(1)
Technische Universität München, Gregor-Mendel-Str. 2, Freising-Weihenstephan, 85354, Germany
Inflammatory bowel diseases (IBD) include the two main phenotypes Crohn’s disease (CD) and ulcerative colitis (UC) both characterized by intermittent conditions of chronic and relapsing inflammation in the entire gastrointestinal tract or colon, respectively. Disease initiation and perturbation is triggered by environmental factors in genetically susceptible individuals [1, 2]. Genome-wide association studies (GWAS) and animal data provide evidence for an interrelated role of the intestinal microbiota, epithelial interface, and immune system in the pathogenesis of IBD [3–6]. This chapter provides an overview of the current state of research on the role of the intestinal microbiota in CD and UC. In addition, approaches to the treatment of IBD by the modulation of the intestinal microbiota are discussed.
Structure and Functionality of the Intestinal Microbiota
The human intestinal microbiota encompasses a variety of microorganisms, including bacteria, archaea, viruses, yeasts, and protozoa. The totality of these microbes and their genes is referred to as “intestinal microbiome” [7]. The microbial colonization of the intestine of newborn depends on the type of delivery (natural birth versus caesarean section ) and the type of food intake (breastfeeding versus bottle milk ) [8]. The first 2 years of life intestinal communities undergo substantial compositional fluctuations reacting to the new environmental cues, such as changes in dietary patterns , and thus it represents a crucial phase for the maturation of the intestinal microbial ecosystem [9–11]. After this critical phase, a relatively stable adult microbiota is established and interference factors (i.e., antibiotics, drugs, infections, excessive hygiene, nutrition, lifestyle) may affect its composition leading to serious long-term consequences [12–16]. For instance, long-term dietary patterns have a significant influence on the selection of specific microbial networks, the so-called enterotypes . A diet rich in animal fat and protein is associated with the Bacteroides enterotype, while a high-carbohydrate diet corresponds to the Prevotella enterotype [13, 17]. The mammalian gut microbiota comprises several hundred different bacterial species, many of which have a beneficial effect on the host and correspond to up to 1012–1014 organisms/g of colon content, exceeding greatly the number of eukaryotic cells. The totality of all microbial gene of the microbiota is called the metagenome and latest estimations suggest a reference catalogue of 9.9 million genes [18, 19]. The adult intestinal microbiota is dominated by the two phyla Bacteroidetes and Firmicutes accompanied at much lower abundance by Actinobacteria and Proteobacteria [7, 20–22]. At the species level, high-throughput 16S rRNA gene sequencing and metagenomic analysis allow the detection of approximately 100–200 operational taxonomic units (OTU) per individual [19, 23]. It should be pointed out that each human being has an individual gut microbiota composition, therefore the relative amounts of different phyla varies considerably between individuals. Overall, more than 1000 different species of bacteria were detected in human fecal samples and biopsies [7]. The high interindividual variability of the intestinal microbiota in healthy people hampers the identification of a reference microbiota. Nevertheless, metagenomic analysis revealed a core functional gut microbiome consisting in approximately 60 bacterial gene families shared by individual subjects with differences in bacterial phylotypes. Thus, different combinations of bacterial species, belonging to a common ecological niche, are able to carry out functions necessary for survival in the gut resulting in a stable microbiota [7, 21, 24, 25]. Hence, an extensive understanding of the functions of the microorganisms is crucial in order to explain the complex intestinal ecosystem and its interaction with the host. Integration of data from metagenomic, metatranscriptomic, metaproteomic, and metabolomics studies may aid to gain insights into the host–microbiota interactome [26]. Elucidating the functions of the microbiota is of enormous physiological relevance for humans, considering the central role the intestinal ecosystem exerts on host immune and metabolic functions [27]. The evidence that many chronic diseases, such as allergies, metabolic conditions, and autoimmune diseases, including IBD, are associated with compositional shifts of the gut microbiota in clinical and/or animal studies underlies the therapeutic importance of this field of research [28–31].
Factors Influencing the Intestinal Microbiota Composition
Under physiological conditions the microbiota is highly resilient to perturbations, such as moderate fluctuations in change in dietary-patterns, smoking, drugs or antibiotics. These factors, the so-called exposomal components , might temporarily or permanently modify the microbiota composition leading the bacterial ecosystem to stabilize within a new “alternative state” [32]. The ability of the microbiota to adapt to alterations in the intestinal milieu maintains intestinal homeostasis. In addition, a variety of host factors , including gender, genotype, age, psychological stress, and health status have been reported to shape the intestinal microbiota [8, 33].
The increased incidence of IBD in more developed country is speculated to be partially due to westernized dietary habits and overuse of antibiotics that disrupt mechanisms involved in development of immune tolerance altering dramatically the microbiota composition [15, 34, 35].
Smoking (e.g., nicotine) is an example of a disease specific modifier that seems to exacerbate CD [36] increasing the risk of surgical and clinical recurrence [37], while being protective against UC [38]. One explanation for the dichotomous effect of smoking in patients with UC and CD is based on the evidence that smoking (e.g., nicotine) impairs autophagy [39]. In contrast, recent findings in animal models of IBD suggested that the cytokine milieu characteristic of UC or CD may differentially regulate the expression of nicotinic receptors harbored by colonic CD4 T cells and therefore inhibiting or promoting their pro-inflammatory function [40].
Drug therapy administered to CD patients , such as anti-TNF-α antibody treatment, has been investigated for its ability to alter the microbiota composition, showing an increase in Faecalibacterium prausnitzii [41, 42]. This evidence indicates that this species is affected by inflammation and reducing it leads to a repopulation. This suggests that the inflammatory milieu may impact the microbiota composition selecting a specific combination of bacterial species.
The Role of the Intestinal Microbiota in IBD
Several lines of evidence implicate the microbiota in the pathogenesis of IBD. For instance, animal models for chronic ileitis and colitis require the presence of gut microbiota to trigger the disease [43–47]. Additionally, inflammation occurs at sites with higher bacterial concentration and the administration of antibiotics usually leads to a reduction of inflammation in IBD patients and animal models [44, 48, 49]. The central role of the intestinal microbiota in the development of IBD was confirmed by GWAS and animal studies. Currently, 163 variant loci are associated with an increased risk of IBD. Their functions are attributed to microbial recognition or defense, epithelial cell function or the activation and regulation of innate as well as adaptive immune functions [50]. In line with this, IBD patients harbor exacerbated immune responses directed against the normal intestinal microbiota due to aberrant immunoregulatory mechanisms and/or mucosal barrier defects . In fact, IBD patients exhibit spatial and compositional modifications within the gut compared to healthy individuals. Clinical data report that the rigorous spatial separation between the epithelial surface and the intestinal microbiota is ablated in biopsies from CD patients [51–53]. The number of mucosa-associated microorganisms increase and the distance between the intestinal microbiota and the intestinal epithelium is reduced by a thinner or more permeable mucous layer [1, 54]. A large number of studies have reported that IBD patients display compositional changes in the microbiota, so-called dysbiosis , compared to healthy individuals [1, 55–59]. Dysbiosis can be defined an alteration in the bacterial ecosystem associated to pathology [55, 60–62]. Intestinal dysbiosis has been associated with a variety of human disease, other than IBD, such as irritable bowel syndrome [63], chronic diarrhea [64], obesity [65], diabetes [31], autism [66], colorectal cancer [67], cardiovascular [68], and liver disease [69]. It is still unclear to what extent the observed changes of the intestinal microbiota are a cause or consequence of the disease, or to what extent they contribute to the further course of the disease .
Intestinal Microbiota Composition in IBD
Several studies compared the gut microbiota of IBD patients and healthy individuals in order to determine microbial signatures associated with disease or healthy status. Changes in the microbiota composition in IBD encompass decrease in α-diversity [61, 70, 71], in Firmicutes , including Erysipelotrichales and Ruminococcaceae [72], especially F. prausnitzii [41, 58, 59], in Bacteroidales, specifically Bacteroides fragilis and vulgatus [73], with concomitant overrepresentation of Fusobacteria [72, 74], of Firmicutes , such as Veillonellaceae [72], and of Gammaproteobacteria , i.e., Pasteurellaceae [75] and Enterobacteriaceae , such as Escherichia coli (E. coli) and specifically adherent-invasive E. coli (AIEC) [60, 74, 76, 77]. It has been observed that infectious bacteria, such as AIEC and mycobacterium avium subsp. paratuberculosis, are frequently associated with the pathogenesis of inflammation in CD patients [77–81]. This observation leads to the tempting speculation that transient infection with pathogens could act as environmental trigger to initiate inflammatory responses. These responses may become chronic and persistent under the stimulation offered by commensal bacteria once the pathogen has been cleared by the host. It is still debated whether bacterial infection is causal or secondary to underlying immune dysregulation in CD patients, such as macrophages unable to clear pathogens in the lamina propria of CD patients [82]. Furthermore, it is suggested that inflammation may lead to modifications in the composition of gut bacterial communities. Animal studies show that the induction of acute inflammation, for example, by chemical substances, such as DSS [83–85], or to a less extend by infectious bacteria such as Citrobacter rodentium [83], can cause changes of the intestinal microbiota.
In a twin cohort study, the authors showed that patients with ileal CD had significantly lower levels of Faecalibacterium and Roseburia and higher levels of Enterobacteriaceae and Ruminococcus compared to their discordant healthy twins [56, 62]. Another study carried out in UC patients and their discordant twins revealed the presence of a dysbiotic microbiota in UC subjects characterized by a reduced bacterial diversity and more Actinobacteria (mostly Rhodococcus genus ) and Proteobacteria (mainly Enterobacteriaceae , i.e., Shigella/Escherichia) than that of their healthy siblings [61]. This evidence is of particular importance because it indicates that the gut microbiota composition is affected much more by the disease state rather than the genetic component in the context of ileitis and colitis.
IBD patients displayed an unusually higher amount of mucosa‐associated aerobic and facultative-anaerobic bacteria [61, 86, 87], supporting the oxygen hypothesis [88]. This theory proposes that an increase in oxygen tension in the gut leads to a bloom of facultative anaerobes and to the ablation of obligate anaerobes. A well-recognized example of bacterial species highly reduced in IBD patients and dramatically affected by oxygen levels is Faecalibacterium prausnitzii [58, 59]. Similarly, the pullulation of facultative-anaerobic members of Enterobacteriaceae, especially E. coli, has been reported in IBD patients [60, 74, 76, 77]. It has been proposed that alteration in oxygen composition may be due to the inflammation status which is characterized by an oxidative burst in the intestinal tissue with release of reactive oxygen species (ROS) [89]. An attractive and innovative strategy will be to balance dysbiosis by modulating the level of oxygen in distal segments of the gut, for instance via drugs or probiotics selected for their ability to consume oxygen. In line with this, it is possible to speculate that small bowel resection, frequently performed in CD to eliminate the inflamed gastrointestinal portion, causes per se the exposition of gut segments to atmospheric oxygen. Thus, bacteria that are able to cope with oxidative stress would be favored to proliferate and this dysbiosis would lead to disease reoccurrence.
Concomitant with changes in microbiota composition, IBD patients display alterations in function and metabolic capacity of the intestinal microbiota. The decreased production of short chain fatty acids (SCFAs) or the ability of host tissues to use SCFAs might be altered under conditions of chronic inflammation [90]. For instance, butyrate is a major nutrient for enterocytes and lack of this energy source leads to energy deprivation, to impaired barrier function, and to increased bacterial translocation [91]. Several butyrate-producing bacterial species, such as Roseburia hominis and Faecalibacterium prausnitzii, were found to be decreased in UC patients compared to their healthy siblings [61], or to healthy controls [92]. By harboring anti-inflammatory properties [93–95], these bacteria may represent an attractive strategy for therapeutic intervention. Another possibility is to establish these indicator species as diagnostic markers for IBD. Examples are provided by studies on the butyrate-producing bacterium Butyricicoccus pullicaecorum which is underrepresented in fecal samples from IBD patients and it is able to reduce trinitrobenzenesulfonic acid-induced colitis in rats [96].
Genetic Background Contribution in IBD
The contribution of genetic factors to the etiology of IBD has been estimated in monozygotic twins to be lower than 50 %, indicating a more predominant role for environmental factors in the development of the disease [56, 97]. Nevertheless, host genomic loci (i.e., NOD2, ATG16L1, and XBP1) have an impact on the composition and functionality of the bacterial community [14, 98, 99]. This concept has been supported by in vivo data generated in Nod2- and Xbp1-deficient mice, which harbor dysbiotic microbiota compared to the WT counterparts [45, 100]. However, these genetically driven changes are not sufficient to trigger the development of IBD, as confirmed by studies in germ-free mice deficient in specific IBD susceptibility loci [45]. Further evidence has been provided by clinical data, which report a reduced diversity of mucosa-associated bacteria in UC patients and in their discordant monozygotic twins compared to healthy subjects [3, 61, 101]. This observation emphasizes that familial aggregation, and thus genetic background and environment, may promote low microbial diversity. How these factors favor the development of IBD in some individuals and not in their siblings is still a forum of speculations. Another piece of evidence supporting the influence of IBD susceptibility loci on the microbiota composition is that subtle immunodeficiency, such as ATG16L1 and NOD2 deficiency, licenses AIEC bacteria to proliferate as a consequence of decreased capacity to kill intracellular organisms due to defective autophagy [102, 103].
Intestinal Microbiota–Immune System Interaction in IBD
Many studies investigated and reviewed the role of the intestinal microbiota in driving the development and maintenance of the gut immune system [104–106]. Members of the microbiota or their metabolites can activate anti-inflammatory immune response by the induction of regulatory T-cells [107]. On the other hand, T cell subpopulations may also cause shifts in bacterial composition of the microbiota, and thereby contribute to dysbiosis and inflammation. Pathogenic NKG2D expressing CD4+ T-cells are preferentially recruited to the ileum in CD and their presence in non-inflamed gut segments correlates with post-resection relapse [108–110]. These cells induce Th17-like responses secreting high level of interleukin (IL)-17 and IL-22 and their clonal expansion is bacterial antigen-specific [109].
It is possible to speculate that the depletion of bacterial species or the selection of more aggressive ones may enhance bacterial recognition by the immune system promoting pro-inflammatory responses, fueling the question whether dysbiosis may lead to immune system dysregulation. An example is provided by a recent study which reports an increased number of cells expressing CD11c and TLR4 in mesenteric lymph nodes of DSS-treated mice. The high number of these enriched immune cells correlates with the increasing amount of mucin-degrading Enterobacteriaceae and Akkermansia in the colonic mucosa [84]. This evidence is in agreement with clinical data indicating an increase in TLR4 expressing dendritic cells in inflamed tissue of UC patients [111].
Manipulation of the Intestinal Microbiota in IBD
Despite the strong correlative evidence of changes in the gut microbial ecosystem and disease activity, functional prove for the causative nature of microbe–host interactions especially the role of complex changes in community structure (dysbiosis) and related clinical adaptation is still lacking in IBD.
At present, microbial therapies are intensively studied with the aim to influence the disease activity by modulating the intestinal milieu. Four therapeutic intention strategies are applied to modulate the intestinal microbiota including the administration of antibiotics, prebiotics (i.e., dietary components that promote the growth and metabolic activity of beneficial bacteria), probiotics (i.e., beneficial bacteria), or fecal transplantation (bacteriotherapy).
Antibiotic treatment is well-known to affect microbiota composition leading to the reduction or elimination of specific taxa within microbial communities [112]. This is an efficient way to eradicate infectious organisms but on the other hand the chronic use of antibiotics may promote dysbiosis [113]. Especially the repeated consumption of antibiotics at an early age lead to persistent disturbances of the microbial ecosystem and associates with an increased risk for the later occurrence of IBD [113, 114].
Several clinical studies have reported the efficacy of probiotics , such as Escherichia coli Nissle 1917, Lactobacillus GG, Bifidobacterium longum, Lactobacillus reuteri, and VSL#3, in maintaining remission in UC [115–120]. In contrast, clinical trials of probiotics in CD have generated heterogeneous results and up to date probiotics have shown to be ineffective in inducing or in maintaining remission in CD patients [121, 122]. Clinical improvement has been achieved with a combination strategy, the so-called symbiotics , of probiotic bacteria, e.g., B. longum, and prebiotics [123]. These data suggest that combination strategies including bacteriotherapy and milieu modifiers offer an additional approach to treat CD.
While the intake of probiotics has only a minor influence on the intestinal ecosystem, relevant changes in the microbiota of the recipient may be induced by the introduction of complex bacterial communities through bacteriotherapy, the so-called fecal microbiota transplantation (FMT) . In patients with refractory Clostridium difficile-associated diarrhea, therapeutic success can be achieved by FMT, which corresponds to the transfer of a “normal” microbiota [124, 125]. The potential of bacteriotherapy is intriguing and applicable to diseases associated with intestinal dysbiosis. Nevertheless, the therapeutic efficacy of FMT in IBD patients is not yet clear. Borody and colleagues exploited FMT to successfully treat six patients with refractory UC [126]. A patient with severe CD complicated by refractory Clostridium difficile infection has been successfully treated by FMT [127]. A pilot study with 30 refractory CD patients showed clinical improvement and clinical remission (>76 %) after FMT up to 15 months follow up [128]. However, a systematic review in 2014 of FMT for IBD revealed that only half of the patients experienced symptoms improvement, disease remission and cessation of medication after FMT treatment [129]. Another systematic review in the same year described studies which report a success rate for the treatment of CD via FMT in the range of 80–100 %, indicating that the outcome of FMT is patient-specific [130], and in some cases no clinical benefit of FMT has been observed [131]. Two recent double-blind randomized control trials investigated the efficacy of FMT in UC patients. While the study from Moayyed et al. reported efficacy of FMT in active UC [132], the trial carried out by Ponsioen group showed that FMT may be beneficial only for some patients [133]. The contrasting results may be explained by the different approach adopted by the two research teams, including mode of administration and the composition and the dose of the donor microbiota used .
Conclusion
A growing body of evidence indicates that the intestinal microbiota play an essential role in IBD pathogenesis, potentially by exacerbation of host immune responses in genetically susceptible host. Consequently, the modulation of microbial communities is an attractive therapeutic option. Despite the residual risk of transmission of pathogens, the unresolved long-term effects, as well as the use of functionally uncharacterized bacterial consortia, bacteriotherapy represents a more promising therapeutic approach to restore eubiosis.
References
3.
Buhner S, Buning C, Genschel J, Kling K, Herrmann D, Dignass A, et al. Genetic basis for increased intestinal permeability in families with Crohn’s disease: role of CARD15 3020insC mutation? Gut. 2006;55(3):342–7.PubMedPubMedCentral
4.
Wehkamp J, Salzman NH, Porter E, Nuding S, Weichenthal M, Petras RE, et al. Reduced Paneth cell alpha-defensins in ileal Crohn’s disease. Proc Natl Acad Sci U S A. 2005;102(50):18129–34.PubMedPubMedCentral
5.
Xavier RJ, Podolsky DK. Unravelling the pathogenesis of inflammatory bowel disease. Nature. 2007;448(7152):427–34.PubMed
6.
Cobrin GM, Abreu MT. Defects in mucosal immunity leading to Crohn’s disease. Immunol Rev. 2005;206:277–95.PubMed
7.
Human Microbiome Project C. Structure, function and diversity of the healthy human microbiome. Nature. 2012;486(7402):207–14.
8.
Dominguez-Bello MG, Costello EK, Contreras M, Magris M, Hidalgo G, Fierer N, et al. Delivery mode shapes the acquisition and structure of the initial microbiota across multiple body habitats in newborns. Proc Natl Acad Sci U S A. 2010;107(26): 11971–5.PubMedPubMedCentral
9.
Koenig JE, Spor A, Scalfone N, Fricker AD, Stombaugh J, Knight R, et al. Succession of microbial consortia in the developing infant gut microbiome. Proc Natl Acad Sci U S A. 2011;108 Suppl 1:4578–85.PubMed
10.
Turnbaugh PJ, Ridaura VK, Faith JJ, Rey FE, Knight R, Gordon JI. The effect of diet on the human gut microbiome: a metagenomic analysis in humanized gnotobiotic mice. Sci Transl Med. 2009;1(6):6ra14.PubMedPubMedCentral
11.
Cotillard A, Kennedy SP, Kong LC, Prifti E, Pons N, Le Chatelier E, et al. Dietary intervention impact on gut microbial gene richness. Nature. 2013;500(7464):585–8.PubMed
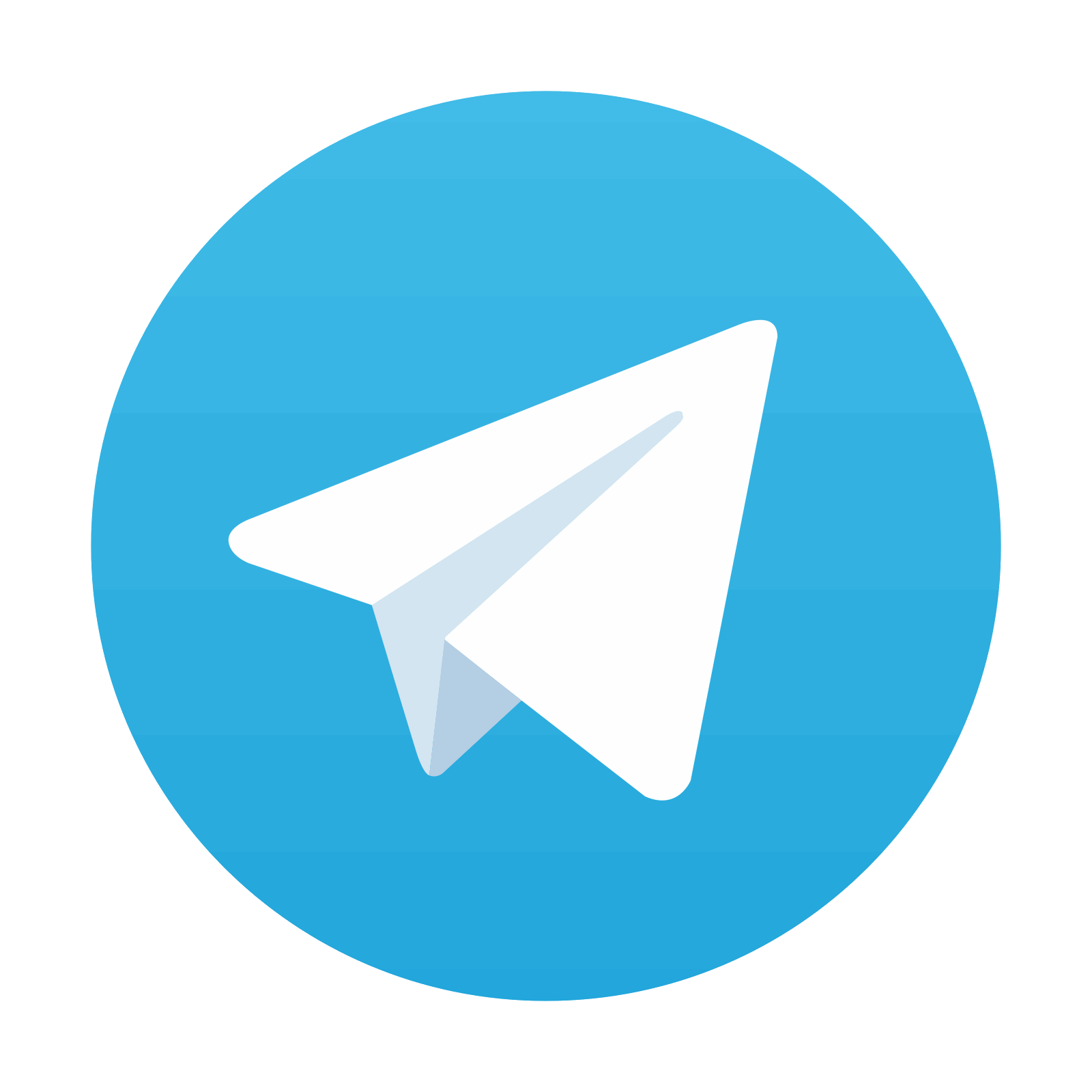
Stay updated, free articles. Join our Telegram channel

Full access? Get Clinical Tree
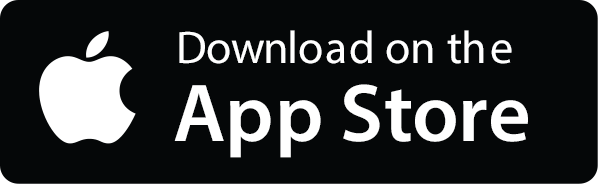
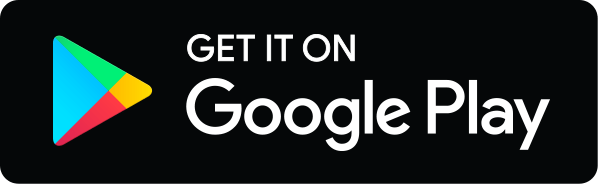
