1.1
Anatomy of an endoscope
Jean Marc Canard
Summary
Introduction 1
- 1
The control handle 1
- 2
Main insertion tube 2
- 3
Bending section 3
- 4
The optical head 3
- 5
Generator connecting cable 3
Conclusion 4
- •
Modern endoscopes are complex and expensive instruments and, for optimum performance and safe use, all endoscopists should be familiar with their design and construction.
- •
Endoscopists should be able to set up and connect an endoscope for a procedure correctly, and safely disconnect it afterwards.
- •
Understanding this allows endoscopists to troubleshoot common problems that may occur with e.g. lighting, suction, valves, etc.
Introduction
Although endoscope performance and reliability have vastly improved since they were first introduced in the 1960s, they are still tricky to handle and knowledge of their various components and features will enable their optimum and efficient use.
An endoscope is composed of the following elements: a control handle, the insertion tube and the connecting cable to the processor/generator.
1
The control handle
The control handle ( Fig. 1 ) combines the following elements:
- •
The main insertion tube, which terminates at the bending section
- •
The generator connecting cable, which has a connector at the end of it.

The control handle is intended for use with the left hand only, and combines all the necessary controls, which are ergonomically positioned. They consist of the following:
- •
Suction valve
- •
Air insufflation and lens cleaning valve
- •
Bending section and the control wheels
- •
Instrument elevator lever (depending on model)
- •
Proximal opening of the operating channel, which combines a watertight seal
- •
Buttons to freeze or capture an image or start video recording
- •
Buttons to activate the electronic zoom mechanism or switch imaging modes (e.g. FICE or NBI; see Ch. 6.2 ).
1.1
Suction and insufflation/cleaning valves
These contain joints that create a watertight seal and operate in cylinders. Suction and insufflation/cleaning occurs continuously so as to minimize delays on activation. The buttons also contain an air vent stack and, when released, return to their resting position by means of retraction springs.
1.1.1
Suction
The cylinder ( Fig. 2 ), which is attached to the aspirator at its base, communicates with the operating channel, to which it is joined by a circular channel.

The plunger houses a vent, which is uppermost when the plunger is in its resting position. The vent points upwards relative to the cylinder lumen, and air enters the cylinder via the plunger’s air vent stack.
When the plunger moves downward with its air vent stack occluded, the narrow portion of the vent appears opposite the lumen, followed by the wider portion of the vent, thus ensuring that there is sufficient suction force.
1.1.2
Insufflation–cleaning
The cylinder ( Fig. 2 ) integrates separate inlets and outlets for air and water. The inlet–outlet group that is closest to the base is the insufflation channel. The second group, which is higher up, is the lens cleaning channel.
A large water reservoir integrated into the middle section of the button separates the air and water.
The insufflation channel is open while in the resting position, but air escapes from the stack, which is larger than the insufflation tube and combines a one-way anti-insufflation valve.
When the plunger moves downwards, its base closes off the insufflation channel, and the water reservoir is moved into a position facing the cleaning channel. Inasmuch as the air pulsed by the insufflation pump cannot escape, it provides the pressure in the cleaning flask necessary to propel the water into the line.
This mechanism is either fully activated or deactivated, i.e. there is no intermediate position.

- •
To activate suction, block the orifice of the air vent stack with your left index finger and press down on the button. The amount of suction force is determined by how far the button travels into its cylinder.
- •
To activate air insufflation, block the orifice of the air vent stack with your left middle finger, but without pressing down on the button. As air can no longer exit the stack, it is forced into the insufflation channel valve.
- •
To clean the lens, press down on the air button as far as it will go.
1.2
Bending section controls
The two flexing controls ( Fig. 3 ) (large wheel for up/down, small wheel for left/right) are coaxial and each has a separate brake.

The most reliable system currently available comprises a cable wound onto a drum. The control cable is attached to the middle of the drum. Each free element makes a dead turn on the drum before being attached to the bending section cable.
When the drum is rotated using the control wheel, it rolls up the cable, which is attached to the tip of a series of articulated steel rings that are attached to each other (like vertebrae) and can bunch up in such way as to provoke the deformation shown in Figure 3 .
There are generally either four or six control buttons housed on top of the control handle.
1.3
Elevator control
The cable’s relatively short path enables a bar to be activated by a lever, which in turn mobilizes the elevator.
1.4
Operating channel
This channel has two functions: it allows instruments to be passed to the endoscope’s distal tip, and it is also used for suction. The channel is metal from its proximal opening to a point beyond the suction/insufflation buttons but from here distally is made of plastic. The two materials are joined at a cone (via a female element) that occludes the tube’s plastic walls.
2
Main insertion tube
The insertion sheath, which is mounted on the handle, combines the following elements:
- •
The fiberoptic image conductor
- •
One or more illumination bundles
- •
The operating channel
- •
The cleaning, and air/water insufflation channels
- •
The four bending section cables, which are in a separate sheath
- •
The elevator control cables.
The sheath, which terminates at the bending section, contains the lens at its distal tip and is used to explore the various parts of the gastrointestinal tract. It is fairly supple for the esophagus and stomach, but far more so in the duodenum. Hence, duodenoscope sheaths exhibit differential flexibility in their distal third segments. The segment of the sheath that is in the stomach is stiff enough to prevent it from forming loops as it passes along the greater curve.
Internally, the sheath ( Fig. 4 ) contains a spiral metal element covered with a metal plait that provides the external synthetic resin coating with support. The characteristics of the spiral determine the texture of the sheath, depending on the thickness of the metal element, and the extent to which the wound elements tend to form joints with each other. The type of metal used is also a key factor. Stainless steel is used for the upper segment, whereas bronze is used for longer colonoscopes. Alternatively, to obtain better rotational torque, two parallel coaxial spiral elements are integrated and move in opposite directions in such a way that they oppose each other.

3
Bending section
The bending section ( Fig. 3 ), which is the continuation of the main sheath, bears a strong resemblance to an alligator’s spine. The bending section is composed of a series of circular rings that are reciprocally articulated at a 90° angle. Each ring combines hinge plates that guide the bending section cables and keep them in place. The cables, which are soldered to the distal chain, pass over the hinge plates, and when they reach the main sheath, they enter the insertion tube. The rings must be non-contiguous, so that when a cable is pulled, causing the rings to bunch up toward the sheath, they cannot pivot on their axes and abut each other. When two cables are pulled concurrently, the flexing occurs at the bisector of the angle formed by the cables. Multidirectionality is obtained by the force of these crossed impulses.
Inasmuch as the rings are non-contiguous, they are covered with a plate that prevents them from pinching the external rubber coating, which is usually glued and stitched to either end of the bending section.
This method, although more rudimentary than vulcanization, is highly advantageous, in that it allows for rapid replacement of the cladding if it shows any signs of weakness.
4
The optical head
The optical head ( Fig. 5 ), which is an endoscope’s most distal component, provides either forward or side-viewing optics, depending on its intended destination and use.

The optical head is mounted on the distal end of the bending section, where all of the elements that are accommodated by the main sheath are attached in a watertight manner.
4.1
Forward-viewing optics
The light bundles are sealed in a watertight casing. The lens casing is rendered watertight by a parallel or prismatic porthole that accommodates the lens in its enclosure with or without a focusing element, and then the image conductor, which is wedged into the lens chamber. The air insufflation channel and the cleaning channel are bundled on a sprayer that moves toward the lens porthole to allow it to be washed.
The operating channel terminates at the lens, the head of which accommodates a protective rubber sleeve that prevents contact with the mucosa.
4.2
Side-viewing optics
The elements of this head are connected as described in the previous section.
The light source is bent at an angle and ends at the lens, which is mounted on the axis of the head and shines through a rectifier prism. The operating channel terminates next to the lens and is fitted with an elevator tab, the rest position of which is at a 45° angle relative to the conduit axis.
5
Generator connecting cable
This cable, which is attached to the control lever, accommodates the endoscope’s electrical and mechanical connections (tubes that bring fluids and also current from the generator to which the cable is connected via a port).
The sheath is structured in exactly the same way as the main sheath. The connector combines lateral elements that accommodate the suction tube and the water bottle tubing.
Conclusion
Videoendoscopes have supplanted fiberscopes. Fiberoptic image conductors have been replaced by miniature charge coupled devices (CCDs) that are increasingly sophisticated and that allow the operator to see far more detail than would be possible with the naked eye, while still employing the same types of operator controls and channels without compromising robustness or reliability ( Fig. 6 ).

Electronic videoendoscopy
- Jean Lapuelle
Summary
Introduction 5
- 1
Electronic videoendoscopes 5
- 2
Electronics and the endoscope 7
Conclusion 8
- •
Videoendoscopes have replaced fiberoptic instruments for almost all indications.
- •
Endoscopists should have a basic understanding of the principles of light transmission and how electronic video imaging works.
- •
Coupled charge device (CCD)s are improving all the time with greater numbers of pixels and miniaturization allowing better quality imaging by smaller diameter endoscopes.
- •
Optical and electronic magnification systems allow greater resolution of surface detail.
- •
Recent advances in technology allow endoscopists to see more mucosal detail and thus a greater understanding of mucosal anatomy and pathology will be required of endoscopists.
Introduction
The endoscope has changed greatly since the Bozzini endoscope was introduced in 1795 as the first instrument that allowed for rectal examinations. In the intervening two centuries, numerous advances have been made, notably the development of the rigid gastroscope, in 1868. The era of modern endoscopy was ushered in when the flexible endoscope came on the market in 1958.
In a fiberoptic endoscope, image resolution is determined by the number of optical fibers in the image bundle. Hence, image resolution is limited by the size of each fiber. This technology gradually improved to the point where, by the mid-1980s, each colonoscope contained 35 000 fibers.
Fiberoptic endoscopes have a number of serious drawbacks:
- •
Only one lens is available, which makes training in diagnosis and therapy difficult ( Fig. 1 ).
Figure 1
Operating principle of a fiberoptic endoscope.
- •
Endoscope fibers tend to break, such that:
- ▪
Increasing numbers of black dots appear, which greatly reduce image quality
- ▪
Bundle frame changes and color distortion occurs, particularly in terms of red light.
- ▪
In 1983, Welch Allyn introduced the first electronic endoscope on whose distal end a miniature camera was mounted, thus replacing the fiberoptic bundle.
The first electronic videoendoscope came on the market around 1986 ( Fig. 2 ).

1
Electronic videoendoscopes
The electronic videoendoscope works like a digital camera. Its distal end combines a coupled charge device (CCD; Fig. 3 ). This technology allows better image transmission and storage, leading to improved diagnosis and therapy. It has a coupled charge device and a color system.

1.1
Coupled charge device (CCD)
A CCD is composed of a silicon semiconductor with an insulating oxide coating to which aluminum electrodes known as MOS (metal oxide semiconductors) are attached. MOS are photosensitive, and convert light to electricity in accordance with the brightness of the light. The atoms of the MOS photosensitive surface store an electric charge when exposed to light. The accumulated electrical charge is proportional to the intensity of the incident light.
The photosensitive surface of a CCD is divided into a large number of photodiodes. This image element is often incorrectly referred to as a pixel. However, the photodiode that captures the light is actually part of the pixel, which is also the charge transmission channel. Thus a pixel actually refers to a device’s resolution, not the number of photosensitive cells (photodiodes) it combines.
If a digital camera is said to have a resolution amounting to 6.2 million pixels, this means that the CCD comprises 3.3 photodiodes. However, this artificially widens the scope of the definition, since additional pixels are produced by means of a software process known as interpolation. Interpolation involves inserting a new pixel into an image via a calculation between a number of existing pixels. It degrades the quality of the original image, which is why it is necessary to speak in terms of photodiodes rather than pixels.
Division of the target allows each photodiode to respond independently to the amount of light reflected by the tissue.
Photodiodes are discharged during the reading process before a new charge acquisition cycle begins. The reading process is extremely rapid, but during it, the electrical charges continue to accumulate on the image that is being transmitted. These additional (thermal) charges accumulate even in the absence of light. This signal is stronger for lines that are read last, i.e. the lines at the top of the image. This results in a problem known as image smearing, which can be resolved by transferring the useful portion of the frame to an area that is shielded from the light source.
This transfer process, which occurs extremely rapidly, occurs in various ways depending on the type of CCD used.
1.2
CCD frame transfer ( Fig. 4 )
CCD frame transfer provides optimal resolution and sensitivity. The image zone that gathers the electrons is transferred to a memory zone of the same size that is shielded from the light source. This separation increases sensitivity, thus providing maximum image-surface efficiency. The drawback of this solution is that it involves the use of a relatively large CCD.

1.3
CCD interline transfer ( Fig. 5 )
This configuration contains, alternatively, an image element area, as well as an electron transfer area that is shielded from the light source. These frames thus integrate a memory adjacent to each sensitive line and engender substantial dead areas in the final images. This is why relatively small CCDs exhibit limited sensitivity, despite their good resolution.

1.4
Full frame read-out CCD ( Fig. 6 )
This technology is identical to frame transfer CCD, but lacks a storage area that is shielded from the light source. Hence, these CCDs are small. Their drawback is that the lighting is not available throughout the reading process, as it is desirable to alternate between luminance at the charge creation locus and dark periods during which these charges are transferred.

A CCD is sensitive to black and white light, but is color blind, i.e. it responds identically to all colors. Hence, its charge is determined by luminous intensity. The advantage of CCDs is that they allow for a light spectrum that is potentially greater than the light that is perceptible to the naked eye.

Color is not a physical reality. It is an impression generated in our brain, via our eyes, by luminous radiation from objects. In 1676, Isaac Newton showed that sunlight can be broken down into the colors of the spectrum by passing light through a prism ( Fig. 7 ).

Light is composed of electromagnetic waves. Visible light comprises a continuous spectrum, the wavelengths of which range from 380 to 780 nanometers (nm). Each element of white light has a refraction coefficient that varies according to the element’s wavelength.
1.5
Color system selection
1.5.1
Sequential system: black and white CCD
This system successively analyzes the light reflected by an illuminated object based on the three primary colors: red, green, and blue. Inasmuch as all colors of the rainbow can be created using these primary colors, a color can be reconstituted via color superimposition using a black and white sensor.
Sequential lighting is obtained via a filter wheel that rotates at a rate of 30–50 times per second in front of the light source, while electric signals are emitted sequentially rather than concurrently. Hence, the information must be stored and projected concurrently 25 times per second via a video monitor. The video processor converts the analog information into a digital code, whereupon the inverse operation generates a television image. This system enables the processing and analysis of increasingly sophisticated CCD images, thus in turn giving an improvement in diagnostic capability.
1.5.2
CCD colors: a mosaic system
A CCD combines a mosaic filter that is transparent to one of the primary colors (red, green, or blue) or their complements (cyan, magenta, or yellow). Each photodiode captures a color and then reconstitutes a pixel using four photodiodes. Inasmuch as cyan is composed of blue and green, and yellow is composed of red and green, the color green has more potential luminance, i.e. red and blue have only 25% as much luminance as green ( Fig. 8 ).

2
Electronics and the endoscope
A video processor combines and processes information transmitted by a CCD in order to translate this information into a television image. This image is transmitted in two steps, or rather in two half-images using lines, i.e. odd-numbered lines followed by even-numbered lines. These are called interlaced images. In endoscopy, a CCD image must comprise a certain minimum number of vertical and horizontal pixels, which determine the image’s definition. Image resolution is determined by the number of pixels per image unit. Hence, image quality is determined by image definition and resolution. If the image does not contain enough pixels, it will not fill the screen. Latest generation CCDs allow for full-screen images.
2.1
Blooming
The capacity of each CCD pixel to store electrons is limited. If there is too much light, the electrons jump to the adjacent pixels, resulting in image distortion, as well as a phenomenon known as blooming (color dissociation at the edge of the object). Integration of an anti-blooming system changes the sensitivity via a loss of some of the photosensitive surface of a pixel.
2.1
Resolution
- •
Image resolution superior to that obtained with fibroscopes.
- •
The image can be viewed by a number of people at the same time in the examination room and remotely.
- •
Electronic videoendoscopes make the endoscopist more efficient by optimizing the intervention procedure and reducing the risk of complications associated with information transfer.
- •
Allows the operator to freeze images and archive the examination.
- •
Electronic videoendoscope hardware has a longer service life than that of videoscopes, with no image deterioration over time (black spots).
A light sensor comprises a fixed number of pixels. A lens’s resolution allows it to separate the image details. Resolution patterns make it possible to take real-time measurements in practice. The theoretical limit is reached when a pair of black and white lines is projected onto a pair of pixels.
The best videoendoscope is one whose CCD contains the most pixels, depending on the type of transfer system used. Some CCDs only use a portion of the image elements, while reserving the others for the transfer process.
Today, owing to improvements in image stability, color CCDs are gaining ground on their black and white counterparts and manufacturers are currently focusing on the following developments:
- •
CCD miniaturization, such that the 1/2″ pixel will be replaced by a 1/10″ pixel, which will allow extremely small devices such as transnasal videogastroscopes that are 5–6 mm in diameter, and twin-channel instruments that expand the range of therapeutic options
- •
High definition CCDs with very high numbers of pixels that allow for full screen images, i.e. very high definition CCDs (≥850 000 pixels).
Completely digital electronic videoendoscope systems (i.e. from the endoscope to the screen) open up (a) a major field of investigation by virtue of the wealth of information that is captured by the CCD; and (b) new image processing options.
Endoscopic imaging is making major advances thanks to the migration to digital technology.
Once the pixel count, lens, and field of exploration have been improved, the range of structures amenable to examination can be expanded by greatly improving the peripheral contrast of a lesion and enhancing its relief.
Addition of a zoom function may represent a major advance, but must nonetheless be validated scientifically. The following types of zooms are currently in use:
- •
An optical zoom function that allows for 100–150 times magnification of the image of the previously viewed lesion. When combined with chromoendoscopy or narrow band imaging (NBI, FICE, iSCAN), these zoom functions enable the detection and assessment of subtle, superficial lesions in the upper GI tract and colon (see Ch. 6.2 ).
- •
An electronic zoom allows artificial magnification of pixels.
Conclusion
Electronic videoendoscopes still await further technological improvements in terms of CCDs and pixel counts.
New high resolution CCDs are 45% smaller than classic CCDs and integrate octagonal photodiodes that theoretically allow storage of more information in the same surface area. With this type of CCD, 1.7 times more photodiodes can be obtained relative to the previous generation of CCDs ( Figs 9, 10 ).


These super-CCDs and their zoom functions facilitate detection and diagnosis of early neoplasia of pit patterns and by characterization of capillary vascular patterns. Other technologies are in the pipeline that are based on certain characteristics of silicon and that will allow a single photodiode to capture three colors. This will obviate the need for colored filters, greatly reduce the scope of color reconstitution calculations and algorithms, increase image processing, and heighten sensitivity.
Thus, in the foreseeable future, we may have videoendoscopes that contain more than 5 million photodiodes.
Further Reading
Endoscopic accessories
- Jean-Christophe Létard
- Jean Marc Canard
Summary
Introduction 9
- 1
Tissue grasping and acquisition 9
- 2
Injection 9
- 3
Clips and ligation devices 10
- 4
Dilatation 10
- 5
Coagulation 10
- 6
Tissue resection 11
- 7
Gastrointestinal stents 11
Conclusion 11
- •
A vast array of endoscopic accessories is available and new ones are being developed as novel interventional endoscopic procedures are described.
- •
Endoscopy units should stock an adequate range and supply of commonly used accessories at all times.
- •
Endoscopists should be familiar with the appropriate and safe use of all endoscopic accessories.
- •
All staff should be trained in the safe use of endoscopic accessories.
- •
In many countries, legislation mandates single-use, disposable accessories to comply with infection control policies.
Introduction
Millions of endoscopic procedures are performed each year around the world and interventions ranging from biopsy to complex therapeutic procedures are increasingly undertaken. At upper endoscopy, biopsies are the most common intervention, while therapeutic procedures, e.g. PEG insertion or variceal therapy, occur in 7–10% of cases. Other procedures such as dilatation, esophageal stenting, argon plasma coagulation (APC), endoscopic mucosal resection (EMR) and polypectomy are increasingly undertaken. Polypectomy is the main therapeutic procedure performed at colonoscopy (10–25%), other interventions being performed in 2.5% of cases (argon plasma coagulation (APC), EMR, dilatation, and stenting).
Medical supplies needed for endoscopic procedures relate to both patients and caregivers: disposable paper hospital gowns, absorbent pads, paper gowns for staff, non-sterile gloves and gauze swabs or compresses. Oxygen masks and tubing, lubricant gel and Xylocaine spray are also necessary. Small transparent caps to facilitate magnifying endoscopy and disposable brushes are also indispensable. Endoscopic accessories must be available that allow for grasping and biopsy, injection, hemostasis, mechanical suture, dilatation, sectioning, and prosthetic re-establishment of gastrointestinal lumens.
1
Tissue grasping and acquisition
Gastrointestinal biopsies pose a major challenge for the endoscopist. They are undertaken using 5 mm-long forceps with spoon-shaped jaws ( Fig. 1 ), which employ one of the following mechanical principles:
- •
Pantograph , whose jaws close via a cable controlled mechanism
- •
Passive sheath , where the jaws scissor and enter via traction provided by a sheathed cable
- •
Ball and socket , which combines the two aforementioned principles, whereby the articulating ball greatly reduces the amount of effort needed to operate the forceps
- •
Forceps with a multiple aspiration chamber and guillotine sectioning, which allow a number of specimens to be taken simultaneously.

Forceps are available in different lengths and external diameters to be compatible with gastroscopes, (including pediatric instruments), colonoscopes, and enteroscopes. Biopsy specimen size appears to correlate with the forceps jaw size. A central metal spike is not mandatory and can in fact damage the endoscope operating channel.
The removal of foreign bodies requires longer, and in some cases rubberized, crocodile or rat-tooth forceps. Dormia baskets, polyp traps and ‘Roth’ nets can be used for batteries and components. It is useful to have an overtube for the extraction of foreign bodies >6 cm in length, to prevent them being dropped and inhaled on removal, and protective sheaths for the removal of sharp objects.
2
Injection
Injections are performed: (a) with the aid of a basic catheter for fluoroscopic opacification (prior to insertion of a gastrointestinal stent); (b) with the aid of a spray catheter for chromoendoscopy, or (c) via disposable needles for tattooing, hemostasis, variceal sclerotherapy, chromoendoscopy (see Ch. 2.4 ), EMR, submucosal dissection, and antireflux treatment ( Fig. 2 ).

Injection hemostasis is performed using adrenaline diluted to 1/10 000 for gastroduodenal ulcers, Mallory–Weiss tears, acute Dieulafoy lesions polyps and bleeding diverticula. Polidocanol (1%), or 5% ethanolamine is used for esophageal variceal sclerotherapy. Injection catheters are being improved constantly, with needles ranging from 18 to 23 gauge that slide very easily in 7–8 French catheters, some of which contain a cleaning system. Biological tissue adhesives can be used with dual-chamber needles. Precision relies on the length and diameter of the chosen needle (narrow for injection of fluid, larger diameter for injection of carbon for tattooing; short bevelled needle for the colon, longer for the upper GI tract).
3
Clips and ligation devices
Hemostasis can be performed using clips and ligation systems. Reusable and disposable clipping systems are available and their use is described in Chapter 7.8 . Clips can also be used for marking the site of a lesion or for closing small perforations that occur during polypectomy, EMR or ESD. Disposable ligation systems are available with 6–10 elastic bands for esophageal variceal ligation and to assist EMR ( Ch. 7.8 ). They are also used for hemostasis of bleeding Dieulafoy lesions, and for Mallory–Weiss tears ( Fig. 3 ).

Disposable miniloops that work like clips can also be used, particularly in the gastric cardia. Detachable snares (‘endoloops’) are very useful for preventing post-polypectomy bleeding. However, they are contraindicated for polyps with thin or short stalks, as premature transection of the stalk and bleeding can occur.
4
Dilatation
Dilatation (see Ch. 7.1 ) is performed using progressively larger bougie dilators over a rigid or semi-rigid metal guidewire or else using disposable balloons ( Fig. 4A ). Balloon diameters and length vary. The balloons are passed through the operating channel and dilatation is performed hydrostatically (except in cases of achalasia) under visual and/or fluoroscopic control.

5
Coagulation
Coagulation (see Ch. 1.4 ) can be monopolar, bipolar ( Fig. 4B ), or multipolar, and can be performed using dedicated probes. Coagulation is useful for tumor debulking and for hemostasis. In monopolar coagulation, a high-frequency electric current is applied to the tissue, requiring a patient grounding pad (25–40 watt (W) pulses for 7–10 s). This method is risky as the muscle layer may be coagulated and delayed perforation may occur. Argon plasma coagulation (APC) is less risky, and is also more appealing by virtue of its cost-effectiveness and multifunctionality (60 W, 0.8–1.5 l/mn). The advantage of bipolar coagulation, which uses three electrodes, is that the electric current is conducted back to the electrosurgical generator (useful in the presence of a pacemaker).
Bipolar probes contain a lateral spiral filament at their distal end (10–20 W, 3–4 pulses lasting 10–14 s each). The contact must be tangential as the distal tip of the probe is perforated and has no conductor, thus allowing for cleaning. Some probes are equipped with a distal injection needle. Diathermic heater probes ( Fig. 5A ), which are used in some countries, comprise an internal thermocouple that generates a constant temperature of 250°C at the distal end (which has an anti-adhesive coating). This system also houses three 1-cm cleaning channels above the active distal portion (8-s 20–30 joule pulses).

6
Tissue resection
Sectioning (see Ch. 7.11 ) occurs using 200–500 volts HF current that generates an electric arc between the diathermy snare and the tissue. The latest generation of electrosurgical generators allows automatic stabilization of fluctuations in potential and intensity. The heat generated at the points where the electric arc comes into contact with the tissue is so high that the tissue is immediately vaporized. Following this, as the snare moves across the tissue, electric arcs are generated continuously wherever the gap between the tissue and snare is small enough, thus producing the resection.
It is useful to have a range of snares ( Figs. 5B,C,D ): monofilament; braided; small size (10 mm); large size (20–30 mm); asymmetric for esophageal EMR; and barbed for large colonic EMRs. Transparent caps with an edge groove (into which the loop inserts) are also essential.
7
Gastrointestinal stents
A range of self-expanding metal stents (SEMS) (see Ch. 7.2 ) are available for use in the esophagus, stomach, duodenum, biliary tree, and colon. Most of today’s gastrointestinal prostheses are made of hardened steel (articulated components that are 2.5 cm in diameter and that do not become shorter on expansion), nitinol or Elgiloy (mesh or webbing composed of one or more wires, the length of which decreases by 30% as the prosthesis expands). The stents are straight, and may or may not have funnel-shaped or ‘dog-bone’ tips. Anchorage, extraction and antireflux valve systems are also available for these devices and some may be completely or partially membrane-covered to minimize tumor ingrowth.
Conclusion
Modern endoscopists must be familiar not only with an increasing range of endoscopes and techniques, but also a growing array of endoscopic accessories with which to undertake interventions. Sophisticated accessories and knowledge of how to use them properly underpin the success of complex therapeutic endoscopy.
Further Reading

The American Society for Gastrointestinal Endoscopy (ASGE) website ( www.asge.org ) contains technology evaluation reports and guidance covering the majority of accessories used in endoscopic practice and is a valuable resource.
Electrosurgical generators: procedures and precautions
- Jean Marc Canard
- Ian Penman
Summary
Introduction 12
- 1
Electrophysical basis of electrosurgery 12
- 2
Problems associated with older electrosurgical generators 13
- 3
Principles of endoscopic diathermy (electrosurgery) 14
- 4
Coagulation 15
- 5
Monopolar and bipolar current 15
- 6
Alarm systems 16
- 7
Statutory requirements of endoscopy rooms 16
- 8
Precautions for cleaning and disinfection of equipment 16
- 9
Practical tips for endoscopic electrosurgery 16
Conclusion 17
- •
Electrosurgical diathermy is an important component of daily endoscopy practice.
- •
A thorough understanding of the correct use of different types of electrosurgical current is essential.
- •
Newer generators provide automatic regulation of output power; they integrate an endoscopic diathermy (‘endo-cut’) mode (fractionated sectioning alternates automatically with low-intensity coagulation).
- •
Bipolar current does not produce effective tissue sectioning.
- •
Complications with electrosurgery result from the effects of the electric current. Perforations are almost always attributable to excessive coagulation of the gut wall and bleeding almost always results from unduly rapid cutting.
- •
Argon plasma electrocoagulation paved the way for the development of interventional endoscopy and has replaced laser methods for most of the latter’s indications.
Introduction
To achieve satisfactory results when using electric current for polypectomy, EMR or sphincterotomy, tissue sectioning and hemostasis must be effective, and the following must be avoided:
- •
Immediate bleeding caused by unduly rapid sectioning or not enough coagulation
- •
Delayed perforation resulting from unduly slow sectioning or excessive coagulation.
Moreover, sectioning must begin carefully to avoid causing changes in tissues that are being coagulated. Advances in electrosurgical generator techniques help achieve these goals and this chapter describes their safe use.
1
Electrophysical basis of electrosurgery
In-vivo application of electric current to biological tissues creates an electrolytic effect , neuromuscular excitation , and a thermal effect .
In endoscopy, only the thermal effect is used. It is obtained via high frequency AC current exceeding 300 kHz which, unlike low frequency current (e.g. household appliances), does not cause neuromuscular excitation or cardiac rhythm disturbances.
The heat provided and the tissue effects engendered by this are determined by current intensity , specific tissue impedance and current-application time .
- •
Tissue resection is obtained using intense heat. When intense heat is applied to tissues, water rushes out of the cells, which explode, thus triggering the resection. It can only occur in the presence of an electric arc and >200 V of electricity between the tissue and electrode ( Fig. 1 ).
Figure 1
During sectioning, which is performed at temperatures exceeding 100°C, water comes rushing out of the cells and the cells explode.
- •
Low-intensity coagulation/desiccation without an electric arc is performed using lesser intensities and engenders less heat. The water flows out of the cells and extracellular space slowly, and the tissues contract. Less than 190 V should be used to ensure that no electric arc forms ( Fig. 2 ).
Figure 2
In coagulation, which is realized at temperatures of <100°C, the water emerges slowly from the cells, which retract.
- •
Forced coagulation involves desiccation but uses higher voltage as for cutting, as well as an electric arc, which carbonizes the tissue.
- •
Spray or fulguration coagulation involves surface desiccation of the tissue, which is induced by ultra-high voltage (>4000 V) that produces a very large electric arc. However, the electrode does not come into contact with the tissue.
- •
Argon plasma coagulation (APC) uses very high voltage via a spray. The effect of the electric arc is mitigated by ionized gas, which distributes the energy evenly across the tissue without carbonizing it.
Sectioning, as well as the aforementioned monopolar coagulation methods, necessitates a second pole in the form of a neutral electrode, to recover the energy generated by the activated electrode or probe.
2
Problems associated with older electrosurgical generators
The power created by older electrosurgical generators is constant and does not vary with tissue and cutting surface impedance. Cutting speed and electric arc intensity are the only variable parameters with these devices. In today’s generators, electric-arc intensity is constant and controlled. Cutting speed is preadjusted in endoscopic diathermy mode without the need for any action on the part of the operator. The electrosurgical generator’s output power is regulated automatically in accordance with the contact surface. The most common unit is the ERBE ICC 200 ( Fig. 3 ), recently replaced by the VIO 200 or 300 series.

2.1
The role of automatic regulation of the electric current
When, for example, 100 W of current pass through the tungsten filament of an electric light bulb, there is a substantial thermal effect that turns the filament white, thus generating light, which is in fact a secondary effect. In the copper wire that provides the electricity for the light bulb, this same electric current engenders little or no heat. Hence, various types of biological tissue exhibit differing levels of current impedance (resistance). In order to obtain a stable effect in tissue, the level of electric current administered to the tissue must be commensurate with the tissue’s impedance, which, if elevated, requires relatively little voltage. Conversely, low-impedance tissue needs considerable voltage to achieve the same effect.
2.2
Sectioning may inadvertently result in tissue coagulation
This can occur if too little current is applied to the target contact surface ( Fig. 4 ). Sectioning a 1 mm 2 contact surface requires a high level of current density. This same current applied to a 1 cm 2 surface will be unduly low and will induce coagulation. New electrosurgical generators avoid this problem by automatically adjusting the instrument’s output current to the characteristics of the tissue being sectioned, within the limits of the maximum-current setting, which must be high enough to allow sectioning, as otherwise the tissue will be coagulated.

2.3
Consequences of using unsuitable settings for electrosurgical generators
The device settings must be defined in such a way as to (a) prevent the generator from peaking out; and (b) ensure that the device’s power is sufficient to perform the section and does not induce coagulation and accidents.

Complications result from the effects of the electric current. Delayed perforations are almost always attributable to excessive gastrointestinal wall coagulation, and bleeding usually results from unduly rapid cutting.
3
Principles of endoscopic diathermy (electrosurgery)
In endoscopic diathermy, all of the electrical settings that are applied to the section and its characteristics are automatically controlled and adjusted so as to achieve optimal cutting performance throughout the process ( Fig. 5 ). The electrical arc’s voltage and intensity between the tissue and cutting wire are measured, analyzed and stabilized by an onboard microprocessor. Endoscopic diathermy is a fractionated process that is carried out via the following stages:
- •
The initial incision (variable duration) via the ‘Power Peak System’ (ERBE) or equivalent facility in other generators
- •
Sectioning when the electric arc first appears (roughly 50 ms)
- •
Low intensity (0 to 60 W) coagulation (roughly 750 ms).

All of these parameters are regulated automatically via the device’s power.
3.1
The Power Peak System and Endocut
At the beginning of the process, the Power Peak System (PPS) delivers power exceeding the configured threshold for a few milliseconds (ms) so as to allow rapid initiation of resection.
Following this, in endocut mode, sectioning occurs for 50 ms, followed by low-intensity coagulation for 750 ms. Sectioning and coagulation alternate automatically until the tissue has been completely resected. When using ‘endo-cut’ mode, the operator should avoid pulling too hard on the snare, or closing it too tightly; it should be in gentle contact with the tissue at all times. This results in a slow cutting process that alternates with coagulation. The risk of hemorrhaging is greatly reduced if the operator does not section mechanically by pulling hard on the snare. By the same token, the risk of secondary perforation and excessive coagulation is reduced if the resection begins immediately on application of current.

During polypectomy, do not pull hard on the snare or close it too tight as this will result in mechanical transection of the stalk and bleeding – let the endo-cut function of the generator section the stalk in a controlled manner.
3.2
What to do if the resection does not start immediately
There are two possible scenarios in this case:
- •
If you pull too hard on the snare or close too tightly, it will be ‘buried’ in the tissue and prevent the electric arc from firing.
- •
The contact surface between the snare and tissue may be unduly large. The larger this surface, the weaker the impedance and the more power needed to start the incision. If the incision fails to start, coagulation will occur instead of cutting (because current is nonetheless administered to the tissue). If this occurs, replace the snare and start again with more power (160–200 W).
The high power generated by an automatically regulated electrosurgical generator poses no risk for the operator for the simple reason that the device only generates the amount of power that is needed for sectioning.
3.3
Endocut for sphincterotomies
Endocut is used frequently for sphincterotomies with lower settings in some cases and identical settings in others, and using less coagulation than for polypectomies (endo-cut I mode in ERBE VIO). The use of an electrosurgical generator for sphincterotomies gives the operator better control over the sectioning process, without provoking bleeding, and this in turn avoids abrupt sectioning, perforations and secondary hemorrhaging. A randomized study comparing an Erbotom T175 (classic electrosurgical generator) with an ERBE ICC 200 (which has an endoscopic diathermy or ‘endo-cut’ function) revealed a significant difference in terms of reducing secondary bleeding.
3.4
Starting endocut
To start (‘endo-cut’) mode, press the yellow foot pedal, whereupon the cutting/coagulation cycle will take place automatically. All you need to define is the sectioning power threshold, in accordance with the diameter of the polyp that is being resected (if in doubt, use the 120 W default setting with effect level 3 or 2 hemostasis) and set the coagulation (blue pedal) to ‘low’ (60 W) to avoid an undesired result in the event of accidentally pressing the wrong pedal.
The low-coagulation power in endo-cut mode is set by default to from 0 to 60 W, which automatically overrides any manual setting on the electrosurgical generator.
4
Coagulation
4.1
Low-intensity coagulation
The low-intensity coagulation provided by using the blue pedal occurs without an electric arc or tissue coagulation. This type of coagulation is slow, penetrating, and provokes no bleeding.
4.2
Forced coagulation
Forced coagulation is obtained using 1300 V resulting in an electric arc and tissue carbonization. This type of coagulation is quicker and less penetrating than low-intensity coagulation.
4.3
Spray-mode coagulation
Spray-mode coagulation is only used in gastrointestinal endoscopy in conjunction with an argon plasma coagulator and requires >4000 V of electricity. In this process, ionized argon gas is applied via flexible probes that do not come into contact with the tissue. The gas acts as an electrical conductor between the probe and tissue. Argon plasma distributes heat evenly across a very large surface. The coagulation is very rapid and does not penetrate nearly as deeply as with laser coagulation. However, the coagulation depth is determined by the preset power and particularly by the target-surface application time (there is no automatic regulation in Argon spray mode). For endoscopic procedures, APC is superior to laser coagulation for all indications other than destruction of bulky tumors, where laser still has a role.
5
Monopolar and bipolar current
5.1
Monopolar current ( Fig. 6 )
Monopolar current circulates between the active electrode (diathermy snare, hot biopsy forceps, sphincterotome), and the patient grounding pad.

5.2
Bipolar current ( Fig. 7 )
Here, the second electrical pole is located in very close proximity to the active element, and the current circulates between the two ends. If a bipolar snare was used with an electrosurgical generator for a bipolar section, the electric arc (and hence the resection) would occur on one side of the snare only, where there is less contact with the tissue. The other side would assume the function of a neutral electrode and would coagulate rather than section. No bipolar instrument is available for endoscopic sphincterotomy and for polypectomy. Bipolar current is used solely for coagulation with specific bipolar probes . The coagulation depth obtained with bipolar current is less than with monopolar current owing to the fact that the current is recovered immediately.

Bipolar devices should not be used for gastrointestinal procedures as they do not provide suitable tissue resection. Bipolar current should only be used for coagulation procedures in patients with cardiac pacemakers.

6
Alarm systems
These alarm systems allow for detection of:
- •
A defective or improperly connected patient grounding pad
- •
Incorrect voltage
- •
Improper pedal activation.
They ensure that endoscopic electrosurgery is safe for the patient and operator. The grounding pad monitoring system (NESSY) guarantees that no skin burn will occur under the neutral pad, providing that double-face pads are used ( Fig. 8 ).

7
Statutory requirements of endoscopy rooms
The endoscopy room must meet the following statutory requirements:
- •
It must have an antistatic floor
- •
It must have safe electrical sockets
- •
It must be devoid of any power strips, extension cords, loose wires or cables
- •
The electrical mains must be isolated by circuit breakers with a nominal current of 10 A.
- •
The electrosurgical generator must be set up in a location that is well ventilated to allow proper cooling.
8
Precautions for cleaning and disinfection of equipment
Clean the generator with products that are non-inflammable and non-explosive in a dry atmosphere. Do not clean the front panel of the device using alcohol or any hard or sharp equipment and do not allow any dampness to enter the generator. Argon plasma coagulation probes are single use and disposable in most countries, as are polypectomy snares and sphincterotomes.
9
Practical tips for endoscopic electrosurgery
9.1
General tips
- •
Only use double-face patient grounding pads
- •
Press the yellow pedal to cut and the blue pedal to coagulate
- •
When performing a polypectomy, bear in mind that the larger the contact surface between the snare and polyp, the lower the impedance in the tissue, which means that more power is needed to start sectioning
- •
An unduly tight snare will make it more difficult to start cutting
- •
If cutting does not begin immediately, withdraw the device at once, because current is still being administered and will coagulate the tissue instead of sectioning it. In such a case, replace the snare and start again
- •
If the polyp has a stalk, resect the polyp at the stalk’s narrowest point.
9.2
Standard settings for an ERBE ICC 200 electrosurgical generator
The endoscopic diathermy (‘endo-cut’) function can only be obtained using the yellow pedal, in which case cutting and coagulation will alternate automatically ( Fig. 9 ).

9.3
Polypectomy
- •
For polyps with a contact surface measuring <1 cm: endo-cut: hemostasis 3; 120 W; low-intensity coagulation: 60 W.
- •
For polyps with a contact surface measuring >1 cm: endo-cut: hemostasis 3; 160–200 W; low-intensity coagulation: 60 W.
9.4
Sphincterotomy
To avoid the problems caused by excessive contact surface between the sphincterotome and tissue, insert only the distal third of the cutting wire into the papilla. The endoscopic diathermy (endo-cut) function is adjusted for hemostasis and using the same amount of power as for a polypectomy (120 W). Low-intensity coagulation is performed using 60 W.
9.5
Endoscopic mucosal resection (EMR)
- •
For EMR without a submucosal injection: deactivate the endo-cut function by pressing the endo-cut button on the front of the generator and use hemostasis level 2 and 120 W. For continuous sectioning, press the yellow pedal.
- •
For EMR with a submucosal injection: endo-cut mode can be used with hemostasis set at 2 and 120 W.
There are small but significant differences with the newest range of ERBE electrosurgical generators (VIO 200 or 300) ( Fig. 10 ). Two different endo-cut functions (Q and I) exist: ‘endo-cut Q’ is designed for polypectomy and functions much as described above with slow cutting and more coagulation. The standard setting for a polypectomy is 120 W, effect level 3 (the effect level can be reduced to 2 if less coagulation is desired). There are, however, many adjustable settings and general recommendations are outlined in Tables 1 and 2 . ‘Endo-cut I’ is designed for sphincterotomy with a different cutting-coagulation cycle, designed for quick cutting and less coagulation, although it can be adjusted.

9.6
Argon plasma coagulation (APC)
Equipment settings and the use of APC is discussed in detail in Chapter 7.4 .
Conclusion
Previous generation HF generators have the following drawbacks relative to the latest-generation devices: less regular cutting speed; sectioning is more difficult to initiate; less regular electrical arc intensity; greater risk of secondary perforation and/or hemorrhaging. New devices are superior in terms of: allowing automatic regulation of output power (regulated electrical arc); they integrate an endoscopic diathermy (‘endo-cut’) mode (fractionated sectioning alternates automatically with low-intensity coagulation) and a Power Peak System. The latter system provides a burst of power (above the configured level) for a fraction of a second, thus allowing for a rapid start and avoiding gastrointestinal wall coagulation. These developments have improved the effectiveness and safety of many interventional therapeutic procedures.
Further Reading
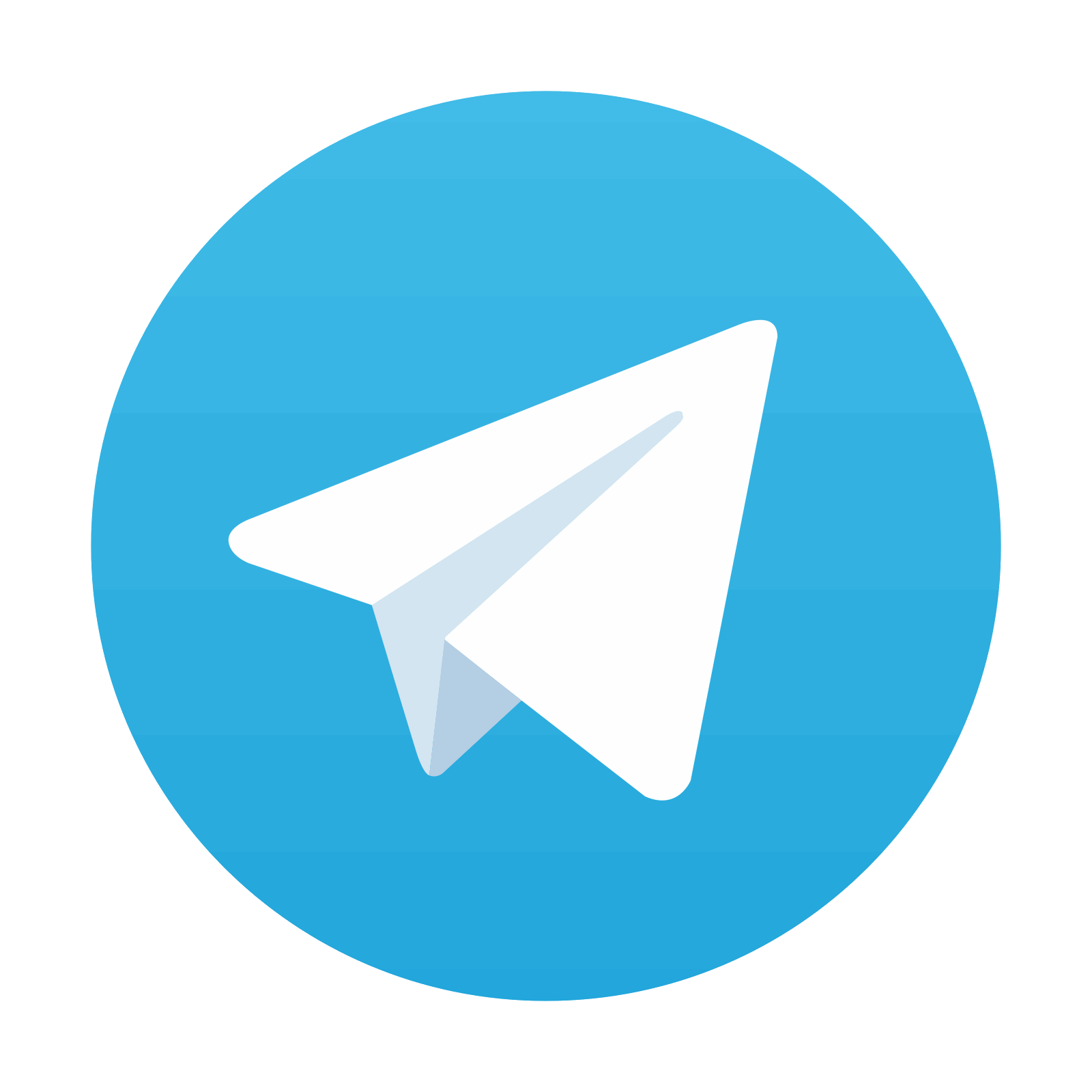
Stay updated, free articles. Join our Telegram channel

Full access? Get Clinical Tree
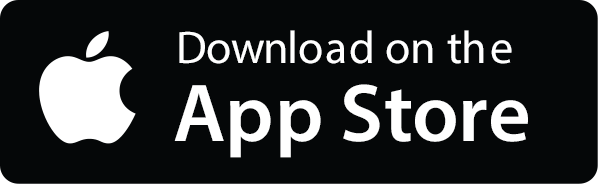
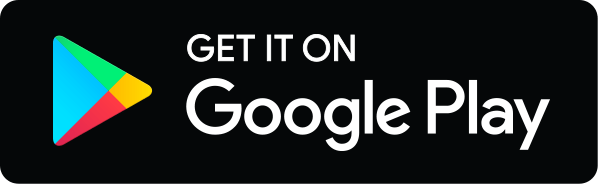