Cardiorenal syndrome (CRS)
A complex pathophysiological disorder of the heart and kidneys whereby acute or chronic dysfunction in one organ may induce acute or chronic dysfunction in the other organ
CRS type I
(acute cardiorenal syndrome)
Abrupt worsening of cardiac function (e.g., ACS or ADHF) leading to AKI or acute worsening of kidney function
CRS type II
(chronic cardiorenal syndrome)
Chronic abnormalities in cardiac function (e.g., chronic congestive heart failure) contributing to progressive and permanent chronic kidney disease
CRS type III
(acute renocardiac syndrome)
AKI or abrupt worsening of kidney function contributing to acute cardiac disorder or decompensation
CRS type IV
(chronic renocardiac syndrome)
Chronic kidney disease (e.g., diabetic nephropathy) contributing to decreased cardiac function, cardiac hypertrophy, fibrosis, and/or increased risk of adverse cardiovascular events
CRS type V
(secondary cardiorenal syndrome)
Systemic condition (e.g., sepsis) contributing to both cardiac and/or kidney injury
6.2.1 Type I Cardiorenal Syndrome
This subtype is commonly encountered and is characterized by an acute cardiac event or disorder that precipitates AKI. The prototypical conditions contributing to type I CRS are acute decompensated heart failure (ADHF) and acute myocardial infarction (AMI).
The pathophysiological mechanisms contributing to AKI in ADHF and AMI are numerous and complex, however, likely involve alterations to myocardial performance, cardiac output, and systemic and central venous hemodynamics that compromise kidney perfusion and coupled with maladaptive and compensatory neuro-hormonal activation (i.e., activation of the sympathetic nervous system, increased activity of renin-angiotensin-aldosterone, and non-osmotic release of arginine vasopressin). These mechanisms will be further modified not only by the severity of the inciting event but also by preexisting susceptibilities including baseline cardiac and kidney function and presence of chronic kidney disease (CKD). Observational data have confirmed that persistent AKI in ADHF is more likely among those with baseline CKD and diminished renal reserve [2].
In both ADHF and AMI, the development of AKI is associated with worse outcomes, higher rehospitalization rates, and increased health-care costs [3, 4]. Moreover, there appears to be a biological gradient between the severity of AKI and risk of death [5, 6]. Among patients reperfused following AMI, those developing AKI tended to have higher plasma norepinephrine, B-natriuretic peptide, and interleukin-6 levels in the 2 weeks after reperfusion compared to those without AKI. Those with AKI show higher risk of in-hospital death and major adverse cardiac events, including greater changes in LV remodeling during recovery. Even small acute changes in serum creatinine modify the risk of death following AMI [6]. Among those developing AKI, greater risk of cardiovascular events such as congestive heart failure (CHF), recurrent AMI, and stroke and need for rehospitalization have been shown [6]. Moreover, patients with AMI complicated by AKI have increased risk of development of incident CKD and accelerated progression to ESKD [7].
6.2.2 Type III Cardiorenal Syndrome
This subtype is characterized by AKI that contributes to the development of acute cardiac injury and/or dysfunction (i.e., AMI, CHF, arrhythmias). While any episode of AKI may predispose to acute cardiac dysfunction and type III CRS, the most common conditions encountered include contrast-induced AKI (CI-AKI), drug-induced nephropathies, AKI after major noncardiac surgery, AKI after cardiac surgery, post-infectious glomerulonephritis, and rhabdomyolysis. The pathophysiological mechanisms of how AKI contributes to acute cardiac injury and/or dysfunction are incompletely understood. An episode of AKI may have effects that depend both on the severity and duration of AKI and that both directly and indirectly predispose to an acute cardiac event. Moreover, baseline patient susceptibility will modify the subsequent risk for cardiac events associated with AKI (i.e., preexisting risk factors and cardiac disease).
Experimental data suggest that cardiac injury may be directly induced by inflammatory mediators release, oxidative stress, apoptosis, and activation of neuroendocrine systems early after AKI [1, 8]. Likewise, AKI may be associated with physiological derangements (i.e., extracellular volume expansion, retention of uremic [cardiotoxic] compounds, metabolic acidosis, electrolyte abnormalities [i.e., hyperkalemia, hypocalcemia]), alterations to coronary vasoreactivity, and ventricular remodeling and fibrosis that indirectly exert negative effects on cardiac performance. AKI may also adversely impact cardiac function by contributing to alternations in drug pharmacokinetics and pharmacodynamics.
CI-AKI serves as a prototypical example of type III CRS. CI-AKI remains a leading cause of iatrogenic kidney injury following diagnostic and interventional procedures and portends adverse effects on prognosis, progression of CKD, and consumption of health resources. While AKI is most often attributable to the administration of radiocontrast media, additional susceptibilities and confounding factors in the population undergoing the procedure are also likely to be contributory (i.e., atheroembolic disease, kidney hypoperfusion, concomitant nephrotoxins). The reported incidence is highly variable depending on the population at risk being evaluated and the type of procedure performed (i.e., emergent, intravascular, type, and volume of contrast media). The natural history of CI-AKI in many patients may follow an asymptomatic rise in serum creatinine with early return to baseline, and these patients would not be expected to fulfill the criteria for type III CRS. However, in an estimated 0.2–1.1 %, AKI progresses to require the initiation of renal replacement therapy (RRT) [9, 10].
In these patients, AKI may be associated with volume overload, retention of uremic solutes, CHF, pulmonary edema, and cardiac arrhythmias. Several factors have been found to independently predict development of more severe AKI after contrast media including older age, preexisting CKD, diabetes mellitus, cerebral vascular disease, heart failure, and volume/dose of contrast media. However, the difficulty in evaluating the epidemiology of type III CRS attributable to CI-AKI or AKI from other causes is that few studies have specifically reported the temporal occurrence of cardiovascular events following AKI.
6.3 The Kidney and the Lung
Kidney and lung injury are highly prevalent in critical illness. These two organ systems are intimately interconnected. Injury and/or dysfunction in either or both of these organ systems can directly incite or exacerbate injury and/or impairment in the other.
6.3.1 Impact of AKI on Lung Function
The loss of metabolic/fluid homeostasis and excretory function characteristic of AKI is associated with the retention of metabolic waste products (i.e., uremic toxins), nonvolatile acids, and the expansion of extracellular volume. This decrement in kidney function can precipitate clinically important and adverse physiological consequences on the normal function of numerous organ systems, in particular the lung [1]. The accumulation of uremic compounds is known to contribute to lung inflammation and injury and has been termed uremic pneumonitis. However, this complication of AKI is rarely seen due to earlier initiation and more intensive application of RRT [11]. Metabolic derangement in AKI, such as abnormalities in serum phosphate and calcium level and metabolic acidosis, may also contribute to respiratory muscle weakness and dysfunction [12]. Perhaps the most common life-threatening pulmonary complication associated with AKI is alveolar edema. Expansion of extracellular volume can contribute to increased pulmonary capillary hydrostatic pressure. This coupled with alterations to pulmonary microvascular permeability and reduced serum oncotic pressure can predispose to rapid increases in extravascular lung water [13]. AKI can also contribute to lowering the threshold for accumulation of extravascular lung water [14] along with impaired clearance of alveolar fluid once present. Indeed, lung injury in AKI can occur in the absence of overt volume overload [15]. This is due, in part, to the downregulation of epithelial sodium transporters (ENaC), sodium-potassium adenosine triphosphatases (Na-K-ATPase), and aquaporins at the alveolar-capillary barrier. In further support of the hypothesis that lung injury in AKI represents more than alveolar edema, experimental models of ischemic-/reperfusion-induced AKI have shown lung injury that is characterized by not only pulmonary vascular congestion and interstitial edema but also focal alveolar hemorrhage and inflammatory cell infiltrate [16]. Indeed, the systemic inflammation incited by AKI, including the release and reduced clearance of proinflammatory mediators, is an important mechanism contributing to acute lung injury. Moreover, the magnitude of AKI, both in terms of severity and duration, can also modify the intensity of lung injury.
Naturally, this organ crosstalk and associated clinical complications may be aggravated in critical illness due to concurrent widespread systemic inflammation (i.e., sepsis, major trauma) and diminished baseline physiological reserve due to preexisting chronic lung, cardiac, or kidney disease and in response to resuscitation (i.e., large-volume resuscitation). Among patients receiving mechanical ventilation, the development of AKI has been associated with impaired or delayed weaning, higher likelihood of tracheostomy, and prolongation of ICU stay [17]. Those patients developing lung injury and respiratory failure necessitating mechanical ventilation, concurrent with or following an episode of AKI, have worse outcome and increased risk of mortality [18, 19].
6.3.2 Impact of Lung Injury on the Kidney
Lung injury, such as acute respiratory distress syndrome (ARDS), can contribute to downstream kidney injury and dysfunction through a number of mechanisms including impaired gas exchange, systemic and regional hemodynamic alterations, systemic inflammation, and the application of mechanical ventilation (Table 6.2).
Table 6.2
Potential mechanisms of impaired kidney function associated with mechanical ventilation
Alterations to cardiovascular function | Reduced cardiac output |
Reduced renal blood flow | |
Altered distribution of intrarenal blood flow | |
Elevated inferior vena cava and renal vein pressure | |
Alterations to neuro-hormonal function | Sympathetic nervous system activation |
Renin-angiotensin-aldosterone system stimulation | |
Reduced atrial natriuretic peptide secretion | |
Increased (non-osmotic) arginine vasopressin secretion | |
Exaggerated effects of mechanical ventilation | Intravascular volume depletion |
Impaired baseline myocardial performance | |
Alterations to pulmonary compliance | |
Prior chronic kidney disease | |
Prior chronic pulmonary disease |
Abnormalities in gas exchange are common among critically ill patients with lung injury. These patients often receive supplemental oxygen, noninvasive ventilatory support, or invasive mechanical ventilation when respiratory failure ensues, with the aim of correcting hypoxemia and restoring near-normal gas exchange. However, this is often challenging or not possible, and many patients may have residual hypoxemia or hypercapnea in the setting of ARDS and lung-protective ventilation and/or permission hypercapnea. The exact mechanisms by which hypoxemia contributes to AKI are incompletely understood and, however, are likely multifactorial and relate to altered cardiovascular function, renal microcirculatory dysfunction, neuro-hormonal activation, and circulating systemic inflammatory mediators [20]. Small clinical studies have suggested that hypoxemia may impair renal autoregulation and glomerular filtration rate (GFR) and contribute to sodium and water retention, while reversal of hypoxemia may improve renal blood flow (RBF) [21, 22]. Hypercapnea has also been associated with impaired kidney function, attributed to alterations in RBF and renovascular resistance; however, experimental data have been inconsistent [23]. Further, acute hypercapnea can also contribute to or worsen existing acidemia. The combined impact of hypoxemia and hypercapnea may act synergistically to impair kidney function [24].
Lung injury may be the result of primary lung disease (i.e., aspiration, contusion) or a systemic process (i.e., pancreatitis, sepsis); however, the application of mechanical ventilation is well recognized as a potentially important precipitant or exacerbating factor in lung injury. Ventilator-induced lung injury (VILI) is attributable to the use of excessive end-inspiratory pressures and volumes coupled with the added effects of barotrauma, atelect-trauma, and biotrauma (Table 6.3). The mechanical disruption of the alveolar-capillary barrier from excessive pressure-volume loading during positive pressure ventilation can induce the release of local inflammatory mediators into the systemic circulation [25]. This inflammation due to VILI can contribute to downstream end-organ injury, including AKI [26]. In a murine model of lung injury, the application of injurious MV was associated with increased expression of IL-6 in kidney tissue, increased evidence of renal tubular apoptosis, and impaired kidney function [27, 28].
Table 6.3
Mechanisms of ventilator-induced lung injury (VILI)
Volutrauma | Ventilation with excessive tidal volume or end-expiratory volumes |
Barotrauma | Ventilation with excessive end-inspiratory or plateau pressures |
Atelect-trauma | Ventilation below the lower inflection point on the pressure-volume curve with cyclic opening and closing of alveoli |
Biotrauma | Local and systemic release of inflammatory mediators in response to mechanical stress and disruption of alveoli |
6.4 The Kidney in the Abdominal Compartment
The kidneys are encapsulated organs, located in the retroperitoneal space of the abdominal compartment. Therefore, alterations in the abdomen can seriously disturb kidney function. This section discusses the impact of elevated intra-abdominal pressure (IAP), deranged splanchnic and renal hemodynamics, and abdominal organ dysfunction on renal function in critically ill patients.
6.4.1 Intra-abdominal Hypertension and Abdominal Compartment Syndrome
There has been increasing focus on the detrimental effects of elevated IAP in AKI [29]. IAP is normally between 5 and 7 mmHg in healthy individuals and ≤10 mmHg in critically ill adults, measured supine at end-expiration, zeroed at the level where the midaxillary line crosses the iliac crest [30]. By consensus, intra-abdominal hypertension (IAH) is defined as a sustained increase in IAP ≥12 mmHg, and abdominal compartment syndrome (ACS) as a sustained increase >20 mmHg with new organ dysfunction/failure [30]. The most important risk factors for these conditions are shock/hypotension, resuscitation with a large amount of fluids, and worsening respiratory status [31]. Therefore, it should not be surprising that IAH is common among critically ill patients and associated with worse outcome [32]. However, it is less clear whether the relationship between IAH and organ dysfunction represents cause and effect and if prevention/treatment of IAH might improve organ function and prognosis.
6.4.2 Elevated Intra-abdominal Pressure and Renal Function
Elevated IAP influences renal function in different ways. First, IAH might lead to a significant decrease in RBF as renal perfusion pressure equals mean arterial pressure minus IAP [33]. Further, higher intrarenal vascular resistance (organ compression) shunts blood away from the kidneys. This is of particular concern for the renal medulla, which receives only ~12 % of the RBF, exacerbated by neurohumoral activation [34]. Indeed, AKI through ischemic tubular necrosis is probably the most common complication in the ICU necessitating RRT. Second, the combination of systemic venous congestion and elevated IAP decreases the glomerular filtration gradient [35]. Because the kidney is an encapsulated organ, a pressure rise in the venous system translates into a higher renal interstitial and Bowman’s capsular pressure, directly impeding glomerular filtration [36, 37]. Intriguingly, in advanced heart failure – presumably because of low renal perfusion – the kidneys are extremely sensitive to even small elevations in IAP (8–10 mmHg) [38]. Moreover, decreasing IAP in such cases, through ultrafiltration or paracentesis, can dramatically improve renal function [38]. Extrapolating these findings to the general ICU population, recent evidence suggests that a comprehensive management strategy with appropriate use of an open abdomen in patients at risk significantly improves survival from IAH/ACS [39].
6.4.3 Gut Microbiota and the Intestinal Barrier Function
A new area of research is the role of gut microbiota in AKI. It has been clearly established that bacterial fermentation processes in the large intestines are an important source of tightly protein-bound toxins such as p-cresyl sulfate and indoxyl sulfate [40]. Because of this protein binding, such toxins are difficult to clear from the circulation, even by means of hemodialysis [41]. They may accelerate kidney dysfunction, and plasma levels are correlated to all-cause mortality [42, 43]. This offers a strong rationale for targeting gut microbiota and toxin production in the bowel compartment with future therapies.
In normal circumstances, the gut has an important barrier function, preventing entrance of toxins and microorganisms into the systemic circulation. However, especially when abdominal perfusion is impaired, like in advanced heart failure or patients with IAH, this function might become compromised [44]. Indeed, it has been shown that the intestinal morphology, permeability, and function are substantially altered in heart failure [45, 46]. Consequently, leakage of lipopolysaccharides in the systemic circulation may cause further hemodynamic compromise leading to a detrimental vicious cycle [44, 47]. As the ICU clusters, the most vulnerable patients with regard to hemodynamic compromise, kidney and organ dysfunction, this is likely an underrecognized problem and should be an area of further research. Only recently, IAP has been identified as a potential missing link in patients with cardiorenal syndrome, and the term cardio-abdominal-renal syndrome (CARS) was coined [44].
6.4.4 Hepatorenal Syndrome
AKI is a fearsome complication in patients with decompensating liver cirrhosis, where it occurs in ~20 %, and is associated with poor outcome [48]. Patients with cirrhosis are susceptible to developing AKI because of the progressive vasodilatory state and reduced effective blood volume. Hepatorenal syndrome is initiated by portal hypertension and may be triggered by bacterial infections, nonbacterial systemic inflammatory reactions, excessive diuresis, gastrointestinal hemorrhage, diarrhea, nephrotoxic agents, or IAH [49]. Orthotopic liver transplantation is the best current treatment and leads to a gradual recovery of renal function in the vast majority of patients.
6.4.5 Conclusions
The kidneys are well-perfused organs, located inside the retroperitoneal space of the abdomen. Elevated IAP may seriously disrupt systemic and renal hemodynamics, which might cause renal dysfunction and loss of intestinal barrier function with subsequent entry of toxins into the systemic circulation. A more thorough understanding of kidney-organ interactions in the abdominal compartment may hopefully lead to new therapeutic targets to better preserve renal function in critically ill patients. Within this regard it is important to consider IAP as a missing link in patients with congestive heart failure developing worsening kidney function. This condition has been termed as CARS.
< div class='tao-gold-member'>
Only gold members can continue reading. Log In or Register a > to continue
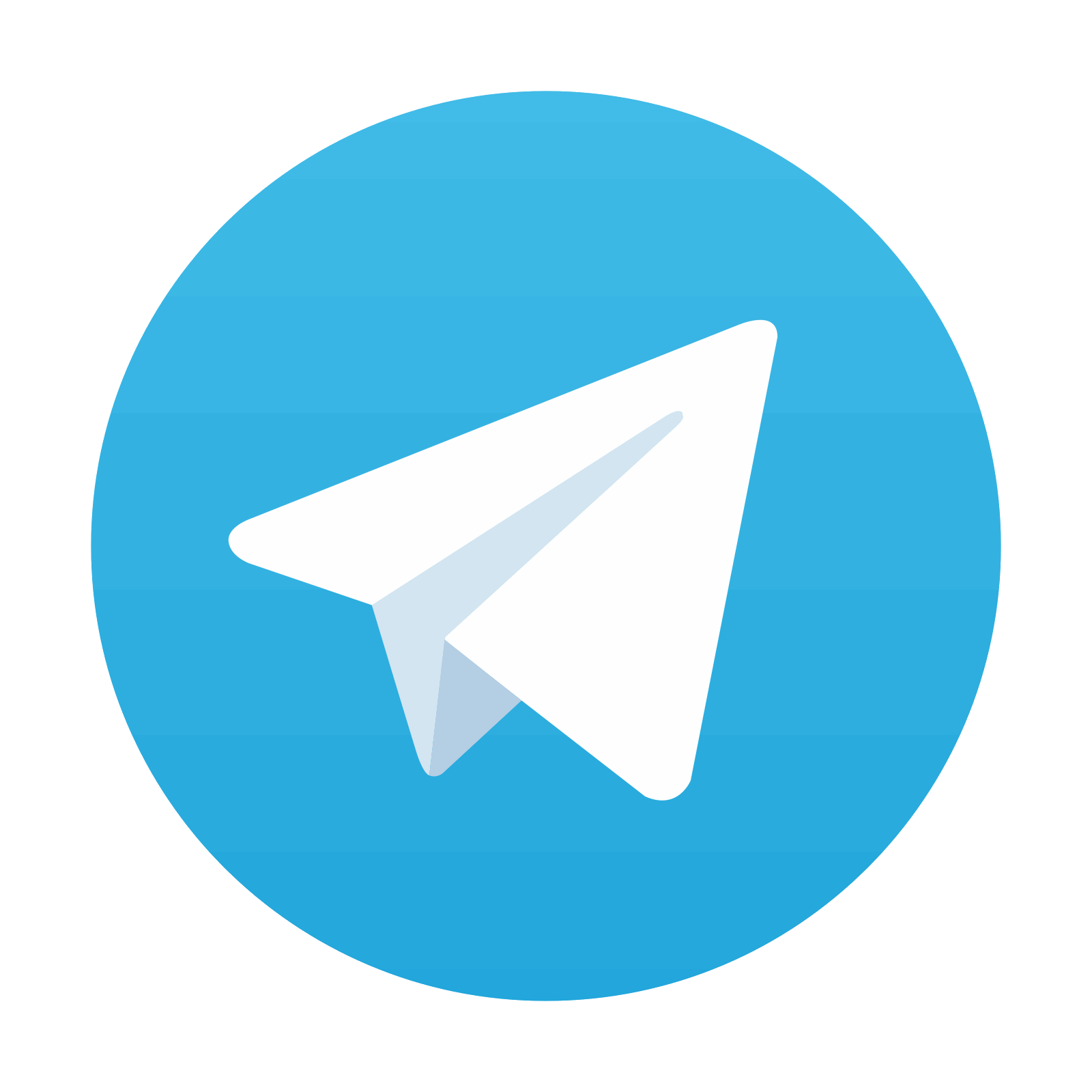
Stay updated, free articles. Join our Telegram channel

Full access? Get Clinical Tree
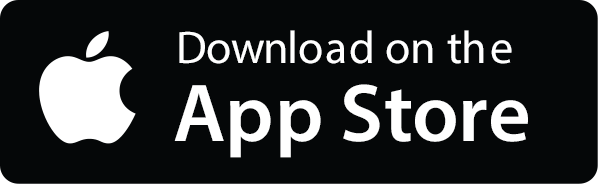
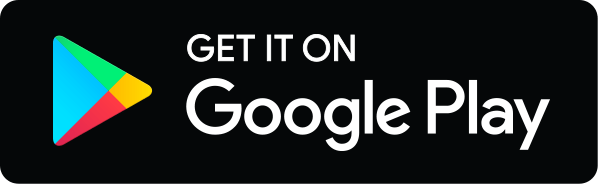