Advanced imaging modality
Pros
Cons
Conventional chromoendoscopy
Detailed surface pit pattern
Useful for dysplasia detection in IBD
Adds time and cost (dyes)
Potential risks with vital stains
Lack of validated classification systems
Evaluation limited to the mucosa
Virtual chromoendoscopy
Detailed surface pit and vascular pattern
Easy and cheap on/off button
Validated classification systems
Useful for neoplasia detection in Barrett’s esophagus, stomach lesions, and colon polyps
Useful for colon polyp characterization
Evaluation limited to the mucosa
Interpretation requires training
Autofluorescence imaging (AFI)
Imaging at greater depth
Low specificity, high false positive rates
Low resolution
Requires special equipment
Confocal laser endomicroscopy (CLE)
High resolution
Visualization of mucosa at cellular level, allows in vivo histology
Time consuming, costly
Typically requires probes (pCLE)
Requires IV contrast agents
Evaluation limited to the mucosa
Optical coherence tomography (OCT)/ volumetric laser endomicroscopy (VLE)
Visualization of mucosa and submucosa at cellular level
VLE can mark abnormal area
Low resolution
Requires special equipment, costly
Requires training
Molecular imaging
High specificity
Adds time and cost
Requires special equipment
Not available for routine clinical use
White Light Endoscopy (WLE) : Standard vs. High Definition
Equipment required for video endoscopy includes a video processor, a light source, the endoscope, and a monitor. An external xenon light source provides the full spectrum of visible white light which travels through fiber-optic glass bundles and is emitted through a lens at the end of the endoscope [4]. Light is reflected off the mucosa, through the objective lens of the endoscope, and reaches the photosensitive surface of the CCD – a small chip in the endoscope tip that senses an image. The CCD captures the image and transmits the charge through electrical wires to the video processor, where a digital image is produced. The initial standard-definition (SD) endoscopes were equipped with 410,000 pixel CCD that provided a digital image that was 640 (width) by 480 (height) [5]. Soon after came the realization that image quality was largely dependent on resolution, which is a function of CCD pixel density.
HDWLE uses smaller chips that produce images with a resolution of more than a million pixels and that are displayed in monitors with either 4:3 or 5:4 aspect ratios and at least 650 pixels in height [6]. In order to truly capture HD images, all of the endoscopy equipment must be HD compatible (endoscope, CCD, processor, monitor, and transmission cables). HD monitors can display progressive images where lines are scanned consecutively and the images painted 60 times per second, which produces fewer artifacts for moving objects. Optical magnification with HD endoscopy can provide images up to 150 times the original size with preserved resolution. This function can be activated with a button in newer endoscopes through a system called near focus, which modifies a mechanical movable lens at the tip of the endoscope [7].
Conventional Chromoendoscopy
This type of AIM enhances the GI mucosa with topically applied dyes to outline lesion borders, highlight surface changes, and delineate mucosal depth. Several methods of dye application are employed depending on the target surface area. For focal suspicious lesions, a 60 mL syringe of diluted dye can be pushed through the instrument channel of the endoscope, and the target area is then examined closely. In cases targeting a larger area of tissue, such as patients with inflammatory bowel disease, a more efficient method for delivering dye is through the water jet irrigation system after mixing 250 mL of normal saline with dye in various concentrations [8]. Each dye has distinct chemical properties designed for different clinical applications.

Squamous cell dysplasia with chromoendoscopy using Lugol’s solution (unstained areas representing areas of dysplasia)
Non-vital dyes are applied to the surface and provide contrast but without being absorbed by the epithelial cells. Indigo carmine is one of the most commonly used non-vital dyes. It collects in the pits and grooves of the mucosa, thereby enhancing visualization of mucosal structures, surface topography, lesion depth, and borders. Acetic acid is a weak acid that induces a chemical reaction in the mucosa with a goal of delineating epithelial structures. Endoscopic delivery of acetic acid through a spray catheter temporarily alters the structure of surface epithelial glycoproteins, which lasts for 2–3 minutes [10]. The unbuffered acid facilitates disruption of disulfide and hydrogen bonds, provokes deacetylation, and in turn denatures the proteins. Repeat application of acetic acid may be necessary to sustain the effect.
Virtual Chromoendoscopy
Virtual chromoendoscopy uses optical lenses and digital processing programs to achieve similar results as conventional chromoendoscopy but with the ease of only pressing a button. The most widely used of these systems is narrow band imaging (NBI, Olympus), which is based on the optical phenomenon that the depth of light penetration into tissue depends on the wavelength; the shorter the wavelength, the more superficial the penetration. In WLE, light at wavelengths 400–700 nm illuminates the surface mucosa and reproduces all images in their natural color. NBI applies an optical filter in real time using a red-green-blue illumination system at a narrower range of 400–540 nm designed to match hemoglobin absorption [11]. This allows structures with high hemoglobin content to appear dark (surface capillaries, brown; submucosal vessels, cyan) which provides a contrast to the surrounding mucosa that reflects the light.
Other systems use the full spectrum of white light to capture images and then perform post-imaging processing. The Fujinon Intelligent Chromoendoscopy (FICE) (Fujinon Inc., Japan) system applies software-based technology to modify images captured through the standard endoscopic video processor [12]. The algorithm selectively enhances specific light wavelengths and creates a reconstructed FICE image. A similar technology is iScan (Pentax, Japan), which uses a digital post-processing system to reconstitute an image [13]. The endoscopist can switch between surface, color, or tone enhancement modes by pressing a button to improve visualization of specific features. Another modality is called blue laser imaging (BLI) or Lasero (Fujinon), which uses a two-laser system. BLI was created in response to the limitations of FICE and NBI as a way to combine the strengths of each individual technology [14]. The limited-wavelength blue laser highlights the mucosal vasculature (similar to NBI), while the second laser induces fluorescent light to illuminate the target.
Autofluorescence Imaging (AFI)
This is a technology dependent on endogenous fluorophores within the GI mucosa, the most important of which is collagen. Fluorophores are naturally occurring substances that absorb energy from short-wavelength light (blue) and in turn emit longer-wavelength light (fluorescent). The patterns of fluorescence vary based on the metabolic activity, blood flow, and biochemical characteristics of the tissue, which can be abnormal with neoplasia and inflammation. Endoscopes with AFI capability have a rotating filter in front of the light source that delivers narrow-spectrum blue light (390–470 nm) alternating with green light (540–560 nm) [15]. There is an additional interference filter whereby only fluorescent and green light are filtered through the CCD to be processed. In the resulting image, normal tissue appears green, and abnormal mucosa appears dark reddish purple in color.
Confocal Laser Endomicroscopy (CLE)
This technology is based on light microscopy, but requires contrast agents administered intravenously (fluorescein) or topically (fluorescein or acriflavine hydrochloride). A laser is then focused by an objective lens to illuminate a single point in the focal plane. Light reflected back from that focal point will converge through a pinhole to the detector [16]. Light that comes from outside the focal point will be scattered and not collected. When the detector processes the light, a high-resolution image at a gray scale will be created showing cellular structures from the mucosal layer (250 um), but not deeper structures. Confocal imaging can be endoscopy based (eCLE) or probe based (pCLE) [17]. Probes are designed to pass through the endoscope working channel toward the target tissue in the biliary tree, upper GI tract, or lower GI tract.
Optical Coherence Tomography (OCT) and Volumetric Laser Endomicroscopy (VLE)
OCT is a disposable probe-based system where long wavelengths of light are used to penetrate into areas of interest and create cross-sectional images [18]. This is similar to endoscopic ultrasound, but infrared light is used instead of acoustic waves to create high-resolution images. A single light source emits two beams, one that is directed at the target tissue and the other to a reference mirror. Light is reflected from both sources and then combined again at a detector to produce interference, which is measured and translated into an image.
VLE uses technology similar to OCT, where rapid scanning facilitates capture of images at a depth of 3 mm with resolution to 10 mm [19]. It is designed for use within a circumferential lumen such as the esophagus. A balloon is passed through the instrument channel and inflated. Then an optical probe is passed through the balloon. The balloon is rotated 360 degrees as the probe is pulled back slightly. The probe VLE has the potential to quickly and effectively image large areas in short periods of time (the entire 6 cm length of the balloon in 90 seconds).
Molecular Imaging
Molecular imaging is an innovative technology where targeted probes are directed to specific molecules in the GI tract. A molecular probe can be designed using a peptide, antibody, nanoparticle, or other molecules [20]. Peptides are the most commonly described probes in molecular endoscopy as they offer certain advantages. They are small for mucosal penetration, are safe, have low immunogenicity, and are relatively easy and inexpensive to mass-produce. The peptide is isolated using a bacteriophage library and then labeled to a fluorophore to be applied topically during endoscopy using a spray catheter. Use of a multimodal video endoscope provides images using a special fluorescent and reflectance filter [21]. This technology has the potential for more accurate in vivo diagnosis and prediction of patients with higher risk of progression into neoplasia before morphologic changes even develop.
Endoscopic Evaluation of the Upper GI Tract
Barrett’s Esophagus, Dysplasia, and Esophageal Adenocarcinoma
Rationale and Limitations of Surveillance Endoscopy
The global incidence of esophageal adenocarcinoma (EAC) is 0.7/100,000 person years and has significantly increased in Europe, Australia, and the United States in the last four decades [22, 23]. Most cases of EAC are diagnosed at advanced stages, which is associated with dismal survival and poor quality of life [24]. Barrett’s esophagus (BE) or intestinal metaplasia (IM) of the esophagus is the precursor lesion for EAC and can be detected endoscopically in the presence of salmon-colored mucosa extending more than 1 cm proximal to the gastroesophageal junction with confirmed IM on biopsies [25].
Progression of BE to EAC involves a series of pathologic changes from non-dysplastic BE (NDBE) to low-grade dysplasia (LGD), high-grade dysplasia (HGD), and finally EAC [26]. Thus, endoscopic surveillance with targeted biopsies of visible lesions and four-quadrant random biopsies every 1–2 cm (Seattle biopsy protocol) is endorsed by international society guidelines to detect dysplasia or EAC at earlier stages, receive curative therapy, and enhance survival [25, 27–30]. Moreover, this approach can help identify patients with neoplastic lesions who are amenable to endoscopic eradication therapies (EETs) in lieu of surgery or chemoradiation. However, this approach has several limitations including sampling errors (focal distribution of neoplasia and surveillance biopsies sample only 5% of the Barrett’s segment), limited reliability of histologic interpretation of dysplasia, and the associated costs, time, and labor, which may explain why community endoscopists do not adhere to the Seattle biopsy protocol [31, 32]. In addition, visible lesions can be easily missed because they are often small and focally distributed.
Endoscopic Inspection of BE
The endoscopist should inspect the Barrett’s segment in a systematic fashion to maximize detection of visible lesions which can harbor dysplasia or early cancer. Careful evaluation of BE with HDWLE is recommended as the minimum standard to maximize detection of visible lesions [27, 33]. However, there are no randomized clinical trials directly comparing HDWLE with standard WLE for detection of visible lesions in BE, and this recommendation is inferred from several other studies [34, 35]. Longer inspection time, along with careful and organized BE inspection, may be associated with higher number of lesions detected and increased diagnosis of HGD/EAC [33]. Careful endoscopic examination can reassure detection of >80% of lesions with HGD/EAC [36].
The following recommendations can be considered to ensure high-quality care. First, consider the use of a transparent distal attachment cap on the tip of the endoscope to facilitate endoscopic view especially in patients with BE-related neoplasia. Second, clean the mucosa by using the water jet channel and carefully suctioning the fluid with minimal mucosal trauma. Third, inspect the suspected BE by varying insufflation and desufflation to detect subtle surface irregularities. Fourth, inspect the distal Barrett’s segment in a retrograde view. Fifth, describe the location of the diaphragmatic hiatus, gastroesophageal junction, and squamocolumnar junction, as well as the extent of BE including circumferential and maximal segment length using the Prague classification [37]. After adequate inspection of BE, biopsies can then be performed. Biopsies should be avoided in normal or irregular Z line to avoid overdiagnosis of BE in patients who in fact have IM of the cardia which is not associated with EAC and in areas of erosive esophagitis until optimizing antireflux therapy, as reparative changes from active esophagitis can be difficult to distinguish from dysplasia.
Uniform Evaluation of Visible Lesions
Subtle mucosal abnormalities, such as ulceration, erosion, plaque, nodule, stricture, or other luminal irregularities in the Barrett’s segment, should be sampled separately, as there is an association of such lesions with underlying dysplasia and cancer [38]. These mucosal abnormalities should undergo endoscopic mucosal resection (EMR), as this provides a better sample for pathologic review and changes the histopathologic diagnosis in approximately 30–50% of patients, compared with biopsies [39, 40]. Moreover, EMR of suspicious esophageal lesions represents a quality indicator of EET of BE, both as a diagnostic (to determine the T-stage and/or grade of dysplasia) and therapeutic maneuver [35]. Chapter 3 of this book offers further details regarding esophageal EMR techniques.

Description of visible lesions in Barrett’s esophagus using the Paris classification. (a) Flat Barrett’s esophagus without visible lesions. (b) Paris IIa diffuse nodularity within Barrett’s segment. (c) Paris IIa and IIc lesion within Barrett’s segment
Quality Indicators of Endoscopic Surveillance
Quality indicators for endoscopic eradication therapy (EET) in Barrett’s esophagus (BE) and suggested median threshold benchmark
Type | Metric | Threshold |
---|---|---|
Pre-procedure | The rate at which the reading is made by a GI pathologist or confirmed by a second pathologist before EET is begun for patients in whom a diagnosis of dysplasia has been made | 90% |
Centers in which EET is performed should have available HDWLE and expertise in mucosal ablation and EMR techniques | NA | |
The rate at which documentation of a discussion of the risks, benefits, and alternatives to EET is obtained from the patient prior to treatment | >98% | |
Intra-procedure | The rate at which landmarks and length of BE are documented (e.g., Prague grading system) in patients with BE before EET | 90% |
The rate at which the presence or absence of visible lesions is reported in patients with BE referred for EET | 90% | |
The rate at which the BE segment is inspected by using HDWLE | 95% | |
The rate at which complete endoscopic resection (en bloc resection or piecemeal) is performed in patients with BE with visible lesions | 90% | |
The rate at which a defined interval for subsequent EET is documented for patients undergoing EET who have not yet achieved complete eradication of intestinal metaplasia | 90% | |
The rate at which complete eradication of dysplasia is achieved by 18 months in patients with BE-related dysplasia or intramucosal cancer referred for EET | 80% | |
The rate at which complete eradication of intestinal metaplasia is achieved by 18 months in patients with BE-related dysplasia and intramucosal cancer referred for EET | 70% | |
Post-procedure | The rate at which a recommendation is documented for endoscopic surveillance at a defined interval for patients who achieve complete eradication of intestinal metaplasia | 90% |
The rate at which biopsies of any visible mucosal abnormalities are performed during endoscopic surveillance after EET | 95% | |
The rate at which an antireflux regimen is recommended after EET | 90% | |
The rate at which adverse events are being tracked and documented in individuals after EET | 90% |
Advanced Imaging Modalities (AIMs) to Enhance Surveillance
Several AIMs have been investigated to overcome some of the limitations of current surveillance practices of BE with WLE. A Preservation and Incorporation of Valuable Endoscopic Innovations (PIVI) statement from the American Society of Gastrointestinal Endoscopy (ASGE) has outlined thresholds for performing AIMs during endoscopic surveillance of BE [42]. To eliminate random biopsies, an AIM with target biopsies should have the following characteristics: (1) per-patient sensitivity of ≥90% and a negative predictive value of ≥98% for detecting HGD/EAC, compared with the current standard protocol, and (2) specificity of ≥80% to allow a reduction in the number of biopsies compared with biopsies obtained using the Seattle protocol. A recent meta-analysis demonstrated that only experts in the field of BE meet these thresholds with acetic acid chromoendoscopy, NBI, and eCLE [43]. Thus, AIMs should not yet replace surveillance endoscopy with random biopsies in non-expert hands. However, AIMs can increase the diagnostic yield for identification of HGD/EAC if added to the Seattle protocol, as recently demonstrated in a meta-analysis with 34% and 35% incremental yield of HGD/EAC with virtual and conventional chromoendoscopy, respectively [44]. In head-to-head studies, both chromoendoscopy modalities have demonstrated comparable detection of HGD/EAC [34, 45].
Virtual Chromoendoscopy

Abnormal NBI pattern of visible lesions in Barrett’s esophagus. (a) Paris IIa and IIc lesion with abnormal NBI pattern from 9 to 1 o’clock position and with normal NBI pattern from 1 to 9 o’clock position. (b) Paris Is lesion in the GE junction with abnormal NBI pattern
Conventional Chromoendoscopy
The dyes most commonly used for conventional chromoendoscopy in BE are acetic acid and methylene blue. No standardized classification criteria have been established for any dye. In the meta-analysis by Thosani et al., acetic acid chromoendoscopy was found to meet the thresholds established by the ASGE PIVI (sensitivity, 97%; negative predictive value, 98%; and specificity, 85%) and can be used in clinical practice at least by experts [43]. In contrast, methylene blue chromoendoscopy fails to meet these thresholds (sensitivity, 64%; negative predictive value, 70%; and specificity, 96%) and does not increase the diagnostic yield over random biopsies for the detection of HGD/cancer [43, 51]. Furthermore, the safety of methylene blue has been questioned as one study suggested that it can cause induce oxidative damage to DNA when photosensitized with light [52]. Acetic acid causes disruption of the columnar mucosal barrier in minutes, leading to whitening of the tissue with vascular congestion and accentuation of the villi and mucosal pattern when the acid reaches the stroma. The whitening effect in dysplastic areas is lost earlier than in the surrounding mucosa, which helps identify neoplastic areas.
Role of AFI, CLE, VLE, and OCT
Other AIMs have been investigated, but none appear to be ready for clinical application at the present time [53]. AFI is limited by its high false positive rate, fair to moderate inter-observer agreement, and minimal incremental diagnostic yield over the Seattle protocol [54]. CLE has the potential to confirm a real-time diagnosis of neoplasia without the need for histology, which could lead to immediate endoscopic therapy without biopsies, such as same-session EMR or ablative therapy. Use of eCLE meets the ASGE PIVI thresholds but is no longer commercially available, while pCLE does not meet these thresholds [43]. A meta-analysis recently showed that VLE is associated with a marginal increase in detection of HGD/cancer and has very high rates of false positive results [55]. However, OCT and VLE can evaluate epithelial thickness and buried glands, which can predict prolonged or failed ablation, and be useful in post-endoscopic ablation surveillance [56, 57]. The clinical applicability of these AIMs needs to be better defined before recommending their routine use in surveillance of BE.
Gastric Intestinal Metaplasia, Dysplasia, and Cancer
Rationale of Screening and Surveillance
Gastric cancer (GC) is one of the most frequent and lethal malignancies worldwide. The introduction of universal screening in Korea and Japan is associated with earlier GC diagnosis and lower cancer-related mortality [58–60]. Thus, universal screening is warranted in individuals from high-incidence countries, but is more selective in low-incidence countries based on demographic data and Helicobacter pylori status [61]. This translates in higher rates of early GC diagnosis – lesion confined to the mucosa or submucosa – in countries with national screening programs compared to Western countries (60 vs. 20%), which can be safely treated by mucosal or submucosal endoscopic resection [62, 63].
Compared with noninvasive tests, endoscopy is the best and most cost-effective screening modality to detect precancerous lesions and GC [64]. The development of intestinal-type GC is preceded by a cascade of several precancerous events that range from non-atrophic gastritis, multifocal atrophic gastritis (AG), IM, dysplasia, and ultimately GC [65]. Management and surveillance intervals are determined based on the individual histologic risk of progression into GC. A population study from the Netherlands illustrated this by showing an annual incidence of GC of 0.2% for AG, 0.3% for IM, 0.6% for mild-moderate dysplasia, and 6% for severe dysplasia [66]. The risk of GC with AG and IM can then be further stratified based upon location, severity, and extension of the lesion. Patients with widespread atrophy or IM pose high risk of cancer and require endoscopic surveillance every 3 years. Patients with LGD should be followed every 12 months, while those with HGD should be followed every 6 months or have the lesion resected [67].
Endoscopic Evaluation of Stomach Lesions

Representative endoscopic images of gastric neoplasia . (a) Paris Is and IIc friable gastric mass. (b) Ulcerated gastric mass with abnormal NBI pattern. (c) Chromoendoscopy with methylene blue determining outer margins of early gastric cancer that was ultimately resected
Role of Virtual and Conventional Chromoendoscopy
After recognition of suspicious lesions with WLE, virtual and conventional chromoendoscopy help in lesion characterization and highlight lesion outer margins (Fig. 1.4b, c). Diagnostic accuracy of NBI is maximized with magnifying endoscopy, by analyzing the microvascular and microsurface patterns separately. In a recent meta-analysis of 14 studies, magnifying NBI showed high sensitivity (86%) and specificity (96%) for detection of early GC [71]. This showed to be especially helpful for depressed or small lesions ≤10 mm in size, which can be more accurate than with conventional chromoendoscopy [71, 72]. Magnifying NBI can also delineate the lateral margins of a lesion even when conventional chromoendoscopy is not able to determine the margins [73]. Further research is needed to establish a standard NBI classification system to reduce various biases and improve its diagnostic accuracy in the assessment of gastric lesions. For example, fine network patterns with abundant microvessels connected one to another are characteristic of adenocarcinoma, and a corkscrew pattern with tortuous isolated microvessels is characteristic of poorly differentiated adenocarcinoma. Conventional chromoendoscopy with indigo carmine and acetic acid has been used in clinical practice for evaluation of gastric lesions, but delineation of margins is not superior to NBI.
Role of AFI and CLE
The role of other AIMs has not been fully established in the screening or surveillance of GC. AFI has limited clinical value due to its high false positive rate and low specificity. CLE has shown encouraging results for the in vivo diagnosis of premalignant lesions and early gastric cancer [74].
Duodenal Adenomas and Cancer
Rationale for Screening and Surveillance
Duodenal cancer is rare among all GI malignancies. For several years, it has been recognized that this malignancy arises from an adenoma-to-carcinoma pathway similar to colorectal cancer (CRC) [75]. Duodenal adenomas should be categorized as being ampullary or non-ampullary and as sporadic or arising in the context of familial adenomatous polyposis (FAP). The lifetime risk of duodenal cancer in patients with FAP is 5–10%, while in the general population, it ranges from 0.01% to 0.04% [76]. In addition, duodenal adenomas are diagnosed in up to 90% of FAP patients, can be multiple, and involve the ampulla. Thus, endoscopic screening and surveillance are recommended in FAP patients [77].
Endoscopic Evaluation

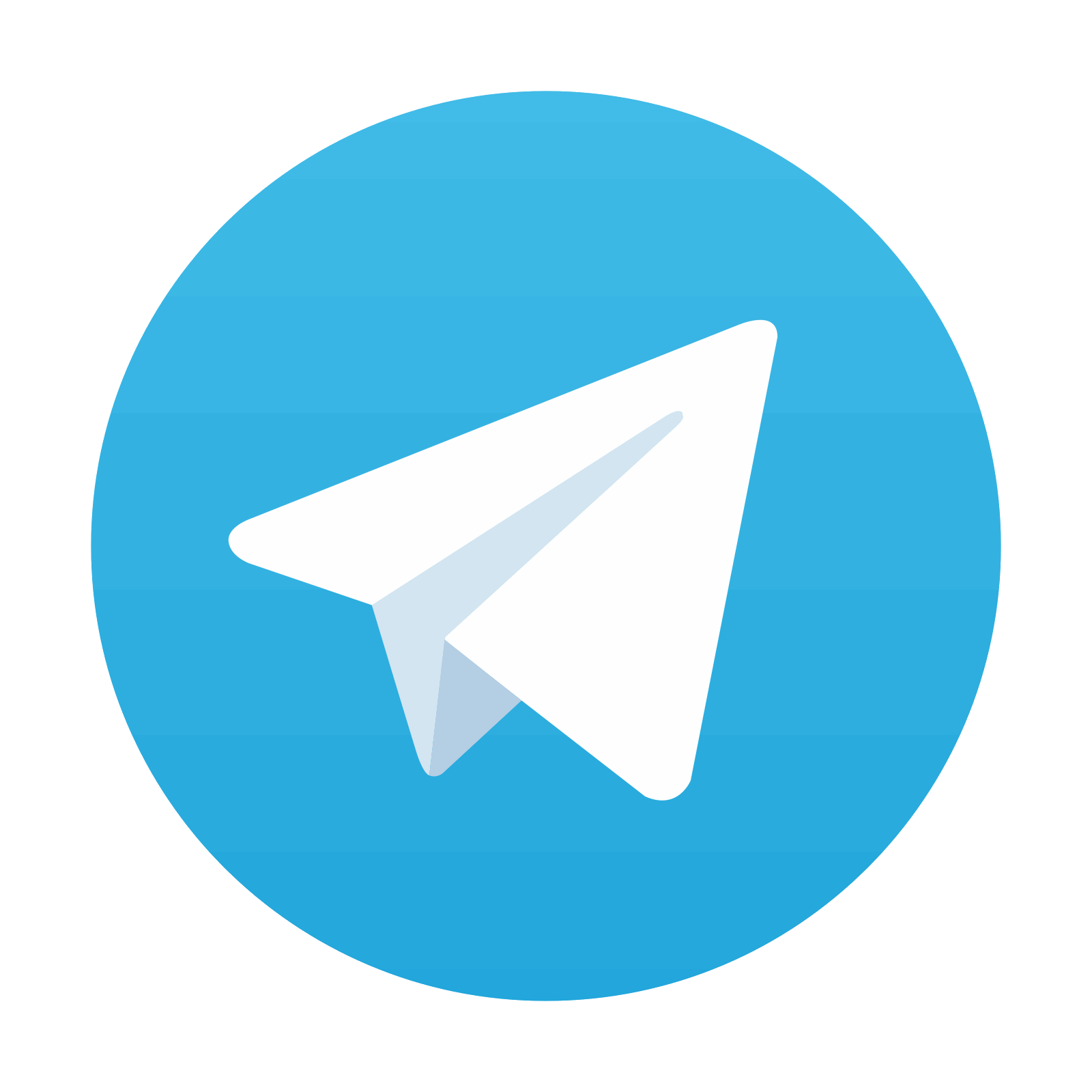
Stay updated, free articles. Join our Telegram channel

Full access? Get Clinical Tree
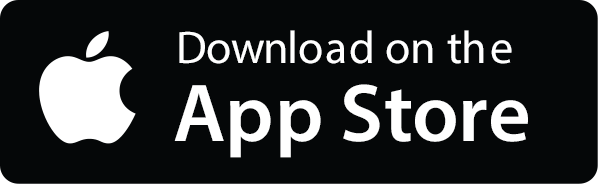
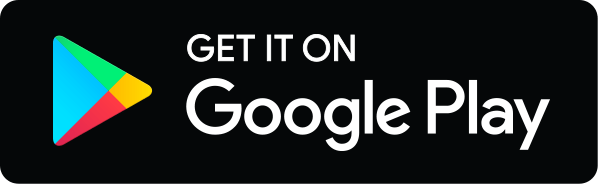