Abstract
Pediatric nephrology has rapidly evolved over the last several decades, with major advances in the pathophysiology, genetics, diagnosis, and treatment of children with kidney diseases. With refined therapeutics, increasing survival of chronic illnesses, and technological advances in hospital and critical care medicine also come the growing awareness of the increased risk of acute kidney injury in hospitalized children. The economic and social burden of chronic kidney disease (CKD) remains substantial in children, although much progress has been made in identifying factors associated with CKD progression and better management of various comorbid conditions. The epidemiology, diagnosis, and management of childhood hypertension have changed greatly in the last decade, in part fueled by the rising obesity epidemic.
Keywords
pediatric nephrology, kidney, hypertension, kidney development, acute kidney injury (AKI), glomerular filtration rate (GFR), chronic kidney disease (CKD)
Kidney Development and Maturation
Nephrogenesis begins in utero at approximately 5 to 6 weeks’ gestation and continues until nephron formation is complete at approximately 36 weeks’ gestation. Fetal urine production commences before the end of the first trimester and by the third trimester becomes the primary component of the amniotic fluid, which is essential for normal pulmonary development. Although the full-term newborn has the same number of nephrons as an adult, the glomeruli and tubules of the infant kidney are relatively immature. The neonatal tubule has a limited ability to concentrate or dilute the urine in response to different environmental or dietary conditions. Furthermore, the ability of the neonatal proximal tubule to reabsorb filtered is less than that of adults. As a result, the average serum
concentration in preterm infants (16 to 20 mEq/L) is lower than in term infants (19 to 21 mEq/L), and older children and adults (24 to 28 mEq/L). Newborns also often have higher serum potassium concentrations (6.5 to 7.0 mEq/L) than older children as a result of a decreased glomerular filtration rate (GFR) and a relative insensitivity of the neonatal tubule to aldosterone.
The GFR of the newborn kidney is only around 20 mL/min per 1.73 m 2 in the first few days after birth, rising to approximately 40 mL/min per 1.73 m 2 near the end of the first week of life, and gradually reaching adult levels of 100 to 130 mL/min per 1.73 m 2 by 2 years of age. The changes in GFR that occur after birth are the result of increased cardiac output and mean arterial blood pressure, a decrease in renal vascular resistance, and an increased surface area available for glomerular filtration. Concomitantly, blood flow is redistributed from the cortical-juxtamedullary glomeruli, which are larger but fewer in number than the more numerous glomeruli in the cortex.
In term neonates, the low GFR results in a serum creatinine (sCr) level that remains similar to the mother’s for the first 24 to 48 hours of life and gradually settles to approximately 0.4 mg/dL at the fifth to seventh day of life. In preterm infants born at 28 to 30 weeks’ gestation, the GFR is only approximately 12 to 13 mL/min per 1.73 m 2 within the first few days after birth, and the sCr may take longer to normalize. The full-term newborn kidney measures 4 to 5 cm in length and continues to grow until reaching 10 to 12 cm by adolescence. Although the glomeruli do grow in size, most of this renal parenchymal expansion results from tubular growth and maturation and from an increased volume of the tubulointerstitial compartment.
Acute Kidney Injury
Acute kidney injury (AKI) is defined as a sudden decrease in kidney function that compromises the normal regulation of fluid, electrolyte, and acid-base homeostasis. The term AKI has largely replaced the term acute renal failure (ARF). The reference to AKI emphasizes the continuum of kidney dysfunction without overt organ failure, which is clinically relevant and linked to morbidity and mortality. In practical terms, AKI is characterized by a reduction of the GFR, which results in an abrupt increase in the concentrations of sCr and blood urea nitrogen (BUN) and/or a sustained reduction in urine output. Despite its widespread use, sCr is a poor marker of kidney function in the setting of AKI, and it best correlates with GFR in steady state. In fact, patients with acute, severe AKI may still show a normal or only mildly elevated sCr concentration due to insufficient time for creatinine accumulation to occur in the serum and relatively low creatinine generation. An emerging area of research is the study of novel biomarkers useful in the prediction of AKI. Biomarkers under investigation include urinary neutrophil gelatinase-associated lipocalin (NGAL), urinary cystatin C, urinary kidney injury molecule (KIM)-1, urinary interleukin (IL)-18, and urinary liver-type fatty acid binding protein (L-FABP), although their use has not yet become part of routine clinical practice.
No current single consensus definition of pediatric AKI exists, which has resulted in substantial variability in the reported incidence, morbidity, and mortality estimates. The three most widespread definitions are the Risk Injury, Failure, Loss and End-stage (RIFLE); AKI network (AKIN); and Kidney Disease: Improving Global Outcomes (KDIGO) classifications ( Table 48.1 ). The RIFLE criteria were developed by an international consensus panel in 2004 and were intended for use in critically ill adults. RIFLE classifies AKI into five distinct categories based upon the magnitude and direction of change in creatinine, urine output, and duration of renal replacement therapy (RRT): (R) Risk, (I) Injury, (F) Failure, (L) Loss of kidney function, and (E) End-stage renal disease. This classification system was subsequently modified for the pediatric population (pRIFLE) by the use of an estimated creatinine clearance based on the original Schwartz formula to quantify the change in GFR (rather than absolute changes in sCr used in the adult version). In addition, all children with an estimated creatinine clearance less than 35 mL/min per 1.73 m 2 are placed in the “pRIFLE-F” category (kidney failure class) instead of waiting for the sCr concentration to reach 4 mg/dL as proposed by the adult criteria.
pRIFLE | AKIN | KDIGO | |||
---|---|---|---|---|---|
Stage | Criteria | Stage | Criteria | Stage | Criteria |
Risk (R) | eCrCl ↓ 25% UOP <0.5 mL/kg/h × 8 h | 1 | ↑ SCr ≥0.3 mg/dL or ↑ SCr ≥1.5–2× a UOP <0.5 mL/kg/h × 6 h | 1 | ↑ SCr ≥0.3 mg/dL or ↑ SCr ≥1.5–2× b UOP <0.5 mL/kg/h × 8 h |
Injury (I) | eCrCl ↓ 50% UOP <0.5 mL/kg/h × 16 h | 2 | ↑ SCr ≥2–3× UOP <0.5 mL/kg/h × 12 h | 2 | ↑ SCr ≥2–3× UOP <0.5 mL/kg/h × 16 h |
Failure (F) | eCrCl ↓ 75% or CrCl <35 mL/min/1.73 m 2 UOP <0.5 mL/kg/h × 24 h or anuria for 12 h | 3 | ↑ SCr ≥3–4× or SCr >4 (within 0.5 in 48 h) or RRT initiated UOP <0.5 mL/kg/h × 24 h or anuria × 12 h | 3 | ↑ SCr ≥3–4× or SCr >4 (and meets criteria for AKI) or RRT initiated or eGFR <35 in patients <18 yo UOP <0.5 mL/kg/h × 24 h or anuria × 12 h |
Loss (L) | Failure >4 weeks | N/A | N/A | ||
End-stage kidney disease (E) | Failure >3 months | N/A | N/A |
The AKIN criteria are based on changes in sCr; AKIN stage 1 describes patients who experience a ≥0.3-mg/dL (26.4-µM) increase in sCr over a 48-hour period. Although AKIN was not adjusted for children, it has been used in research in pediatric AKI, as has the most recent 2012 KDIGO definition, which uses a more flexible timeline than AKIN and has a specific modification for children (see Table 48.1 ). The KDIGO definition and staging criteria include a 0.3-mg/dL increase in sCr over 48 hours, or a urine volume ≤0.5 mg/kg per hour for 6 hours. The diagnostic inclusion criteria for an sCr elevation ≥1.5 times baseline within the prior 7 days allows for the inclusion of patients with late-onset AKI.
Urine volume in AKI is variable: patients may be anuric, oliguric, and in some cases (particularly in neonates) polyuric. AKI develops over a period of hours to days, whereas chronic kidney disease (CKD) develops over months to years. Short stature, renal osteodystrophy, delayed puberty, normocytic anemia, and hyperparathyroidism all suggest long-standing and advanced CKD rather than AKI. However, at a single point in time of an acute clinical presentation, it may be difficult to differentiate AKI from CKD without imaging studies, extensive laboratory testing for the aforementioned complications, and possibly a kidney biopsy.
Kidney function becomes impaired when adequate blood supply and oxygenation, parenchymal integrity, and/or patency of the urinary collecting system are interrupted. Consequently, AKI can be viewed as caused by prerenal, intrinsic kidney, or postrenal factors, although substantial overlap and multifactorial etiologies can exist, particularly in hospitalized children. The likelihood of recovery from AKI depends in part on the presence or absence of urine output, the quantity of urine output, the duration of anuria, and the underlying cause and severity of kidney injury. Quantifying the urine output is essential, as this predicts the clinical course and may aid in identifying the underlying insult. Oliguria is defined as a urine output less than 1 mL/kg per hour in infants and young children and less than 0.5 mL/kg per hour in older children. Patients with nonoliguric AKI have lower complication rates and higher survival rates than those with anuric or oliguric AKI. Causes of nonoliguric AKI include acute interstitial nephritis (AIN) or nephrotoxic kidney insults, including aminoglycoside nephrotoxicity. In contrast, AKI in children with kidney hypoperfusion injury, acute glomerulonephritis, or hemolytic uremic syndrome (HUS) is usually associated with oligoanuria. In infants, oliguric AKI normally occurs as a result of either renovascular accidents (renal artery or vein thrombosis) or ischemic acute tubular necrosis (ATN) from shock.
Prerenal AKI results from kidney hypoperfusion caused by intravascular volume contraction, decreased effective circulating blood volume, or altered intrarenal hemodynamics. Intravascular volume contraction can be seen with volume depletion, acute blood loss, or extravascular accumulation of fluid (so-called third spacing of fluid), the latter most often in patients with the systemic inflammatory response syndrome (SIRS) or hypoalbuminemia. Decreased effective blood circulation occurs when the true blood volume is normal or increased, but renal perfusion is decreased, such as in left-sided heart failure, cardiac tamponade, or hepatorenal syndrome. Intrarenal afferent arteriolar vasoconstriction develops in hepatorenal syndrome, calcineurin inhibitor toxicity, and nonsteroidal antiinflammatory drug (NSAID) use; intrarenal efferent arteriolar vasodilation occurs with the use of angiotensin-converting enzyme (ACE) inhibitors and angiotensin receptor blockers. Kidney dysfunction in prerenal AKI is reversible by restoration of kidney perfusion, although severe and/or prolonged kidney hypoperfusion often leads to tubular injury and intrinsic AKI.
Intrinsic kidney injury or pathology may occur at the level of the kidney vasculature, tubules, interstitium, or glomeruli. The basic mechanisms of such injuries include hypoperfusion and ischemic cell damage, toxin-mediated cell injury, and inflammation. Vascular injury may occur in large vessels (e.g., renal artery or vein thrombosis) or in the microvasculature (e.g., HUS or thrombotic thrombocytopenic purpura, TTP). Direct tubular injury, also known as ATN, is the end result of either ischemic or toxin-mediated damage to the tubules. Ischemia-induced ATN is the result of prolonged or severe kidney hypoperfusion. Toxin-mediated intrinsic ATN may be secondary to medications (aminoglycosides, amphotericin, acyclovir, cisplatin, iodinated radiocontrast agents), endogenous toxins (e.g., heme pigments from myoglobin and hemoglobin from rhabdomyolysis or hemolysis), and exogenous toxins (e.g., mercury, crystal formation from ethylene glycol, and methanol poisoning).
Inflammatory injury in the form of AIN as a cause of intrinsic AKI most often occurs in the setting of medication exposures. Medications commonly associated with interstitial nephritis include extended-spectrum penicillins, NSAIDs, sulfonamides, and rifampin. AIN is also associated with infections, systemic diseases, tumor infiltrates, or genetic conditions. Acute glomerulonephritis is more common in school-age children but is rare before 2 years of age. Postinfectious glomerulonephritis is the most common cause of acute glomerulonephritis and AKI in childhood; however, many other conditions produce a glomerulonephritic injury as well, including antiglomerular basement membrane (anti-GBM) antibody disease, antineutrophil cytoplasmic antibody (ANCA) disease, lupus nephritis, IgA nephropathy, Henoch-Schönlein purpura (HSP) nephritis, and membranoproliferative glomerulonephritis (MPGN).
Despite the fact that congenital obstructive uropathies are among the most frequent causes of CKD in children, AKI itself is rarely the result of postrenal obstruction, except when this occurs in a solitary kidney either from aplasia, nephrectomy, or transplantation. More commonly in children with two functioning kidneys, postrenal AKI occurs in the setting of complete urethral or bladder neck obstruction, or in the unusual circumstance of bilateral ureteric obstruction. A variety of conditions can cause such unilateral or bilateral obstruction and subsequently lead to AKI, including calculi, ureteral blood clots, retroperitoneal fibrosis, neurogenic bladder, bladder or pelvic tumors, and urethral stricture.
General measures to prevent AKI include restoration of intravascular volume, avoidance of hypotension and renal ischemia by providing inotropic support in critically ill children following volume repletion, and careful readjustment of nephrotoxic medications based on close monitoring of drug levels and kidney function. Whereas several pharmacologic agents including mannitol, loop diuretics, low-dose dopamine, fenoldopam, and N -acetylcysteine have been studied in children with AKI (with no convincing evidence of benefit), none are routinely recommended to prevent AKI or its progression.
Management of AKI includes relief of obstruction if present, judicious fluid administration to maintain euvolemia, treatment of electrolyte abnormalities including hyperkalemia, metabolic acidosis, hypocalcemia, hyperphosphatemia, and blood pressure management. Loop diuretics are often used to induce diuresis in the setting of volume overload or for the treatment of hyperkalemia, but they have not been shown to prevent AKI or to substantially alter the natural history of AKI other than enhancing urine output in the nephrons that remain functional. Restriction of sodium, potassium, and phosphate intake may be indicated, as may the use of potassium-binding agents to treat for hyperkalemia and oral phosphate binders for the management of hyperphosphatemia. Metabolic acidosis should be corrected carefully; the exchange of protein-bound hydrogen ions with calcium can result in a decrease of the available ionized calcium and result in tetany. Frequent dose adjustment or elimination of potentially nephrotoxic medications is necessary when the GFR is significantly compromised, and a multidisciplinary approach among intensivists, nephrologists, and specialized pharmacists may be helpful. Polyuria and tubular dysfunction (hypokalemia, hypophosphatemia, hypomagnesemia) can be noted in the recovery phase of AKI due to a lag in the tubular reabsorptive capacity, and careful management of fluids and electrolytes during the diuretic phase is essential.
Kidney replacement therapy (KRT) is indicated when conservative measures fail. Frequent indications include fluid overload (10% to 20% excess), hyperkalemia or severe acidosis unresponsive to pharmacologic therapy, uremia (typically marked by a BUN >100 mg/dL [30 mM] or symptoms), or an inability to provide adequate nutrition. Volume overload is increasingly recognized as a predictor of unfavorable outcomes, with an overall trend in many centers toward earlier initiation of KRT. Modalities used in AKI include peritoneal dialysis (PD), hemodialysis (HD), and continuous renal replacement therapy (CRRT); the choice is dictated largely by the patient’s clinical status, as well as by the availability of equipment and the center expertise. Peritoneal dialysis is advantageous in neonates and younger children and in resource-limited countries, with no requirements for systemic/regional anticoagulation, vascular access, or specialized equipment or personnel. Hemodialysis offers the advantage of being able to rapidly correct fluid or electrolyte imbalances but does require patients to tolerate a large extracorporeal volume through an adequate vascular access and mandates specially trained personnel. Finally, where equipment and trained personnel exist, CRRT may be preferred in children with multisystem organ dysfunction or hemodynamic instability; it permits gentler fluid removal rates with less dynamic fluid shifts than HD while allowing full enteral or parenteral nutrition.
Chronic Kidney Disease
CKD is characterized by functional or structural damage to the kidneys and/or a decrease in GFR to less than 60 mL/min per 1.73 m 2 for ≥3 months. Importantly, the GFR may be normal or near normal in the early stages of CKD ( Table 48.2 ) when intervention is most likely to successfully prevent or slow progression and adequately treat accompanying comorbidities. Although diabetes and hypertension are the disorders that account for the vast majority of CKD cases in adult patients, approximately two thirds of childhood CKD is attributed to congenital anomalies of the kidney and urinary tract (CAKUT), including renal hypoplasia/dysplasia and obstructive uropathy (e.g., posterior urethral valves). Other common causes include focal segmental glomerulosclerosis (FSGS), chronic glomerulonephritis (lupus nephritis, IgA nephropathy, HSP, or MPGN), ciliopathies (autosomal recessive polycystic kidney disease, nephronophthisis), and HUS. Renovascular accidents, Alport syndrome, congenital nephrotic syndrome, primary hyperoxaluria, and cystinosis all remain rare but important causes of CKD in childhood.
GFR Marker | GFR Equation |
---|---|
SCr by alkaline picrate (Jaffe) | Schwartz equation a eGFR (mL/min/1.73 m 2 ) = k × Ht (cm)/Scr |
Counahan-Barratt equation b eGFR (mL/min/1.73 m 2 ) = 0.43 × Ht (cm)/Scr | |
SCr by IDMS traceable enzymatic methods | CKiD bedside equation c eGFR (mL/min/1.73 m 2 ) = 0.413 × Ht (cm)/Scr |
CysC | CKiD cystC equation d eGFR (mL/min/1.73 m 2 ) = 70.69 (CystC) −0.931 |
Zappitelli et al. cystC equation e eGFR (mL/min/1.73 m 2 ) = 75.94 (CystC) −1.170 | |
Filler and Lepage cystC equation f eGFR (mL/min/1.73 m 2 ) = 91.62 (CystC) −1.129 | |
SCr + CysC | CKiD multivariable equation g eGFR (mL/min/1.73 m 2 ) = 39.8 × Ht (m)/Scr) 0.456 (1.8/CystC) 0.418 (30/BUN) 0.079 (1.076) male (Ht(m)/1.4) 0.179 |
Zappitelli et al. equation h eGFR (mL/min/1.73 m 2 ) = 43.82 (1/CystC) 0.635 (1/Scr) 0.547 (1.35 height ) | |
Bouvet et al. equation i eGFR (mL/min/1.73 m 2 ) = 63.2 (1.2/CystC)[(96/88.4)/(Scr)] 0.35 (weight/45) 0.30 (age/14) 0.40 | |
Comments | |
a k is a constant, directly proportional to the muscle component of body, and varies with age. The value for k is 0.33 in premature infants through the first year of life, 0.45 for term infants through the first year of life, 0.55 in children and adolescent girls, and 0.7 in adolescent boys. Formula developed against measured inulin GFR [PMID 951142] (Schwartz et al., 1976) | |
b Jaffe reaction after adsorption onto ion exchange to remove noncreatinine chromogens. Recommendations for subtracting 0.14 mg/dL for automated Jaffe creatinine. Formula developed against measured Cr-EDTA GFR [PMID: 1008594] (Counahan et al., 1976) | |
c Formula developed against measured iohexol GFR [PMID: 19158356] (Schwartz et al., 2009) | |
d CystC by PENIA. Formula developed against single injection iohexol [PMID: 22622496] (Schwartz et al., 2012) | |
e Formula developed against cold iothalamate [PMID: 16860187] (Zappitelli et al., 2006) | |
f CystC by PENIA. Formula developed against single injection Tc-DTPA [PMID:12920638] (Filler and Lepage, 2003) | |
g Serum creatinine by IDMS traceable enzymatic method. CystC by PENIA. Formula developed against single injection iohexol [PMID: 22622496] (Schwartz et al., 2012) | |
h Serum creatinine by enzymatic creatinine. CystC by PENIA. Formula developed against nonradioactive iothalamate steady-state method [PMID: 16860187] (Zappitelli et al., 2006) | |
i Serum creatinine by noncompensated Jaffe method. CystC by PENIA. Formula developed against single injection 51 Cr-EDTA [PMID: 16794818] (Bouvet et al., 2006) |
The determination of GFR is integral to the diagnosis of CKD. The original Schwartz formula to estimate GFR in children was developed in the mid-1970s using sCr, height, and an empiric constant for age/gender. However, the sCr assay has changed from the Jaffe chromogen reaction, the assay used for the original formula, to the enzymatic method now used in most centers, requiring a refinement of pediatric GFR estimating formulas. The Schwartz estimating equation was, in turn, updated in 2009 based on results from the prospective, observational multicenter Chronic Kidney Disease in Children (CKiD) study, in which GFR was measured through the plasma disappearance of iohexol. The estimating formula is as follows:
eGFR = 39.1 × [ height / SCr ] 0.516 × [ 1.8 / cystatin C ] 0.294 × [ 30 / BUN ] 0.169 × [ 1.099 ] Male × height / 1.4 ] 0.188
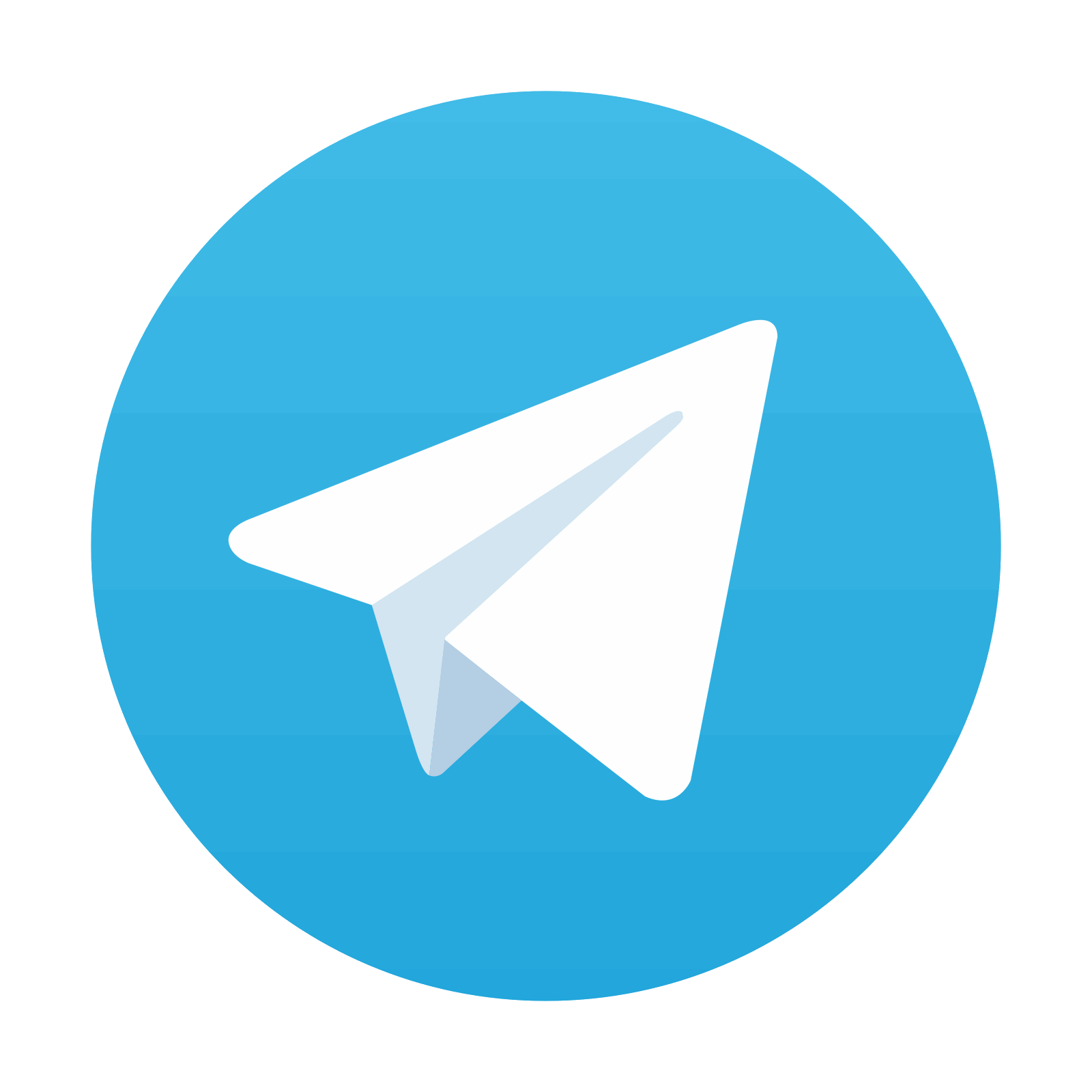
Stay updated, free articles. Join our Telegram channel

Full access? Get Clinical Tree
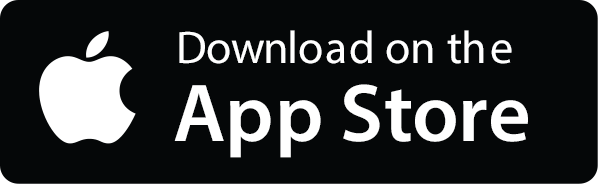
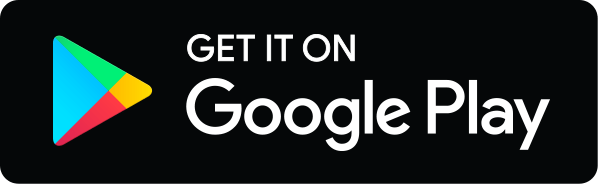