Introduction
At the start of the first transplant programs, the donor and recipient would be operated in the same surgical center. The only preservation method therefore undertaken would be to flush out the kidney with either blood or Ringer’s lactate and to use surface cooling with ice water, because hypothermia was supposed to be protective by reducing metabolism. Very early transplant work in twins and animal studies suggested that an ischemic time of less than 1 hour should be aimed for, with irreversible damage suspected beyond 3 hours. This target would later be extended to 12 hours with improved flushing and cooling. The necessity of transporting kidneys to compatible recipients in other local centers meant adequate preservation for longer periods was needed. The first method of addressing this was the machine preservation system developed by Belzer ( Fig. 9.1 ). It was not until the late 1960s that preservation fluids were developed that allowed static cold storage without continuous perfusion.

Modern transplant programs often involve the sharing of organs over much larger geographic distances. The sharing of kidneys in this way permits better matching between donor and recipient, but may have the additional effect of longer cold ischemic times, which can affect graft survival.
The first transplant programs used either live donors or donation after circulatory death (DCD) when the opportunity to quickly perform organ retrieval was available. In 1968 a committee at Harvard Medical School met to examine and redefine the definition of brain death. This was followed by an expansion in the use of organs from donation after brain death (DBD). These were typically younger people with irreversible brain damage during motor vehicle accidents, referred to as standard criteria donors (SCD). There is a declining number of this donor type, and transplant programs are therefore turning to older and higher risk donors, previously defined as expanded criteria donation (ECD) and DCD.
The change in the risk profile for kidneys we now use to meet the demand for organs means that it is now necessary to revisit our methods of preservation. The UK Kidney Donor Risk Index (UKKDRI) uses donor factors shown in Table 9.1 to predict overall transplant survival. The proportion of DBD kidneys transplanted in the UK from high-risk donors (HR ≥1.35) using this index has increased from 29% to 39% in the past 10 years. The Kidney Donor Profile Index (KDPI) is used to predict the risk of failure for a kidney transplant in the US; the current proportion of deceased donor kidneys with the worst KDPI (86%–100%) is 18%, having risen from 14% in 2012.
Factors Used to Calculate the UKKDRI, Watson et al. | Factors Used to Calculate the KDRI and KDPI, OPTN |
---|---|
Donor age History of hypertension Donor weight Use of adrenaline Days in hospital | Donor age History of hypertension Donor weight Donor height Ethnicity/race History of diabetes Cause of death Serum creatinine Hepatitis C virus status DCD/DBD |
The increasing use of DCD kidneys has also prompted a renewed interest in preservation methods; despite the adequate long-term function of kidneys from DCD, they still have a higher risk of delayed graft function (DGF) compared with kidneys from DBD. In the UK the use of DCD kidneys has risen dramatically, from 3% of deceased donor transplants in 2000 to 42% in 2017. In the Eurotransplant region the use of DCD kidneys has permitted a large increase in available kidneys for transplantation. The use of DCD in the US has increased, although not at such a rapid rate; DCD accounted for approximately 17% of deceased donor kidney transplants in the US in 2016, having increased from 10% in 2007.
Organ Damage After Death
Organ damage around the time of death can be divided into five broad phases that have different implications for the transplant and may potentially be addressed by targeting preservation techniques at each phase ( Fig. 9.2 ).

Cerebral injury and brain death are associated with the release of cytokines and the initiation of inflammatory processes that can directly injure organs and lead to further immune damage at reperfusion in the recipient. These changes in immunogenicity are characterized by increased expression of damage-associated molecular patterns (DAMPs) that may include cytokines, adhesion molecules, and major histocompatibility complex class II antigens in the retrieved organ. Hormonal changes after brain death include reduced levels of antidiuretic hormone and thyroid hormones. Brain death also causes myocardial suppression and decreased vascular tone, which results in hypotension and inadequate perfusion of organs. Kidneys in this environment are exposed to perfusion dynamics and inflammatory cytokines that can lead to necrosis of renal tubules and fibrous proliferation of the arterial intima.
Organs from DCD are exposed to inflammatory cytokines, but in a less profound way to DBD. Organs from DCD, however, undergo a period of warm ischemia before retrieval. The extent of this damage is related to the situation of the donor, and this may be categorized using the Maastricht system, which is shown in a modified form in Table 9.2 . It should be noted that not all of these categories are acceptable in some countries. In the case of Maastricht category III donors, the warm ischemic period follows the withdrawal of supportive treatment once a retrieval team is ready, intending to minimize warm ischemic time. In some cases the withdrawal of support may be prolonged, and a systolic blood pressure of less than 50 mm Hg results in functional warm ischemia and may affect the transplant outcome. In the case of the so-called “uncontrolled” donors of Maastricht categories I and II, there may be a prolonged warm ischemic period after circulatory arrest and before cold perfusion of the organs can be achieved.
Uncontrolled DCD | |
I. Found dead | Sudden unexpected cardiac arrest without any resuscitation by a medical team; may be in hospital or out of hospital |
II. Witnessed cardiac arrest | Sudden unexpected irreversible cardiac arrest with unsuccessful resuscitation by a medical team; may be in or out of hospital |
Controlled DCD | |
III. Withdrawal of life-sustaining treatment | Planned withdrawal of life-sustaining therapy, expected cardiac arrest |
IV. Cardiac arrest while brain dead | Sudden cardiac arrest after brain death diagnosis during donor life-management but before organ recovery |
The period between the initial flush of cold preservation fluid through the organ’s arteries in the donor and the reperfusion of an organ with blood in the recipient is the cold ischemic time (CIT). The length of this potentially modifiable period is an important factor in determining the outcome of solid-organ transplantation. Analysis of the Collaborative Transplant Study (CTS), a voluntary, multinational database of transplant outcomes, found that kidneys preserved for longer than 19 hours had worse graft survival. A longer CIT is also associated with an increasing risk of DGF of kidneys.
The simplest method of dealing with the harmful cellular processes after donor death has been to cool the kidneys. This slows down the metabolism but does not completely stop it, leading to ongoing damage in cold-preserved organs, and hence the critical importance of limiting cold ischemic times.
Cold Storage
When a kidney is removed from the donor’s body, perfusion completely ceases, leading to an absence of oxygen and nutrient delivery to the renal cells, which will rapidly lead to serious metabolic problems. Suppression of metabolism is therefore essential to maintain organ viability during the preservation period. Reduction of the core temperature of the kidney below 4°C will result in a reduction of metabolism to 5% to 8% and will diminish enzyme activity. The concept of simple cooling with ice proved to be successful; however, it was unfit for longer preservation periods. The concept of simple cold static storage was investigated by several groups, resulting in new solutions and providing an insight into the side effects of ischemia in the preserved organ. Transplant pioneers started perfusion of kidneys with cryopreserved plasma-based solutions, leading to improved preservation, although accompanied by logistical problems. In current clinical practice several preservation fluids are used. Both in situ in the deceased donor, and on the theater workbench, kidneys are flushed through the renal artery with a preservation fluid until donor blood is completely cleared. The kidney is then packaged in a sterile bag of this fluid and kept on ice in a cool box. It is important that handling of the kidney and workbench preparation are undertaken with the organ submerged in preservation fluid. The fluid should also be kept on ice until required, to prevent warming to ambient temperature. This method is relatively cheap, easily transportable, and does not require input from the retrieval or implant team during the preservation period.
The classic unwanted side effects of hypothermia are swelling, acidosis, altered enzyme activity, and production of radical oxygen species upon reperfusion. Effective preservation solutions are therefore composed to counteract these processes using different types of buffers, electrolyte compositions, and additives. These processes are summarized in Fig. 9.3 .

Cell Swelling
The main cause of cell swelling is the impaired activity of Na + /K + ATPase. As a result, sodium passively enters cells, attracted by the negative charge of cytoplasmic proteins. This creates a hyperosmolar intracellular environment and subsequently an influx of water. To reestablish the disturbed Donnan equilibrium and to prevent cell swelling, impermeants such as colloids (hydroxyethyl starch in the University of Wisconsin (UW) solution or polyethylene glycol in IGL-1) are added to preservation solutions. It was thought that intracellular composition was required to diminish the exchange of electrolytes. The high potassium, however, also leads to initial vasoconstriction, hampering flush-out in the donor. The extracellular-type solutions are as effective as the intracellular solutions indicating that creating an osmotic pressure is more important than the composition.
Energy and Acidosis
The absence of oxygen during storage results in a rapid fall in intracellular ATP levels. Even at 0°C to 4°C cellular ATP content is rapidly depleted, and cells will switch to anaerobic metabolism of glucose. This will lead not only to a much less efficient production of ATP but also to production of lactic acid. Severe acidosis activates phospholipases and proteases causing lysosomal damage and eventually cell death. Adequate control of pH by a buffering agent is therefore an important function of preservation solutions.
Calcium
Under normal physiologic conditions the free calcium concentration difference between the intracellular and extracellular fluid is maintained by ATP-dependent transporters. During cold preservation, cellular ATP concentrations are low, leading to increased intracellular calcium. Preservation solutions therefore contain a low concentration of calcium. Experimentally calcium blockers in preservation solutions are shown to be effective in preventing activation of calcium-dependent processes such as calpain activation, an enzyme involved in the breakdown of the cytoskeleton.
Intracellular Enzymes and Organelles
Intracellular proteases are involved in the breakdown of proteins during preservation, most likely due to the absence of oxygen. Also, matrix metalloproteinases (MMPs) may be activated during cold preservation leading to detachment of endothelial cells from the underlying matrix. Hydroxyethyl starch present in UW solution may reduce this detrimental effect. Another relevant family of enzymes activated during cold preservation are apoptosis-related caspases. The release of mitochondrial proteins leads to endoplasmic reticulum stress and dysfunctional autophagy fluxes.
Reactive Oxygen Species
Reactive oxygen species (ROS) are generated by several processes in ischemic and postischemic reperfused organs. A well-studied generator of ROS is xanthine oxidase, which simultaneously produces hydrogen peroxide (H 2 O 2 ) and the superoxide anion (O 2 − ). The subsequent reduction of H 2 O 2 , catalyzed by iron, leads to hydroxyl radical formation. The infiltration of leukocytes in the graft after reperfusion also results in the production of superoxides (the respiratory burst). Lastly, mitochondrial dysfunction resulting from partial reduction of the respiratory chain is an important contributor to ROS formation after reperfusion. These ROS react rapidly with other intracellular molecules, causing severe damage to lipids, nucleic acids, and proteins during reperfusion. Some reports suggest that oxygen radicals are formed during reperfusion and during cold preservation. Modern preservation solutions contain scavengers or precursors for ATP production, which are shown to be effective in experimental research but less evident in clinical trials.
Clinical Use of Preservation Solutions
Worldwide, the UW solution and histidine-tryptophan-ketoglutarate (HTK) solution have been most commonly used since the early 1990s when two randomized controlled trials (RCTs) showed lower DGF rates compared with Euro-Collins’ solution. Registry data from the CTS suggests that at very long preservation times (beyond 24 hours) UW-stored kidneys have a reduced risk of graft loss compared with other preservation fluids. Registry data from the United Network for Organ Sharing (UNOS) show that kidney preservation in UW has improved graft survival independent of preservation time. The lack of adequately powered studies comparing Institut Georges Lopez-1 (IGL-1) solution, Celsior, or hyperosmolar citrate (HOC) against HTK or UW so far prevents definite conclusions regarding the superiority of any newer preservation fluids. From small published studies one could conclude that results are similar to UW or HTK. Most studies were conducted with relatively good quality donor kidneys. Globally UW and HTK are most predominant, with some countries using other preservation fluids that were developed locally being popular alternatives, see Tables 9.3 and 9.4 . There is retrospective evidence from the UK, where HOC is still used for the preservation of large numbers of kidneys, that HOC is an acceptable alternative to UW with a mean CIT of 17 hours.
Euro-Collins’ Solution (developed from Collins’ Solution) | EC | 1960s–1970s |
---|---|---|
Hyper-Osmolar Citrate (Marshall’s Solution) | HOC | 1970s |
Histidine-Tryptophan-Ketoglutarate | HTK | 1970s |
University of Wisconsin Solution | UW | 1980s |
Belzer Machine Perfusion Solution | MPS | 1980s |
Celsior Solution | Celsior | 1990s |
Institut Georges Lopez-1 Solution | IGL-1 | 1990s |
Component Type | Component | Celsior | EC | HOC | HTK | IGL-1 | UW | MPS |
---|---|---|---|---|---|---|---|---|
Colloids (mM) | HES | — | — | — | — | — | 0.25 | 0.25 |
PEG | — | — | — | — | 0.03 | — | — | |
Impermeants (mM) | Citrate | — | — | 80 | — | — | — | — |
Gluconate | — | — | — | — | — | — | 85 | |
Glucose | — | 195 | — | — | — | — | 10 | |
Histidine | 30 | — | — | 198 | — | — | — | |
Lactobionate | 80 | — | — | — | 100 | 100 | — | |
Mannitol | 60 | — | 185 | 38 | — | — | 30 | |
Raffinose | — | — | — | — | 30 | 30 | — | |
Ribose | — | — | — | — | — | — | 5 | |
Buffers (mM) | HEPES | — | — | — | — | — | — | 10 |
K2HPO4 | — | 15 | — | — | — | — | — | |
KH2PO4 | — | 43 | — | — | 25 | 25 | 25 | |
NaHCO3 | — | 10 | 10 | — | — | — | — | |
Electrolytes (mM) | Calcium | 0.25 | — | — | 0.0015 | — | — | 0.5 |
Chloride | 42 | 15 | — | 32 | 20 | 20 | 1 | |
Magnesium | 13 | — | 40 | 4 | 5 | 5 | 5 | |
Potassium | 15 | 115 | 84 | 9 | 25 | 120 | 25 | |
Sodium | 100 | 10 | 84 | 15 | 120 | 30 | 100 | |
ROS scavengers (mM) | Allopurinol | — | — | — | — | 1 | 1 | — |
Glutathione | 3 | — | — | — | 3 | 3 | — | |
Tryptophan | — | — | — | 2 | — | — | — | |
Substrates (mM) | Adenine | — | — | — | — | — | — | 5 |
Adenosine | — | — | — | — | 5 | 5 | — | |
Glutamate | 20 | — | — | — | — | — | — | |
Ketoglutarate | — | — | — | 1 | — | — | — | |
Osmolality (mOsm) | — | 255 | 406 | 400 | 310 | 320 | 320 | 300 |
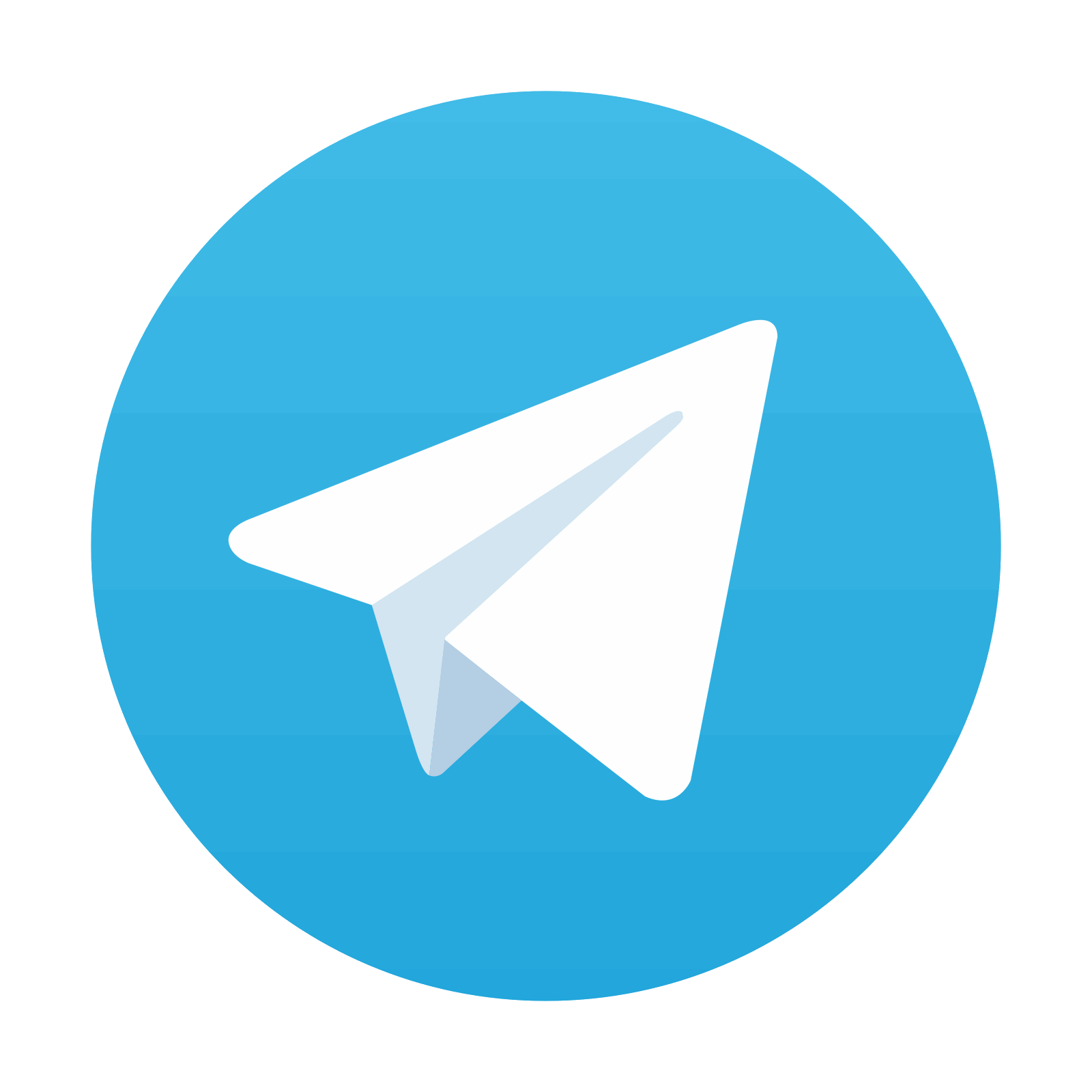
Stay updated, free articles. Join our Telegram channel

Full access? Get Clinical Tree
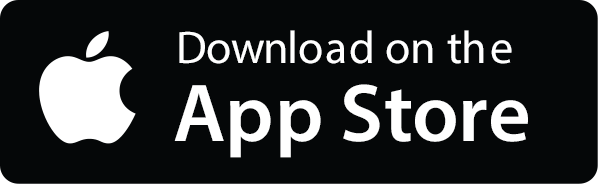
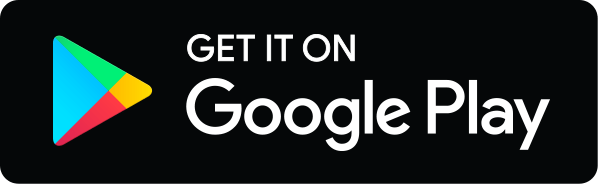
