Abstract
Since their discovery > 60 years ago, tuft cells have intrigued and mystified researchers. Early studies focused on the unique morphology of tuft cells and their distribution pattern across mucosal surfaces, but their function remained unknown. The last 10 years have finally brought rapid advances in our understanding of intestinal tuft cells, including: (1) a detailed characterization of tuft cell ontogeny and associated lineage-specific transcriptional regulators in the small intestine; (2) accumulating evidence for a possible contribution of tuft cells to tumorigenesis; (3) identification of tuft cells as critical regulators of type 2 immune responses in the small intestine; and (4) experimental support for the long-standing hypothesis of chemosensing by tuft cells. Nonetheless, the homeostatic function of intestinal tuft cells remains unknown.
Keywords
Tuft cells, Brush cells, Chemosensing, Type 2 immunity
31.1
Overview
Since their discovery > 60 years ago, tuft cells have intrigued and mystified researchers. Early studies focused on the unique morphology of tuft cells and their distribution pattern across mucosal surfaces, but their function remained unknown. The last 10 years have finally brought rapid advances in our understanding of intestinal tuft cells, including: (1) a detailed characterization of tuft cell ontogeny and associated lineage-specific transcriptional regulators in the small intestine; (2) accumulating evidence for a possible contribution of tuft cells to tumorigenesis; (3) identification of tuft cells as critical regulators of type 2 immune responses in the small intestine; and (4) experimental support for the long-standing hypothesis of chemosensing by tuft cells. Nonetheless, the homeostatic function of intestinal tuft cells remains unknown.
31.2
Discovery
In the late 1920s, Dr. Chlopkow, a Russian scientist working at the state university in Tomsk, undertook a project to map out the developmental stages of intestinal goblet cells. Peering through his microscope, Dr. Chlopkow spotted a cell with a bundle of unusually long and thick microvilli protruding into the intestinal lumen. He sketched the cell in his notebook and included an illustration when writing up his work for publication ( Fig. 31.1 A ). Dr. Chlopkow concluded that he had found an early-stage goblet cell, and that the microvilli were lost during development. In fact, Dr. Chlopkow had provided the first report of a novel epithelial lineage now known as the tuft cell, but the “discovery” of these cells would take another 30 years.

With the emergence of electron microscopy came a more careful classification of epithelial cells based on their ultrastructural features. In 1956, Rhodin and Dalhamn described cells in the rat trachea marked by a cluster of long microvilli at their apical surface, and later that year Järvi and Keyriläinen found similar cells in the mouse stomach. It soon became clear that these cells constituted a distinct lineage that has been variously referred to as multivesicular, caveolated, fibrillo-caveolated, brush, and tuft cells. Here we will use the term tuft cell, which has emerged as the preferred nomenclature for these cells in the gastrointestinal tract.
31.3
Distribution
Generally, tuft cells are found in the columnar epithelium of endoderm-derived hollow organs. In rodents, they have been definitively identified in the trachea, the auditory tube, the thymus, the excretory ducts of salivary glands, the glandular stomach, the gall bladder and bile duct, the pancreatic duct, the small intestine, the cecum, the colon, and the urethra. Cells with tuft cell-like morphological features have also been described in the rat and perhaps human alveolar epithelium, but appear to be absent in mice, and a full characterization of these cells is still needed.
Besides rodents, tuft cells have also been described in various endoderm-derived tissues of fish, frogs, dogs, humans, and numerous other placental mammals. In this chapter we focus primarily on tuft cells of the rodent gastrointestinal tract, although many morphological features, cellular markers, and perhaps even functions appear to be shared across all anatomical sites and species.
In most cases tuft cells appear as isolated cells and constitute < 1% of the epithelium, but there are notable exceptions. In the mouse gall bladder and the rat bile and pancreatic ducts, tuft cells still appear as isolated cells but are far more abundant. Additionally, tuft cells have been shown to cluster around sphincters, such as the esophageal sphincter and the sphincter of Oddi, and in the mouse stomach tuft cells make up ~ 30% of the cells at the gastric groove that divides the forestomach and corpus. However, this gastric groove does not exist in humans.
31.4
Morphology
The ultrastructure of tuft cells, as revealed by transmission electron microscopy, freeze-fracture microscopy, and scanning electron microscopy, is remarkably similar across all tissues and species examined to date. The iconic feature of tuft cells is the cluster of microvilli at the apical surface ( Fig. 31.1 C–E). These microvilli are thicker and longer than those of neighboring cells and give tuft cells their name. Other consistent features of tuft cells include: (1) a relatively narrow apical membrane, which leads to the impression that tuft cells are pinched at the top ( Fig. 31.1 B and E); (2) prominent actin microfilaments that begin at the tips of the villi and extend into the cell, terminating just above the nucleus ( Fig. 31.1 E and F); (3) abundant but largely empty apical vesicles that form a complex tubulovesicular network ( Fig. 31.1 F); (4) a network of microtubules that runs among the microfilaments and the tubulovesicular structures; (5) a Golgi apparatus on the apical side of the nucleus; (6) a lack of rough endoplasmic reticulum and secretory vesicles, and (7) desmosomes and tight junctions that anchor tuft cells to their neighboring cells ( Fig. 31.1 G). Collectively, these features define the prototypical tuft cell depicted in Fig. 31.2 .

While there is widespread agreement about the features described above, other aspects of tuft cell morphology remain in dispute or have only been described once or twice. For example, some tuft cells have lateral microvilli that extend into neighboring cells and contain the same microfilaments as those at the cell surface ( Fig. 31.1 G). Several papers also described abundant glycogen deposits in tuft cells, but this was not universally noted. Additionally, one study found that the membrane of tuft cell microvilli is particularly cholesterol-rich compared to nontuft epithelium, and staining with the lectin UEA-1 demonstrated that the apical glycocalyx of tuft cells, which is generally thinner than on surrounding cells, contains abundant fucose residues. The functional relevance of these observations remains to be determined.
There are also areas of disagreement or discrepancy. For example, while most studies noted the elongated appearance of mitochondria, one study noted an abundance of mitochondria, while another commented on their paucity. The precise nature of the apical tubulovesicular network also remains in dispute. Early studies using isolated thin sections described only abundant vesicles, but serial sectioning and 3D imaging later revealed the complex interconnected network of hollow structures ( Fig. 31.3 ). The presence of this tubulovesicular network is now widely accepted, but it remains unclear whether it is continuous with the intestinal lumen. Nabeyama and Leblond argued that the invaginations (or caveolae) between microvilli at the apical surface connect to the tubulovesicular network, but this has been challenged by others.

How do we explain the discrepancies noted above? It is possible that different fixation protocols could reveal or mask certain structures or even give rise to artifacts. More interestingly, these differences may reflect the underlying biology of tuft cells, such as tissue-specific specialization and/or dynamic changes in tuft cells that occur following a meal or other shift in intestinal physiology. It has been suggested, for example, that a dynamic tubulovesicular network would allow tuft cells to rapidly alter their luminally exposed surface area. Perhaps live-cell imaging of tuft cells could provide additional insights.
31.5
Markers
While electron microscopy facilitated the initial discovery and characterization of tuft cells, several excellent tuft cell markers are now available for antibody-based immunofluorescence and immunohistochemistry ( Table 31.1 ).
Expression in Tuft Cells | Expression in Other Epithelial Cells | References | |
---|---|---|---|
Structural | |||
acTUB | All (apical accumulation) | None reported | |
CK18 | All | Some weak staining | |
DCLK1 | All | Rare enteroendocrine and stem cells; induced in tumors | |
UEA-1 | All | Most cells in ileum | |
VIL1 | All (apical accumulation) | All (no apical accumulation) | |
Chemosensing | |||
CHAT | All | None reported | |
GNAT3 | Some | Some enteroendocrine cells | |
PLCB2 | Some | Some enteroendocrine cells | |
Taste receptors | Some | Some enteroendocrine cells | |
TRPM5 | All | Rare enteroendocrine cells | |
Other | |||
GFI1B | All | None reported | |
HPGDS | All/most | None reported | |
POU2F3 | All | None reported | |
PTGS1 | All | None reported | |
SIGLECF | All/most | None reported |
31.5.1
Structural
One set of tuft cell markers derive from the unique cell biology of tuft cells. For example, the first antibodies used to identify tuft cells labeled the actin-binding proteins villin 1 (VIL1) and fimbrin (a.k.a. plastin 1). Although both proteins localize to the apical membrane of all intestinal epithelial cells, the immunofluorescence signal is concentrated in the microvilli of tuft cells, making them readily distinguishable. Of note, while the Vil1 promoter is often used to drive “intestine-specific” expression of the Cre recombinase in mice, tuft cells express Vil1 in the airways and likely other nonintestinal tissues as well. Another example of structure-based tuft cell staining is the lectin Ulex europaeus Agglutinin I (UEA-1), which binds to abundant fucosylated proteins on the apical surface of tuft cells. In the duodenum and jejunum UEA-1 labeling is quite selective for tuft cells, but in the ileum UEA-1 broadly labels epithelial cells. Antibodies against cytokeratin 18 (CK18) and acetylated tubulin (acTUB) are also widely used and, among epithelial cells, are largely selective for tuft cells, although weak staining of nontuft epithelium by CK18 has been noted. Another excellent structure-based marker of tuft cells is doublecortin like kinase 1 (DCLK1), a microtubule-associated kinase first characterized in neurons. All tuft cells express DCLK1 and > 95% of DCLK1 + epithelial cells are tuft cells. Weak expression has, however, been described in insulinoma-associated 1 (INSM1) positive enteroendocrine cells, and epithelial expression of DCLK1 has been reported in tumors and perhaps also rare cancer stem cells.
31.5.2
Chemosensing
Remarkably, tuft cells share structural similarities with taste buds and express components of a canonical taste transduction pathway. The functional relevance of this pathway is discussed below. Here we focus on the utility of proteins in the taste transduction pathway as tuft cell markers. In taste buds, bitter or umami ligands bind cognate surface-expressed G-protein-coupled taste receptors ( Fig. 31.4 ). These receptors activate guanine nucleotide binding protein alpha transducing 3 (GNAT3; a.k.a. alpha-gustducin) to trigger phospholipase C beta 2 (PLCB2)-mediated inositol trisphosphate (IP3) production and release of intracellular Ca 2 + stores. The rise in Ca 2 + then opens transient receptor potential cation channel 5 (TRPM5) on the cell surface, allowing influx of Na + and depolarization of the cell, which drives release of acetylcholine that binds adjacent sensory nerve terminals. In the intestinal epithelium, taste receptors, GNAT3, and PLCB2 are expressed by some, but not all tuft cells, and their utility as tuft cell markers is further decreased by their expression in some enteroendocrine cells. TRPM5 is a better tuft cell marker. Experiments using anti-TRPM5 antibodies and Tg( Trpm5 -GFP) mice found that all tuft cells are TRPM5 + , and that besides tuft cells, only taste receptor cells, beta cells in the pancreas, and a few cells in the duodenal gland with enteroendocrine-like morphology express TRPM5. Lastly, tuft cells and taste buds also share expression of choline acetyltransferase (CHAT), the enzyme that acetylates choline to generate the neurotransmitter acetylcholine. Among epithelial cells, tuft cells throughout the intestinal tract are selectively marked in CHAT reporter mice and by antibody labeling of CHAT.

31.5.3
Others
In 2008, our understanding of tuft cells was advanced significantly by a study that used Tg( Trpm5 -GFP) mice to sort tuft and nontuft epithelial cells for transcriptome analysis by microarray. This study confirmed selective expression in tuft cells of the markers discussed above, and also suggested new pathways of interest. In particular, the authors noted that tuft cells express all of the enzymes required for biosynthesis of prostaglandins and leukotrienes—lipid-derived signaling molecules. Accordingly, while antibody labeling of prostaglandin-endoperoxide synthase 1 (PTGS1) or hematopoietic prostaglandin D synthase (HPGDS) marks many hematopoietic cells in the intestinal lamina propria, among epithelial cells only tuft cells are PTGS1 or HPGDS positive. The microarray study also identified sialic acid binding Ig-like lectin F (SIGLECF) as a surface marker that is selectively expressed in tuft cells. SIGLECF together with epithelial cell adhesion molecule (EPCAM) labeling of epithelial cells has been used to identify and sort tuft cells by flow cytometry, but whether all tuft cells or just a subset express SIGLECF remains uncertain.
In summary, while several antibodies appear to label all tuft cells, there is always some concern about expression in other epithelial cells. Nonetheless, the overwhelming majority (> 95%) of DCLK1, TRPM5, or PTGS1 positive intestinal epithelial cells are tuft cells, and definitive identification of tuft cells can be achieved by pairing these markers with differential interference contrast microscopy to identify unique tuft cell morphology or, in the case of DCLK1, with the enteroendocrine marker INSM1 to exclude the rare DCLK1 + enteroendocrine cells ( Fig. 31.5 ). Lastly, as discussed in detail below, POU2F3 and GFI1B were recently proposed as tuft cell specific transcription factors and may eventually become the markers of choice.

31.6
Ontogeny and Maintenance
Villus formation and differentiation of the intestinal epithelium occurs in the latter stages of embryogenesis (E14.5–15.5 in mice ), and the first tuft cells appear soon afterwards. Two studies have identified tuft cells in the small intestine of mice at E16.5–18.5, and tuft cells were noted in a 16-week-old human fetus. Tuft cells appear somewhat later in the mouse stomach than in the small intestine, and the number of tuft cells clustered at the gastric groove increases steadily until 21 days post weaning. Interestingly, it seems that in rats tuft cells do not appear until just after birth.
The epithelial stem cell compartment of the small intestine is used as a model system for stem cell biology and is therefore well characterized. These epithelial stem cells are marked by leucine rich repeat containing G-protein-coupled receptor (LGR5) expression and reside at the base of the intestinal crypts, where they are interspersed with Paneth cells that help form the stem cell niche. Nascent epithelial cells enter the transit amplifying zone just above the stem cell compartment, where they continue to divide and adopt their terminal fates before leaving the crypt. Except for Paneth cells, which migrate downward following differentiation, emerging cells continually push the epithelial sheet upwards and cells are extruded as they reach the tips of the villi. As a result, the entire epithelium of the small intestine is replaced every 3–5 days. Despite this rapid turnover, cellular replication is completely restricted to the crypts and therefore mature epithelial cells, including tuft cells, never express replication markers such as Ki67. Additionally, lineage tracing experiments in Lgr5-creERT2;Rosa26-LacZ mice have confirmed that tuft cells arise from LGR5 + stem cells, and that their half-life is similar to that of other epithelial cells. Overall, there is little evidence to suggest that the development, adult maintenance, and half-life of tuft cells in the small intestine is much different than that of their surrounding epithelium. The identity of adult stem cells that replenish the epithelium of other gastrointestinal tissues, such as the pancreas, gall bladder, and stomach, remain poorly defined. Similarly, it is generally unknown how tuft cells arise in these tissues.
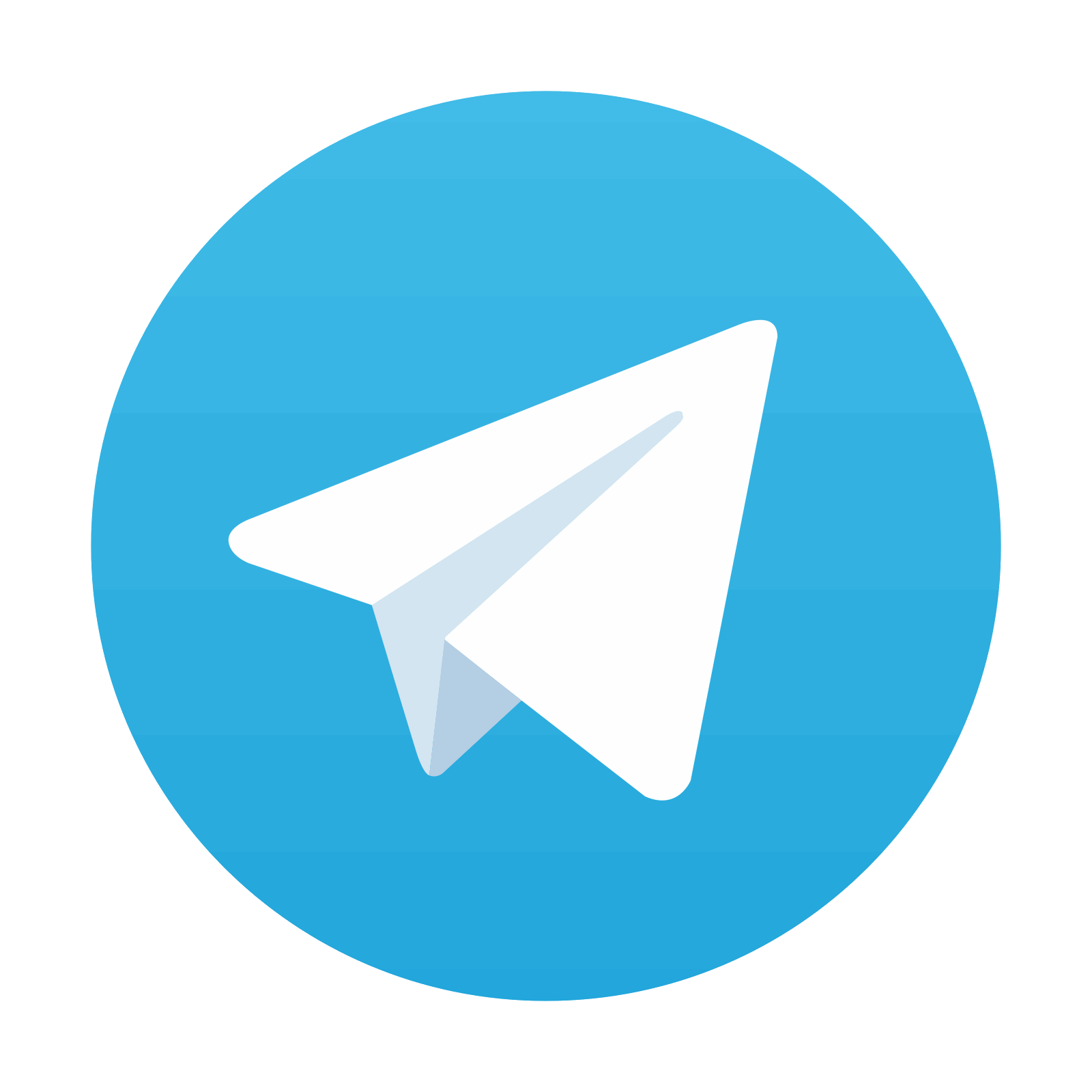
Stay updated, free articles. Join our Telegram channel

Full access? Get Clinical Tree
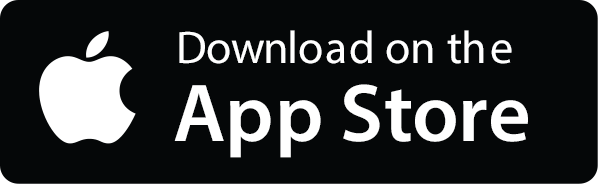
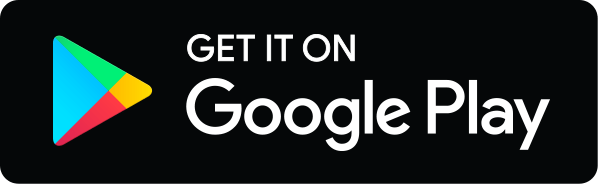