© Springer International Publishing Switzerland 2017
Vincent Obias (ed.)Robotic Colon and Rectal Surgery10.1007/978-3-319-43256-4_2020. Intraoperative Conversions in Robotic Colorectal Surgery
(1)
Department of Surgery, George Washington University Hospital, 22nd & I Street, NW, 9th Floor, Washington, DC, 20037, USA
Keywords
RoboticsColorectalLaparotomyConversionIntroduction
Robotics is quickly becoming the new gold standard for minimally invasive colorectal surgery, with recent studies showing shorter postoperative courses , decreasing operative times, and improved conversion rates when compared to laparoscopy [1–5]. Operative times currently appear to be longer with robotic colectomy, in part because most published studies are retrospective and typically clump actual operative time with docking and repositioning the robot. While prospective databases like ROLARR (NCT01736072) mature, it is important to note that early meta-analysis and single surgeon analysis have shown that operative times approach that of laparoscopy as surgeon and staff experience grow [5, 6]. The benefits of robotic colorectal surgery are especially apparent with rectal cancer and low pelvic dissections, as operative times and rates of conversion to laparotomy are superior compared to laparoscopy [7, 8].
Minimally invasive colorectal surgical operative times and conversion rates are important to consider, as studies have shown benefits versus laparotomy but diminishing returns in patient outcomes as operative time surpasses 180 min [9, 10]. In MIS and laparoscopy in particular, longer operative times are usually a result of five factors: excessive tumor fixity, anatomic uncertainty, tumor clearance, patient obesity, and surgeon experience [11, 12]. Recently published data from Canada citing approximately 500 robotic and 8400 laparoscopic colectomies demonstrated no difference in mean operative time (190 versus 187 min, respectively) and a significantly lower incidence of conversion to laparotomy in the robotic group (9.5 % versus 13.7 %, respectively) [13].
With more than 30 % of the US population over the age of 20 having body mass index (BMI) values above 30 [14], the effects of BMI values on surgical outcomes have taken center stage. The visceral adiposity and larger abdominal wall can confound fine movements and make intraperitoneal manipulation of organs cumbersome. Multiple advanced laparoscopy studies have demonstrated direct links to complications related to obesity, including superficial and deep infections, longer operative times, and incisional hernia formation [15–17], as well as conversion from MIS to laparotomy [9, 18, 19]. Regarding infectious and hernia-related complications, the short-term outcomes of robotic colorectal surgery are similar to laparoscopy as BMI values increase [9, 18]. Other datasets suggest that while BMI values greater than 30 increase average operative times by up to 30 min, conversion rate, blood loss, leak rate, and overall complication rate are unchanged [13, 20, 21], supporting the claim that robotics are unaffected by BMI values. Resilience of robotic surgery outcomes to the effects of elevated BMI values is likely due in part to the fact that the robot mitigates the fulcrum effect of the trocar and the abdominal wall, stabilizing the instrument and camera and allowing for fine motion with minimal effort.
Generalizations can be made supposing that advanced tumors, prior radiation, and pathology resulting from inflammatory conditions like diverticulitis and inflammatory bowel disease lend themselves to increased anatomic fixity [22, 23] and increase the risk of unplanned conversion [13]. In fact, colon cancer (OR 1.810), Crohn’s (OR 2.194), and diverticular (OR 1.980) disease were shown to be independent risk factors for unplanned conversion. Robotic surgery, however, was found to be protective against conversion versus laparoscopy (OR 0.713) for the aforementioned pathologic processes [13]. Interestingly, ulcerative colitis was not found to be an independent risk factor for unplanned conversion. Other studies have also shown viability of robotic completion proctectomy and ileal pouch anal anastomosis for ulcerative colitis [24, 25] with improved conversion rates but longer operative times when compared to laparoscopy [26].
As in the early days of laparoscopy, there is a learning curve associated with robotics. Early studies investigated single surgeon progression using cumulative sum analysis for the experienced colorectal laparoscopist and discovered three distinct phases: a learning phase when docking the robot is mastered, a plateau phase when the surgeon consolidates his/her skills and confidence, and an mastery phase when the surgeon expands case variety [27, 28]. Multiple smaller studies report that approximately 25 cases were required to reach the “mastery” phase. However, a significantly larger Korean single surgeon study concurred with the general findings of three stages of learning robotics, but cited initial phases of 35 cases, plateau phases of 93 cases, and mastery phases of 69 cases. It is important to note that the demographics of the later phases of the Korean study included lower rectal lesions and neoadjuvant chemoradiation, while the first phase was comprised of a statistically higher number of tumors more than 8 cm from the anal verge without chemoradiation [29]. Most importantly, none of the studies showed a significant increase in intraoperative conversion or complication rate as the surgeon progressed through phases one and two, suggesting that learning robotic colorectal surgery does not place the patient at undue risk.
Specific Topics
Pneumoperitoneum
Carbon dioxide (CO2) is the medium currently used to establish insufflation for MIS due to its stable combustion profile and its ability to be physiologically absorbed in tissues and subsequently expelled in the lungs of healthy patients. Along these lines, most contraindications to pneumoperitoneum revolve around inability to tolerate increased abdominal pressures and an inability to absorb and excrete CO2 in the lungs. As with laparoscopy, complications that arise from establishing pneumoperitoneum are usually resolved by evacuation. In these cases, it may be wise to discuss the patient’s condition with the anesthesiologist and consider delaying the procedure or proceeding with laparotomy in cases of emergency.
Capnothorax is a phenomenon caused by insufflation of the thorax with CO2. In colorectal surgery this is usually from micro/macroscopic defects in the diaphragm and can ultimately lead to tension pneumothorax physiology [30]. Pathologically, this manifests as hypercarbia and decreased cardiac output. The body attempts to correct by increasing expulsion of CO2 by increasing cardiac output and thus pulmonary blood flow but is unable to given decreases in preload from the increased intrathoracic pressure [31]. Visually this can be verified by caudal displacement of one or both hemidiaphragms with absent or decreased breath sounds in the affected hemithorax.
Management is based on the clinical significance, which is stratified by a drop in systolic pressure between 15 and 35 mmHg, increased airway pressures, PaCO2 greater than 50 mmHg, or SpO2 less than 95 % [30, 32–34]. When these conditions are met, immediate evacuation of pneumoperitoneum with concomitant surgical evacuation of the affected hemithorax should be performed. Following hemodynamic stabilization, the operator may attempt reinsufflation but should entertain conversion to laparotomy. Conversely, capnothorax without hemodynamic compromise can be managed with observation and usually resolves shortly into the postoperative period [34, 35].
CO2-related embolic events occur rarely (15 per 100,000 per year in laparoscopy) and are usually heralded by sudden hemodynamic collapse with decreased end-tidal CO2 and decreased chest compliance. Physiologically this occurs from CO2 accumulating in the right ventricle or in the pulmonary arterial system, causing a “gas lock,” and preventing forward flow of blood [36]. The remedy is placing the patient in the Trendelenburg position, with subsequent placement of a central venous catheter and aspiration of the air from the right side of the heart. Prophylactically, this can be prevented by ensuring adequate resuscitation in the preoperative phase. This condition is traditionally seen with liver resection, given the caliber of the venous sinuses; however, occurrences following manipulation of sacral plexus veins have also been reported [36, 37].
Robotic Malfunction
As with any complex machine, mechanical and software failures do occur. Between 2000 and 2013 of 1,745,000 robotic procedures performed in the United States across multiple surgical specialties, an estimated 10,624 adverse events (0.6 %) were related to malfunction reported using the MAUDE database [38]. Of these 10,624 incidents, 1535 had clinically significant patient outcomes including injury (1391) and death (144). By comparison, there is little reported regarding the failure rate of laparoscopic instruments. The malfunctions included video/imaging (7.4 %), broken/burnt pieces falling into the patient (14.7 %), electrical discharge (10.5 %), unintended instrument action (10.1 %), and other issues with “electrosurgical units, power supplies/cords, patient side manipulators, etc.” Fortunately, approximately 10 % of these malfunctions were identified prior to beginning surgery and the case could be delayed/rescheduled. Other large multi-institutional urologic reports cite a critical failure rate of 0.4 %, leading to case cancellation in 24 cases and conversion in 10 cases out of 8240 [39]. Single institutional studies cite a total malfunction rate of 2–4 % with a robotic system failure rate of less than 2 % (excluding instrument failure) and a need for resulting conversion of less than 1 % [40–42].
Focusing specifically on mortalities associated with robotic surgery in this particular report, 17 % of the 144 reported deaths occurred during the procedure itself while 75 % in the postoperative period. Intraoperatively, less than ten deaths were related to inadvertent damage to organs, while the rest were secondary to uncontrolled bleeding and cardiopulmonary pathology. Overall, 50 % were reported as risk inherent to the procedure, 11 % as underlying medical disease, and 7 % as surgeon/staff mistakes [38].
Based on the nature of the malfunction, the da Vinci ® System classifies the faults as recoverable (yellow light on instrument) or nonrecoverable (red light on instrument). When faults occur, all robotic arms are locked and can be moved by clutching, but require additional effort to do so; for nonrecoverable faults, the offending instrument must be removed from the body and the robot must be restarted; undocking is not required. Conversely, recoverable faults can be overridden on the surgeon’s console.
The console allows the operating surgeon to disable certain arms if isolated faults occur, but the camera cannot be controlled from the console after it has been disabled from the master control. If an emergency stop (on right side of console) is required, all instruments and the camera will remain in their last reported positions, and mechanical force on grasping devices will decrease. An emergency stop constitutes a recoverable fault and can be overridden on the surgeon’s console [43].
When instrument failures occur, the grip of the device may need to be manually released. This process begins with visualizing the offending instrument and then initiating an emergency stop. The grip release tool is then inserted onto the anterior portion of the device housing and turned clockwise for clip appliers and harmonic devices and counterclockwise for other instruments. The offending instrument can then be moved to a safe location and removed in the standard fashion. If the robotic arm is providing temporary hemorrhage control or critical visualization, an emergency stop can be initiated and the surgeon can leave the console and convert to laparotomy around the current instruments [43].
Reoperation and Adhesions
Anecdotally, the benefits of robotic surgery for complex revisional surgery and adhesiolysis center around a fusion of the hardware and software capabilities of an inorganic operating platform. Foremost, the robotic interface provides a stable camera and axis of control allowing the surgeon to perform fine movements. Wristed instrumentation then provides additional degrees of freedom, while robotic servos negate muscle fatigue and facilitate unwavering exposure, enabling the surgeon to perform prolonged adhesiolysis. Comparatively, muscle fatigue and fine tremor in laparoscopy is translated over the entire length of the instrument and becomes clinically significant during prolonged dissection.
With more than 95 % of abdominal surgeries leading to future adhesions and 10–20 % of individuals requiring additional surgical procedures following a colorectal or general abdominal surgery, intraabdominal adhesive disease is a regular obstacle for the colorectal surgeon [44]. Intuitively, adhesions make subsequent dissection more precarious, decreasing the quality of the resection and increasing overall morbidity [45, 46]. Other studies report an almost 15 % increase in major surgical-related complications in patients requiring adhesiolysis, but no decrease in the quality of the oncologic resection [47].
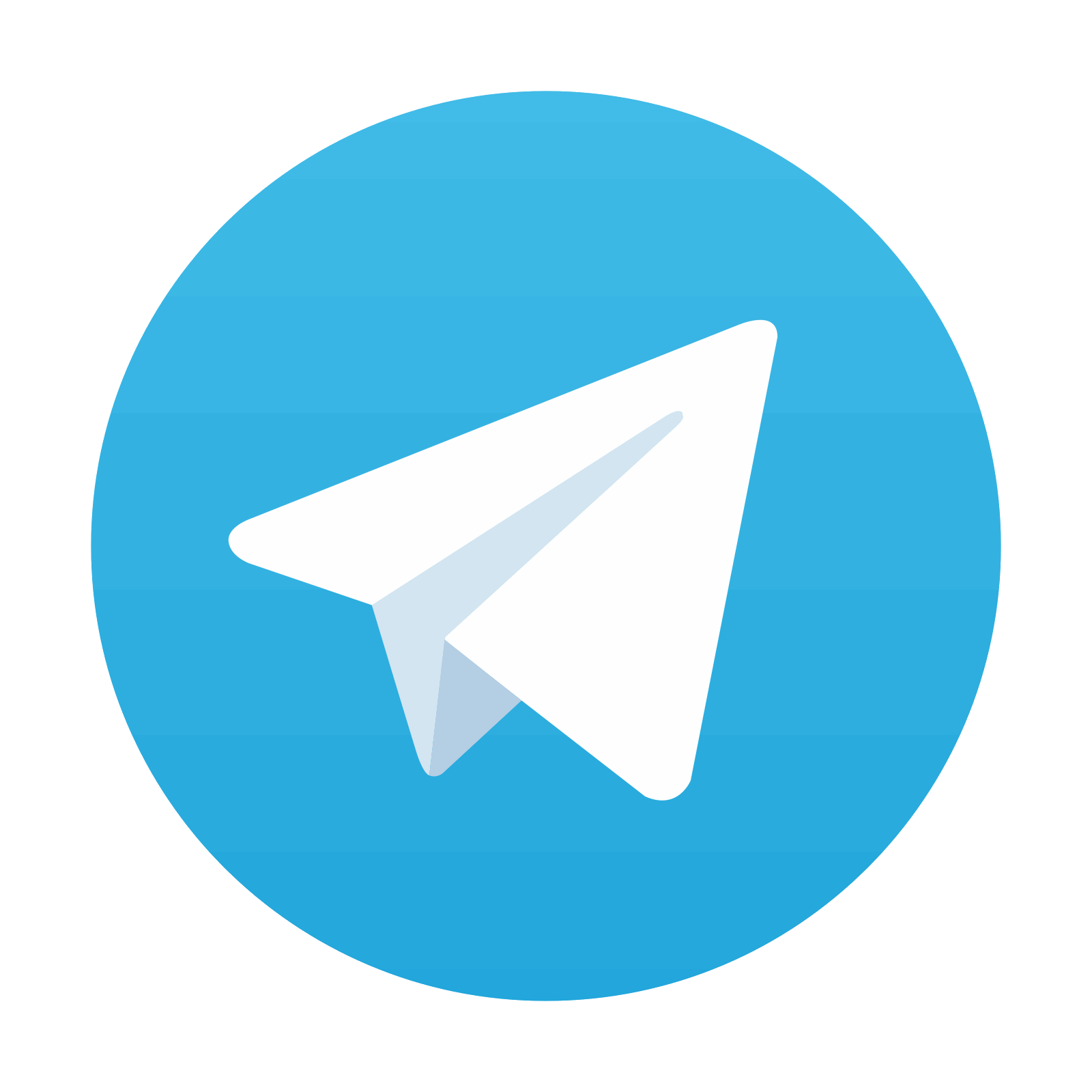
Stay updated, free articles. Join our Telegram channel

Full access? Get Clinical Tree
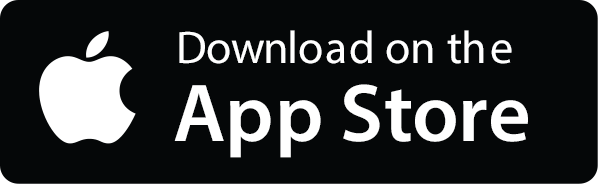
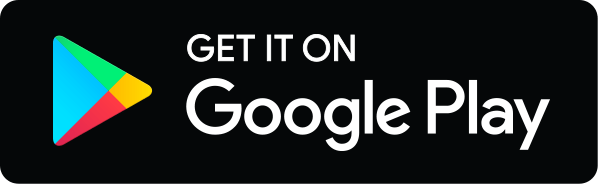