Laparoscopic Ablation of Small Renal Masses
DANIEL RAMIREZ
JEFFREY A. CADEDDU
The pervasiveness of cross-sectional abdominal imaging over the last decade has substantially increased the rate of incidentally diagnosed small renal masses (SRMs). This trend has impelled minimally invasive urologists to examine alternatives to complex extirpative surgical treatments. Cryotherapy and radiofrequency ablation (RFA) were initially described over a decade ago (1,2) with maturation of large, long-term series with these ablative techniques now available. Unfortunately, there currently exist no randomized controlled trials comparing ablative techniques with surgery or active surveillance. Available evidence has largely been mined from retrospective cohort reviews and case series. Ablative treatment particularly offers some advantages over partial nephrectomy, including decreased convalescence and improved preservation of renal function. Comparative studies have shown ablative techniques to have decreased blood loss, decreased length of stay, lower complication rates, and superior renal preservation when compared to partial nephrectomy (3,4,5). Traditionally,
ablation has been reserved for older patients who are poor candidates for surgery or those patients who require maximal preservation of renal function. With oncologic outcomes and treatment efficacy of RFA and cryotherapy nearing that of surgical extirpation, many academic hospitals are now offering ablative techniques as first-line treatments for SRMs in the young and healthy patient. This chapter will focus on the rational and indications for renal ablative therapy with specific emphasis on a laparoscopic approach to applying RFA and cryotherapy for the treatment of SRMs.
ablation has been reserved for older patients who are poor candidates for surgery or those patients who require maximal preservation of renal function. With oncologic outcomes and treatment efficacy of RFA and cryotherapy nearing that of surgical extirpation, many academic hospitals are now offering ablative techniques as first-line treatments for SRMs in the young and healthy patient. This chapter will focus on the rational and indications for renal ablative therapy with specific emphasis on a laparoscopic approach to applying RFA and cryotherapy for the treatment of SRMs.
INDICATIONS FOR LAPAROSCOPIC APPROACH
Ablation of SRMs, in general, is largely reserved for tumors measuring less than 4 cm in size. The authors of this chapter typically reserve ablation for tumors measuring less than 3.5 cm because oncologic efficacy is inferior for tumors larger than this size (6). Historically, ablative therapy has also been reserved for patients with limited renal functional capacity, bilateral or multiple masses, and patients with genetically inherited renal cell carcinoma (RCC) syndromes requiring management of multiple metachronous or synchronous lesions. Typically, ablative therapy for SRMs has been employed via a percutaneous technique because it minimizes morbidity. Nevertheless, there are specific instances where a percutaneous approach may not be feasible or is too dangerous to pursue. A laparoscopic approach is indicated when there is a necessity to physically mobilize critical structures away from the area of ablation and to allow for direct visualization of the ablated area in relation to other structures. For these reasons, the rate of intraoperative complications during laparoscopic ablative treatment of SRMs is low (7,8,9,10,11). A laparoscopic approach is usually indicated for masses in an anterior or lateral location because this is where many vital structures converge and where percutaneous probes cannot reach. Posterior tumors can generally be treated percutaneously, although a retroperitoneal laparoscopic approach may also be applied.
The only absolute contraindication to laparoscopic ablation of SRMs is irreversible coagulopathy. Relative contraindications include inability to tolerate insufflation necessary for laparoscopic abdominal surgery, tumors with cystic components, large diameters, and close approximation to hilar structures. Ablation of endophytic renal lesions not seen on ultrasound preoperatively should not be attempted via a laparoscopic approach because identification of the mass is dependent on the surgeon’s ability to identify it on intraoperative ultrasonic imaging.
MECHANISMS OF ACTION OF ABLATIVE THERAPIES
Cryotherapy
Cryoablation is the most studied ablative procedure for renal masses and was first described in canine models in 1994 by Uchida et al. (12). Since that time, cryoablation techniques have improved with the introduction of small probes and use of argon and helium gas, enhancing the accuracy of the freeze-thaw cycle. The smallest available probes needles are 17-gauge (1.47 mm) in size (Endocare, Irvine, California).
Once probe placement is confirmed on intraoperative imaging, argon gas is rapidly injected through the tip of the needle which briskly creates an ice ball. Once the freezing cycle is complete, pressurized helium is then forced through the needle probe to induce thawing. This precipitous freezing of renal tissue results in extracellular and intracellular ice crystal formation leading to acute intracellular pH changes, protein denaturation, decreased intracellular osmotic concentration, and immediate physical mechanical destruction of the cellular membrane (13). Injury to vascular endothelium is also exhibited which results in demonstrable microvascular thrombosis and ischemia leading to delayed necrosis over hours and days after treatment. Freeze-thaw cycles reliably and reproducibly cause necrosis of renal parenchyma at temperatures at or below -19.4°C (14). Malignant lesions may require even lower temperatures to result in cellular death, thus most clinical protocols call for freezing temperature of -40.0°C (15). Experimental models have demonstrated that ice balls produced via cryotherapy only reach these extreme temperature 3.1 mm within their inner edge (16). Because of this, the ice ball is allowed to extend 5 to 10 mm beyond the boundary of the renal lesion in clinical practice.
Ice ball size and temperature monitoring are verified with intraoperative imaging (computerized tomography [CT], magnetic resonance imaging [MRI], or ultrasound [US]) and thermocouples found at the end of the ablation probes. The majority of large clinical series describing outcomes of cryoablative treatments of SRMs use a double freeze-thaw cycle to ensure cellular death. This has been shown to increase oncologic efficacy compared to single-cycle protocols (17).
Radiofrequency Ablation
The mechanism of RFA relies on the transformation of highfrequency electromagnetic waves into heat causing thermal damage. The increase in temperature is not applied directly by the probe. An alternating monopolar current is passed from the electrode in to the target lesion, resulting in ionic disturbance and molecular friction which, in turn, causes a release of energy as heat (18). This increase in thermal energy results in permanent protein denaturation and desiccation which occurs instantly at a temperature greater than 60°C (19). These extreme temperatures also lead to breakdown of cell membrane, nuclear instability, microvascular thrombosis, and, ultimately, ischemia and necrosis of target tissue (20). The currently available platforms for RFA differ in the manner in which they modulate energy delivery. Temperature-based feedback inhibition (AngioDynamics, Latham, New York) measures the temperature at the edge of the ablation field in order to avoid overheating. Impedance-based feedback inhibition (RF 3000, Boston Scientific, Natick, Massachusetts; Cool-tip, Covidien, Mansfield, Massachusetts) reduces delivered current by sensing the increased resistance in target tissue. These feedback inhibition mechanisms reduce the production of charred tissue, thus improving efficacy and maintains the size of the ablation zone.
Concurrent intraprocedure monitoring of the lesion with CT, US, or MRI is problematic due to the production of microbubbles and poor innate delineation on imaging between normal renal parenchyma and tissue ablated via RFA (21).
However, real-time imaging may be unwarranted as the efficacy of RFA is determined by the deployment diameter of the probe tines and maximum temperature reached. In contrast to the ice ball seen in cryoablation, the size of the lesion does not grow with increased activation time beyond the minimum threshold.
However, real-time imaging may be unwarranted as the efficacy of RFA is determined by the deployment diameter of the probe tines and maximum temperature reached. In contrast to the ice ball seen in cryoablation, the size of the lesion does not grow with increased activation time beyond the minimum threshold.
SURGICAL TECHNIQUE AND POSTOPERATIVE CARE
Perioperative Preparation and Considerations
Careful assessment of the patient’s past surgical history and evaluation of cross-sectional imaging should be performed in each case to identify the best surgical candidate for laparoscopic ablation. Appropriate consent should be obtained from the patient after the risks and benefits of ablation and other alternative procedures are discussed in detail. A transperitoneal approach is employed for superior and anterior renal masses. A retroperitoneal method may be considered for posterior and lateral tumors when a percutaneous technique is not possible. Renal ultrasound should also be performed on masses that are found to be endophytic on cross-sectional imaging in order to assess whether intraoperative ultrasonic evaluation can be successfully performed. Completely endophytic masses that are not seen on preoperative renal ultrasound should not be treated with laparoscopic ablation as intraoperative identification may not be feasible.
Positioning, Port Placement, and Operative Technique for Exposure of the Tumor
Once the patient is bought to the surgical theater, a Foley catheter is placed to ensure complete decompression of the bladder and the patient is appropriately positioned. For a transperitoneal approach, the patient is placed in a modified flank position. A standard flank position is applied when a retroperitoneal approach is proposed.
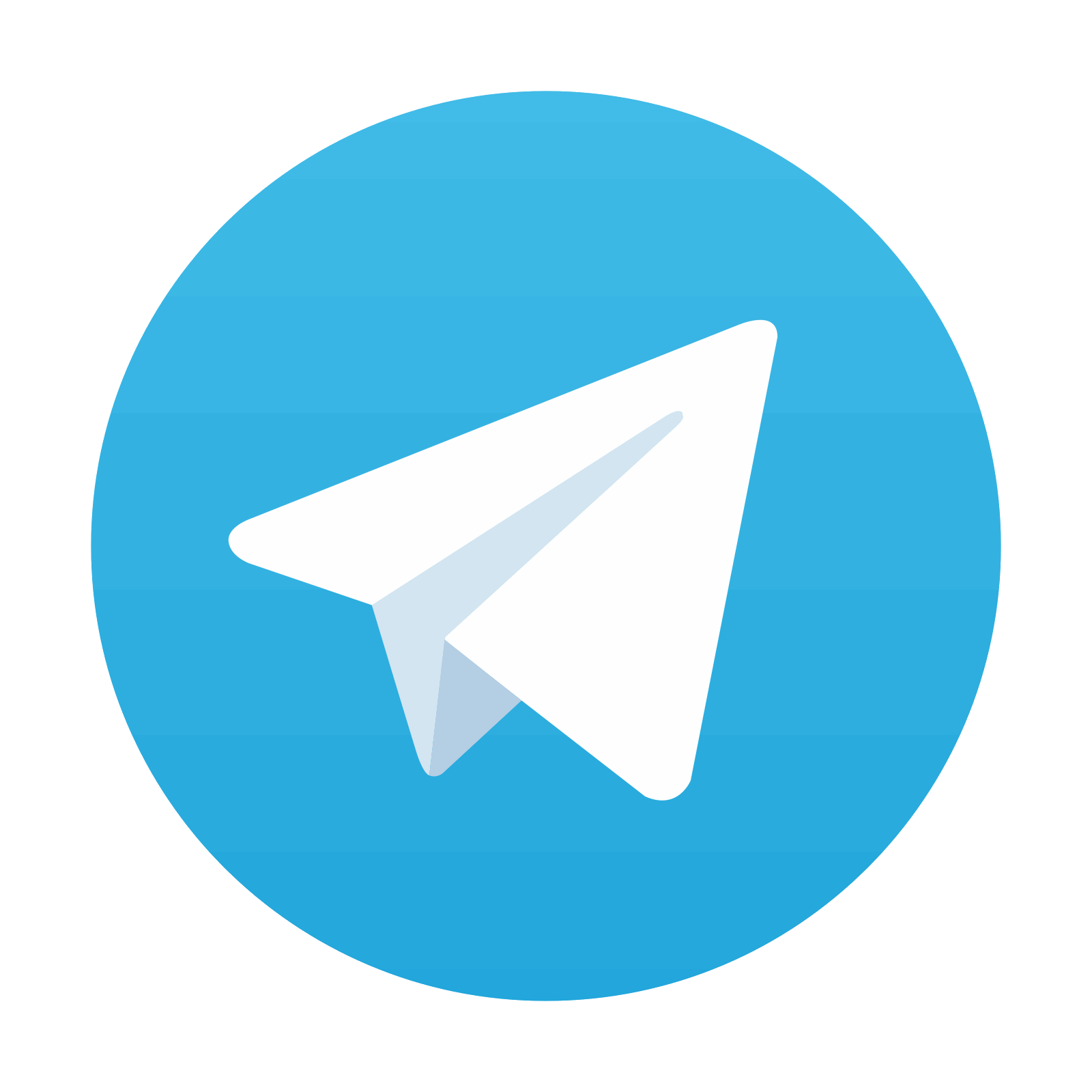
Stay updated, free articles. Join our Telegram channel

Full access? Get Clinical Tree
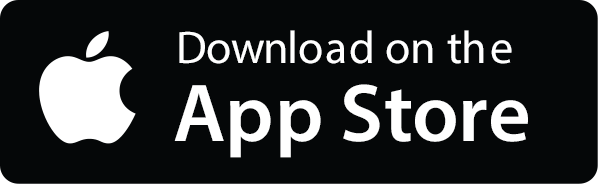
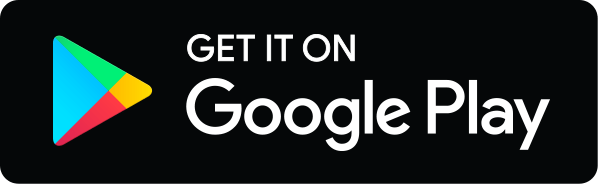