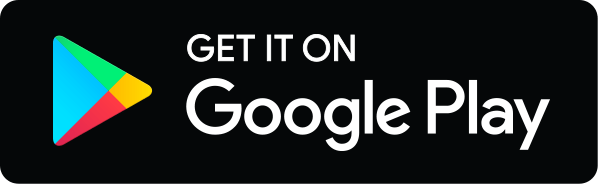