Abstract
The objective of this chapter is to provide an overview of our current understanding of the mechanisms underlying absorption of predominant anions [chloride and short-chain fatty acids (SCFAs)] and the regulation of anion transporters in the mammalian intestine. As a result of the availability of knockout mouse models and models of intestinal diseases, novel mechanisms regulating the expression, trafficking, and function of the key anion transporters in response to various physiological and pathophysiological conditions have been elucidated. New data on the fine structural details of the anion transporters (such as the N-glycosylation) and the role of some of these anion transporters as tumor suppressors have come to surface. This has been reviewed in detail in this chapter. In addition, this chapter also reviews briefly the anion transporters involved in the absorption of sulfate and oxalate. However, mechanisms of absorption of phosphate will not be included here and will be presented in another chapter of this book. Since, the general mechanisms of intestinal electrolyte transport and the physiology of their integration are being addressed elsewhere in the book, the focus of the current chapter is, therefore, more on recent advances in the molecular identity and regulation of the transporters involved in Cl − and SCFA − absorption under normal and disease states.
Keywords
SLC26A3, SLC26A6, Short-chain fatty acid, Monocarboxylate transporter-1, Intestinal chloride transport, Anion absorption, Oxalate transport, Sulfate transport
Acknowledgments
Studies in the author’s laboratories were supported by NIDDK grants RO1 DK54016 (PKD), RO1 DK81858 (PKD), RO1 DK92441 (PKD), RO1 DK71596 (WAA), RO1 DK109709 (WAA) and Department of Veterans Affairs Merit Awards BX002011 (PKD), BX000152 (WAA), BX002687-01 (SS) and VA Senior Research Career Scientist Award (PKD), Research Career Scientist Award (WAA).
57.1
Mechanisms of Intestinal Chloride Absorption
57.1.1
Chloride Absorption in the Intestine
Chloride is an inorganic anionic halogen that occurs primarily in the extracellular fluid compartment (the blood/plasma and the interstitial fluid compartment). Its efficient absorption is critical to maintain the optimal levels of this “essential” mineral nutrient in the body. In the mammalian intestine, chloride is avidly absorbed throughout the intestinal tract during postprandial and fasting states. The mechanism of chloride transport exhibits significant heterogeneity among different species and across different intestinal segments and regions. Chloride is also secreted into the intestinal lumen via chloride channels that are stimulated by intracellular cAMP and Ca 2 + . Disturbances of chloride movement across the luminal membrane of the intestinal epithelial cells are implicated in the pathophysiology of diarrhea in a number of diseases. Diarrhea could be the result of stimulation in chloride secretion or reduction in NaCl absorption or both. However, this chapter focuses mainly on the process of chloride absorption in the intestine. There are three major mechanisms for the absorption of chloride from the intestinal lumen, (i) passive Cl − absorption, (ii) HCO 3 − -dependent Cl − absorption, and (iii) active Cl − absorption. The paracellular or passive pathway is the major mechanism for chloride uptake in the jejunum and is dependent on transmural potential differences and on downhill concentration gradients. In the HCO 3 − -dependent Cl − absorptive pathway, Cl − is absorbed via a Cl − /HCO 3 − exchanger, which is not coupled to a parallel Na + /H + exchanger. However, active Cl − absorption is an electroneutral process and involves concerted functioning of Na + /H + and Cl − /HCO 3 − exchangers. This is the predominant route for Cl − absorption in the ileum and proximal colon with less prominence in the distal colon. Coupling of the Na + /H + and Cl − /HCO 3 − exchangers occurs via alterations in intracellular pH and is not associated with the generation of transepithelial current. In this model, the efflux of H + via Na + /H + exchanger makes the cytoplasm alkaline, which activates the Cl − /HCO 3 − exchanger functioning. The intracellular substrates, H + and HCO 3 − , for these transporters are produced by the action of carbonic anhydrase (CA). The net result is movement of Na + and Cl − into the cell in exchange for the exit of H + and HCO 3 − ( Fig. 57.1 ). This model is further supported by several studies demonstrating that colonic NaCl absorption is dependent on CA activity and is acutely sensitive to alterations in HCO 3 − and CO 2 concentrations. As both the transporters employ a stoichiometry of 1:1, there is no change in the distribution of charge across the membrane and thus the transport is called as electroneutral. Sodium at the basolateral membrane exits the enterocyte via the Na + /K + ATPase, while chloride may get out of the cell by either basolateral channels or exchangers ( Fig. 57.1 ). Na + /K + ATPase extrudes three sodium ions in exchange for two potassium ions with each cycle and generates the driving force for electroneutral NaCl absorption and all other transepithelial active transport processes.

57.1.1.1
Species Differences in Cl − /HCO 3 − Exchange in Mammalian Intestine
Cl − /HCO 3 − exchangers in the mammalian intestine play a pivotal role in transepithelial chloride absorption, bicarbonate secretion, and maintenance of intracellular pH and chloride concentrations. Significant regional, surface-crypt axis, and species differences exist with respect to the expression of these transporters and their regulation. Studies utilizing different complementary methods such as uptake measurements by purified apical plasma membrane vesicles (AMV) and intact tissue preparations in rabbit and rat duodenum and ileum have indicated the presence of Na + -independent Cl − /HCO 3 − exchange process. On the other hand, perfusion studies performed in normal humans and patients with congenital chloride diarrhea (CLD) demonstrated gross impairment of chloride absorption with absence of bicarbonate secretion in CLD patients indicative of the fact that the basic defect is an impaired Cl − /HCO − 3 exchange in the intestinal luminal membrane in the ileum and colon. Thus providing a compelling evidence for the presence of a Cl − /HCO 3 − exchange process as the major route for vectorial Cl − absorption in the human ileum and colon. CLD is a rare autosomal recessively inherited disorder characterized by defective intestinal Cl − absorption, diarrhea, and metabolic alkalosis. The diagnosis can be confirmed by measuring stool chloride concentration, which always exceeds 90 mmol/L in CLD patients (when corrected for water and electrolyte balance) compared to 10–15 mmol/L in normal individuals. Further, recent studies utilizing purified human small intestinal and colonic AMVs prepared from mucosal tissues harvested from organ donors have directly demonstrated the presence of an electroneutral Cl − /HCO − 3 exchange process as the major mechanism for Cl − uptake in human ileum, proximal, and distal colon.
In contrast, in the distal colonic region of rat, studies utilizing purified AMVs demonstrated the presence of two distinct exchangers involved in the process of electroneutral Cl − absorption along the crypt-to-surface cell axis (i) a Cl − /HCO 3 − exchanger predominantly in the surface coloncytes and (ii) a Cl − /OH − exchanger present mainly in colonic crypt area. Although, the physiological significance of the two distinct electroneutral anion exchangers for chloride transport in rat colon is not clear, it is suggested that Cl − /HCO 3 − exchange may be the predominant chloride absorbing transporter in surface cells, whereas Cl − /OH − exchange process may be important for other cellular functions in the crypts, for example, maintenance of cell volume and intracellular pH. There is evidence for the existence of only a single luminal Cl − /HCO 3 − /(OH) exchanger in the human colon. Recent studies have shown activity of Cl − /HCO 3 − exchange in colonic crypts isolated from human biopsy or surgical samples with higher activity in the surface vs. the middle and base of the crypts. The characteristics of human small intestinal and colonic Cl − /HCO 3 − exchange processes appear to be similar to those of their counterparts in other species such as rabbit ileum and rat jejunum. Furthermore, the presence of two distinct chloride transporters (Cl − /HCO 3 − and Cl − /OH − exchanger) does not appear to be a generalized phenomenon.
57.1.2
Molecular Identity of Cl − /HCO 3 − Exchangers
Human intestinal Cl − /HCO 3 − exchange process across the cell plasma membrane is mediated by multiple chloride and bicarbonate transporting anion exchangers, which are the members of solute-linked carrier (SLC) gene families SLC4 and SLC26 . The SLC4 family is also referred to as the bicarbonate-transporter super family and includes 11 genes (SLC4A1–11; where A6 is a pseudogene). Members of this family are mainly important for basolateral Cl − transport ( Section 57.1.4 ). The SLC26 family or the “sulfate permease” anion transport family consists of multifunctional anion exchangers capable of transporting monovalent and divalent anions with variable specificity as discussed in the following section.
57.1.2.1
SLC26 Gene Family
SLC26 family consists of highly versatile anion transporters that are conserved in bacteria (prokaryotic SulP/SLC26 transporters), fungi, yeast, plants (Sultr/SLC26 transporters), and animals, including human (SLC26 transporters). SLC26 family is genetically distinct from SLC4 family and encodes 11 genes, SLC26A1-A11, with SLC26A10 likely being a pseudogene. These genes exhibit a tissue-specific expression pattern with a robust expression found in organs such as stomach, intestine, pancreas, kidney, lung, and inner ear. A wide range of anions are transported by SLC26 family members with variable specificities including halides (Cl − , I − , Br − ), monovalent anions (OH − , NO 3 − , HCO 3 − , formate and glyoxylate), divalent anions (SO 4 2 − , oxalate), and thiocyanate (SCN − ). Members of this family usually operate as anion exchangers. However, some SLC26 isoforms are capable of functioning as chloride channels (SLC26-A7, A9, and A11).
57.1.2.1.1
Quintessential Features of SLC26 Protein Structure
SLC26 transporters are large proteins comprised of 606–970 amino acid residues. These proteins are predicted to contain between 10 and 14 transmembrane α-helices and a C-terminal domain. The SLC26 exchangers show considerable homology within the hydrophobic transmembrane domains (TMD). The -NH2 terminal region contains the 22-amino acid “sulfate transport” consensus signature (prosite, PS01130) comprising of several conserved residues critical for anion transport. The TMDs of SLC26 exchangers are followed by a linking region that connects to a carboxy-terminal sulfate transporter anti sigma factor antagonist (STAS) domain, extending into the cytoplasm. The STAS domain shares significant homology with bacterial antisigma factor antagonists, such as, SpoIIAA of Bacillus subtilis. Mammalian STAS domain also contains a short stretch of amino acids referred to as the “intervening sequence” (IVS) present between the α1 and β3-helices. IVS is absent in the SpoIIAA of B. subtilis and its function in the mammalian STAS domain is not yet elucidated. Mutations in the STAS domain result in defective functional expression of the protein largely due to improper trafficking of the protein to the membrane and retention of the protein in the endoplasmic reticulum. Mutations in the STAS domain of three members of SLC26 family, namely, SLC26A3, A4, and A2 have been associated with diseases, such as congenital chloride diarrhea (voluminous diarrhea), pendred syndrome (most common form of syndromic deafness), and diastrophic dysplasia (chondrodysplasias that cause skeletal defects), respectively. This is indicative of the fact that STAS domain plays a crucial role in the regulation of functional properties of these transport proteins. Furthermore, STAS domain also mediates protein-protein interactions. For example, STAS domain from Escherichia coli YchM (SLC26-SulP family) has been shown to form a complex with acyl-carrier protein (ACP), an essential component of the fatty acid biosynthesis pathway. Also, human SLC26A3 interacts with CFTR R-domain via the STAS domain. Besides the STAS domain, most of the SLC26 proteins also possess a class I PDZ interaction motif at the C-terminal end. The PDZ motif is not essential for the transport activity. However, the presence of these PDZ-binding motifs permits interaction of SLC26 family members with the NHERF family of PDZ adaptor proteins resulting in anchoring together various other transporters forming a multiprotein network at the plasma membrane. Formation of such multiprotein assemblies is an important step for different transport proteins to work together in an integrated manner during vectorial ion transport in the intestine (detailed in Section 57.1.2.2.2.2 ).
Among the SLC26 gene family members, two candidates SLC26A3 and SLC26A6 are of utmost importance in mediating apical anion exchange in the intestinal epithelial cells and will be discussed in the following sections.
57.1.2.2
SLC26A3 (DRA, CLD)
DRA was originally cloned as a candidate tumor suppressor gene that was downregulated in colonic adenoma and adenocarcinoma (hence, the name DRA). Subsequently, positional cloning studies identified DRA as the gene mutated in congenital chloride diarrhea (and its name changed to CLD), making it a candidate gene involved in intestinal Cl − absorption. According to HUGO Gene Nomenclature Committee, the name SLC26A3 is recommended instead of DRA or CLD to match the functional classification for the anion exchanger gene family. SLC26A3 gene consists of 21 exons and is localized to chromosome 7q22-q31.1. DRA cDNA encodes a protein of 764 amino acids with a predicted molecular mass of 85 kDa. SLC26A3 protein bears 44% identity and 60% similarity to SLC26A4. Also, the amino acid sequence of SLC26A3 protein exhibited high homology to the other SLC26 sulfate transporters: SLC26A1 and A2. A direct evidence supporting the role of SLC26A3 as the major Cl − /HCO 3 − exchanger was confirmed from brush-border vesicle studies performed in SLC26A3 KO mice. SLC26A3-null mouse displayed a CLD phenotype with sharp decrease in apical membrane chloride/base exchange activity concomitant with acidic luminal content in the colon, high chloride content diarrhea, volume depletion, and growth retardation.
57.1.2.2.1
Membrane Topology, Expression Profile, and N-Glycosylation Sites
Several membrane topology models have been proposed for SLC26A3 protein utilizing theoretical structure prediction algorithms. The protein product of SLC26A3 gene is predicted to contain 10–12 trans-membrane domains (TMD) that are typical for an integral membrane transporter. The NH 2 terminal is 500 amino acids long, and the COOH terminal domain contains about 250 amino acids and both of these termini are present in the cytoplasm. As mentioned previously ( Section 57.1.2.1.1 ), the C-terminal region of SLC26A3 harbors a PDZ domain and the STAS domain that are crucial for protein-protein interactions ( Fig. 57.2 ). A short stretch of four amino acids present at the C-terminal of SLC26A3, glutamic acid-threonine-lysine-phenylalanine (ETKF) forms a PDZ interaction motif. This motif facilitates the functional coupling of SLC26A3 with the apical Na + /H + exchanger isoform, NHE3. Moreover, PDZ motif is essential in retaining SLC26A3 in the constitutive endocytic-recycling pathway. As studies in heterologous expression system showed that absence of this motif in DRA-ETKF minus mutant resulted in significant reduction in the half-life of the transporter and facilitated its association with late endosomes, destined for lysosomal degradation. However, PDZ motif is not essential for transport activity, as DRA-ETKF minus mutant (which lacks PDZ motif) has been shown to be functionally active. The STAS domain of SLC26A3 is expected to begin after amino acid 500 and expands till amino acid 741. However, the exact structural details of the STAS domain for the mammalian SLC26 polypeptides are unavailable due to difficulties in crystallization and NMR solution structure determination of the intact STAS domain. SLC26A3 is expressed in the small intestine and colon of all species and its protein product is localized to the apical membranes of differentiated mucosal columnar cells. The expression of SLC26A3 varies along the longitudinal axis of the intestine, that is, colon shows higher expression of SLC26A3 compared to distal ileum with jejunum exhibiting a modest expression. In the mouse intestine, the highest expression was found in the cecum followed by distal colon and proximal colon. However, studies utilizing human biopsy samples showed relatively similar levels of SLC26A3 mRNA in human proximal and distal colon. With respect to the vertical axis distribution, in situ hybridization studies showed expression of SLC26A3 in the upper crypt and surface epithelium of the colon, while ileum shows expression in the deep crypt as well. In murine duodenum, SLC26A3-mediated Cl − /HCO 3 − exchange has been shown to be concentrated in the lower villus region. Besides the region specific expression of SLC26A3, its expression also varies ontogenically. A low level of SLC26A3 mRNA is present at birth, with a marked increase (134-fold) observed in its colonic expression during postnatal development in mouse. In the small intestine, however, SLC26A3 mRNA remains at a low constant level throughout the postnatal development. Similarly, SLC26A3 expression is present in the well-differentiated postconfluent Caco-2 cells but not in preconfluent cells. The expression of human SLC26A3 (CLD) has also been detected in extra intestinal tissues such as pancreas, eccrine sweat glands, and seminal vesicles.

SLC26A3 gene product is a glycoprotein. Protein sizes of the glycosylated and deglycosylated SLC26A3 vary among different species. Both rabbit and human SLC26A3 are glycosylated with protein sizes detected at 116, 108, and 97 kDa in rabbit intestine and 116 kDa in human. A number of other studies have reported sizes of SLC26A3 as 85 kDa in mouse and 70 kDa in Caco-2 human intestinal epithelial cells, where the 70 kDa band in Caco-2 cells is likely to be a deglycosylated form. This discrepancy between the glycosylated and deglycosylated forms of SLC26A3 protein may be attributed to the differences in number of glycosylation sites. N-glycosylation involves the addition of oligosaccharides to asparagine within the sequence motif Asn-X-Ser/Thr (-NXS/T-) of the membrane domains of the various SLC26 family members. Human SLC26A3 harbors five putative N-glycosylation sites (N153, N161, N164, N165, and N621) located between TMD 3 and 4, present on the second extracellular loop. Site-directed mutagenesis and biochemical modification studies performed in HEK (Human embryonic kidney cells) and CHO (Chinese hamster ovary) cells transfected with human-SLC26A3 revealed that out of these five sites, N153, N161, N164, and N165 are utilized for N-glycosylation of SLC26A3 ( Fig. 57.2 ). This phenomenon of N-glycosylation does not contribute toward anion selectivity and Cl − /HCO 3 − exchange activity of DRA. N-glycosylation does appear to play a role in transport of SLC26A3 from the ER to the Golgi complex ; however, it is not an obligatory requirement for trafficking of DRA to the cell surface. As glycosylation is known to prevent the cellular protease-mediated degradation of the plasma proteins, it should be noted that N-glycosylated SLC26A3 is more resistant to tryptic proteolysis. This suggests that glycosylation is essential for the protection of the DRA protein from digestion by luminal proteases under physiological conditions thus facilitating its stabilization at the plasma membrane.
57.1.2.2.2
Features of SLC26A3 Transport Function
Several studies performed in the last decade have provided strong evidence that SLC26A3 is the major anion exchanger involved in electroneutral NaCl absorption in the intestine, where it acts in a synchronized and interdependent manner with NHE3 ( Fig. 57.3 ). In addition to this bidirectional Cl − /HCO 3 − exchange, SLC26A3 also participates in HCO 3 − secretion, a process requiring functional CFTR ( Fig. 57.3 ). These two transport modes of SLC26A3 function are discussed in detail in the following sections:

57.1.2.2.2.1
Electroneutral NaCl Absorption: Collaborative Interplay of SLC26A3, NHE3, and Accessory Proteins
Since, the expression and characteristics of Na + and Cl − transporters vary remarkably along the length of human intestine, a functional coupling to mediate NaCl absorption requires that NHE3 be coexpressed with SLC26A3 along the apical surface of the intestinal epithelial cells. Interestingly, similar to SLC26A3, NHE3 expression also exhibits regional variations, where it is predominantly expressed on the apical membrane of surface epithelial cells. Recent studies utilizing quantitative immunofluorescence microscopy in consecutive colon segments of rats and mice proposed a topographical model pertaining to spatial distribution of NHE3 and SLC26A3 along the colonic axis. In cecum, proximal colon, and distal colon, NHE3 and SLC26A3 exhibited reciprocal expression profiles ( Fig. 57.4 ). Unpaired NHE3 expression in proximal colon favored H + secretion with acidic mucosal surface pH (pHms) of 6.2, whereas unpaired SLC26A3 activity in the cecum and distal colon resulted in alkaline mucosal surface pH (~ 7.5, pHms) consistent with net HCO 3 − secretion ( Fig. 57.4 ). Based on this model, NHE3 and SLC26A3 coexist only in the mid colon resulting in neutral pHms, favoring coupled electroneutral NaCl absorption. However, direct studies systematically evaluating the function and expression of both NHE3 and SLC26A3 across the length of the human intestine are not available. Additionally, in vivo studies utilizing transgenic mice also suggested coupling of SLC26A3 with NHE3. Direct coupling of SLC26A3 with NHE3 was also demonstrated in Caco-2.BBe cells infected with tetracycline-off-inducible SLC26A3 transgene. The heterologous expression of SLC26A3 in Caco2.BBe cells increased Cl − uptake that was dependent on both CA and apical NHE activity, suggesting that apical NHE3 and SLC26A3 when coexpressed mediate coupled electorneutral NaCl absorption in the intestine. Further, pharmacological inhibition of NHE3 with EIPA or of SLC26A3 with niflumic acid did not alter pHi, but coordinately inhibited both the exchangers in mouse jejunal epithelium, indicating functional coupling. Hence, both in vitro and in vivo studies point to coupling of the NHE3 and SLC26A3 exchangers, presumably via changes in intracellular pH. In fact, SLC26A3 has been suggested to possess a pHi sensor in the c-terminal domain. Such a pH i sensor is expected to sense sufficiency of intracellular HCO 3 − concentration and to inhibit SLC26A3-mediated Cl − /HCO 3 − exchange before pH i is acidified as seen in Xenopus oocytes where intracellular acidification inhibited hSLC26A3-mediated Cl − transport. In parallel, SLC26A3 is stimulated by alkaline pHi. Thus, each component of the NHE3-DRA dyad is allosterically stimulated by the transport activity of the other. Where intracellular acidification in response to Cl − /HCO 3 − exchanger by DRA favors stimulation of NHE3 and alkalinizing effect of NHE3 contributes to activate DRA, emphasizing their cooperative mode of operation.

Further, a model was proposed where a direct structural link between SLC26A3 and NHE3 was established via the PDZ adapter protein E3KARP (NHERF2) through its dimerization. In this model, SLC26A3 protein was shown to interact specifically with the second PDZ domain of E3KARP, through the PDZ interaction motif located in C-terminal region of DRA. As NHE3 also binds to the second PDZ domain of E3KARP, such an interaction enables DRA to be in close proximity with NHE3 attesting the functional coupling of the two transporters ( Fig. 57.3 ). Also, immunofluorescence studies showed DRA, NHE3, and E3KARP to be colocalized in the apical compartment of the human proximal colon indicative of the physiological relevance of this multimolecular assembly. Recent studies have also shown the interaction of SLC26A3 with other members of NHE3 regulatory factor (NHERF) family of PDZ adaptor proteins such as PDZK1 (NHERF3), E3KARP (NHERF2), and IKEPP (NHERF4) in the specialized compartments of plasma membrane known as lipid rafts. Lipid rafts (LR) are dynamic sphingolipid- and cholesterol-rich membrane micro-domains, which are resistant to detergent solubilization. Both NHE3 and SLC26A3 are lipid-raft-associated proteins ; however, whether their functional coupling depends on integrity of intact lipid rafts is not yet entirely understood.
57.1.2.2.2.2
Coupling of SLC26A3 with CA and Interactions with Accessory Proteins
DRA absorbs Cl − and exports HCO 3 − with equal affinity in a Carbonic Anhydrase II (CAII)-dependent fashion, demonstrating that its activity is regulated by intracellular and extracellular ion composition. CA drives the generation of H + and HCO 3 − ions from hydration of CO 2 and plays a crucial role in coupling of DRA and NHE3 for mediating electroneutral NaCl absorption. CA enzymes are abundantly expressed in the intestine and are present in both the apical membrane (CAIV) and the cytoplasm (CAI and CAII) of enterocytes. The administration of CA inhibitors reduces colonic chloride absorption indicating a functional relationship of CAs and Cl − /HCO 3 − exchangers in intestinal ion homeostasis. The physical and functional complex of a bicarbonate transporter with CA is referred to as “bicarbonate transport metabolon,” which facilitates coupled CO 2 /HCO 3 − metabolism and HCO 3 − transport. Indeed, SLC26A3-mediated bicarbonate transport is functionally coupled to the activity of the cytosolic carbonic anhydrase CAII. Also, inhibition of CAII activity by acetazolamide reduced SLC26A3 activity in HEK-293 cells (transiently transfected with SLC26A3 cDNA). However, in contrast to other bicarbonate transporters, SLC26A3 lacks a consensus CAII-binding motif in its C-terminal and does not physically interact with CAII. It was proposed that in the physiological context, an accessory protein binds CAII and localizes the enzyme in the proximity of DRA for optimal function ( Fig. 57.3 ).
57.1.2.2.2.3
Role of SLC26A3 in HCO 3 − Secretion-Coupling With CFTR and Preservation of Epithelial Barrier Integrity
Bicarbonate secretion is essential for the maintenance of pH and formation of alkaline mucus barrier against gastric acid in the luminal membrane of the intestine. As mentioned above, the coexistence of SLC26A3 and NHE3 points to a functional coupling between NHE3 and SLC26A3 to drive electroneutral NaCl absorption both in the ileum and colon. However, SLC26A3 is suggested to exhibit multiple transport modes based on its biological environment. In duodenum and pancreas, SLC26A3 activity has been shown to favor duodenal electroneutral HCO 3 − secretion compared to NaCl absorption. Functional studies utilizing KO mice supported the role of SLC26A3 in both basal HCO 3 − secretion and cAMP-stimulated HCO 3 − secretion in duodenum. Therefore, dudodenal bicarbonate secretion is in part electrogenic mediated by CFTR and partly electroneutral due to secretory activity of SLC26A3. The predominant expression of SLC26A3 in the crypt region in duodenum further indicates its role in duodenal HCO 3 − secretion. The secretory activity of SLC26A3 might be of relevance in pathophysiology of duodenal disorders including ulcer disease and cystic fibrosis (CF). Cystic fibrosis (CF) is an autosomal recessive disease characterized by aberrant HCO 3 − transport and results from mutational inactivation of CFTR, the cAMP-sensitive Cl − channel. In this regard, studies utilizing knockout mice demonstrated that a functionally active CFTR is necessary for SLC26 (SLC26A3 or SLC26A6)-mediated HCO 3 − secretion but not for SLC26-mediated fluid absorption. SLC26A3 interacts directly and functionally with CFTR. Coupling of SLC26A3 with CFTR at the plasma membrane involves interaction between the STAS domain of SLC26A3 and regulatory (R) domain of CFTR resulting in simultaneous increase or decline of ion transport by both the proteins ( Fig. 57.3 ). The indirect association between the two proteins is facilitated by PDZ domain proteins such as E3KARP and CAP70.
Further, SLC26A3 deficiency was correlated to a significant reduction in the rate of HCO 3 − secretion in the chronically inflamed distal ileum and colon of TNF +/ΔARE mice both by in vitro (Ussing chamber) and in vivo perfusion studies. Also, genetic ablation of DRA resulted in severely reduced colonic HCO 3 − secretory rate and the absence of an adherent inner mucus layer in colon of the knockout mice. Normal mucus release requires the presence of HCO 3 − anion for efficient sequestration of mucin bound cations thereby allowing its expansion. The absence of protective colonic mucus layer is considered as a potential mechanism involved in the development of colonic inflammation in both murine colitis models and IBD patients. This was also evident from the increased susceptibility of SLC26A3 −/− mice to DSS-induced colitis in this study. Based on the above observations, it can be stated that SLC26A3 deficiency not only results in decreased absorptive efficiency of colon but may also lead to impaired barrier function and aggravated inflammation associated with IBD.
57.1.2.2.2.4
Anion Specificity and Inhibitor Sensitivity of Slc26a3
Although, the role of SLC26A3 in mediating apical anion transport in mammalian intestinal epithelial cells is supported from studies performed in Xenopus oocytes, cell lines, CLD patients, and knockout mice models; function of this transporter still remains controversial with respect to anion specificity and inhibition profile. For example, initial studies using microinjected Xenopus oocytes as an assay system showed that mouse SLC26A3 encoded for a Na + -independent transporter for both sulfate and oxalate. Human and mouse SLC26A3 proteins were shown to mediate Cl − /OH − and Cl − /HCO 3 − exchange processes when expressed in Xenopus oocytes and human embryonic kidney (HEK) 293 cell line, respectively. Another report showed hDRA to mediate Cl − /Cl − and Cl − /HCO 3 − exchange but not the transport of sulfate or OH − , when expressed in Xenopus oocytes. Additionally, hSLC26A3 stably transfected in HEK-293 cells was demonstrated to function as an electroneutral Cl − /HCO 3 − exchanger with low Cl − /OH − exchange and minimal SO 4 2 − /HCO 3 − exchange activity. Contrary to this report, a recent study established that in mouse cecum DRA also contributes to SO 4 2 − secretion in exchange for HCO 3 − in addition to its role as a primary Cl − /HCO 3 − exchanger. In another study, transepithelial Cl − and oxalate fluxes measured in short-circuited segments of ileum, cecum and distal colon from wild-type and DRA knockout mice revealed that DRA significantly contributed to net oxalate absorption and that its deficiency led to a significant reduction in urinary oxalate excretion. It appears that, in addition to its primary role as Cl − /HCO 3 − exchanger, DRA may also contribute to oxalate and sulfate transport.
In addition, there are significant conflicting reports in the literature with regard to the inhibition of SLC26A3-mediated transport by stilbene derivatives, such as DIDS. For example, SLC26A3-mediated sulfate transport in Xenopus oocytes and Sf9 cells as well as Cl − transport was highly sensitive to inhibition by DIDS. On the other hand, some studies suggest very low sensitivity of SLC26A3-mediated Cl − transport to DIDS, when expressed in Xenopus oocytes or HEK 293 cells. The differences in these studies with regard to DIDS sensitivity are not clearly understood yet, and will require more exhaustive analysis of the inhibition profile of this transporter. Potent inhibition of SLC26A3 by the antiinflammatory drugs, such as tenidap and niflumic acid, is also reported.
57.1.2.2.3
SLC26A3 Structure-Function Relationship Lessons from CLD
Recent advances utilizing heterologous mammalian expression systems have identified distinct mechanisms by which CLD-causing mutations in SLC26A3 can cause disease by a better understanding of its structure-function relationship and regulation. In this regard, over 55–60 different mutations spreading over exons 3–19 have been identified in CLD patients from different geographical areas. .The most common of them being the three founder mutations present among Finnish (V317del), Polish (I675676ins), and Arabic (G187X) populations. Notably, SLC26A3 mutations in CLD patients (found in all parts of the protein) cluster around three locations that are critical for SLC26A3 function. Majority of the mutations are associated with the coding sequence and are located either in the N terminal, COOH terminal, or the transmembrane domains. Presence of several of these mutations in the intracellular C-terminal region of CLD protein is suggestive of the fact that the C-terminal domain of SLC26A3 possesses an important regulatory role, vital for the function of SLC26A3 as a transporter or as a regulator for another transporter. Mutations positioned in the STAS domain of SLC26A3 have also been identified in CLD patients, which result in the loss of SLC26A3 at the plasma membrane, secondary to combined abnormalities in protein folding, polypeptide trafficking, and stability. SLC26A3-structure-function studies utilizing Xenopu s oocytes have also demonstrated that truncation of N-terminal cytoplasmic domain or C-terminal region (44 amino acids) do not affect SLC26A3 function; however, deletion of STAS domain leads to complete loss of Cl − transport. Of note, none of these mutations are reported in the PDZ motif.
SLC26A3 expression is downregulated in adenomas; however, direct studies of the role of SLC26A3 gene in carcinogenesis done in Finnish CLD families did not demonstrate a strong increase in the risk of intestinal cancer in CLD mutation carriers. Along the same lines, a role for C-terminal region of SLC26A3 as a growth suppressor was identified in cancer cell lines. The V137del mutation in SLC26A3 found in Finnish CLD patients resulted in a decrease in chloride and sulfate transport in Xenopus oocytes, but had no effect on growth suppression function of SLC26A3 C-terminus. Functional defects in SLC26A3 expressed in tissues other than intestine such as eccrine sweat glands and seminal vesicles have not been reported in CLD patients. Reduced fertility has occasionally been seen in adult Finnish male CLD patients. Additional studies are warranted to pinpoint the role of specific structural residues of SLC26A3 in its regulation as well as its role in growth suppression and tumorigenesis.
57.1.2.3
SLC26A6 (PAT1)
SLC26A6 also referred to as putative anion transporter-1 (PAT-1), or chloride/formate exchanger (CFEX), was identified exclusively through database mining based on homology to DRA (SLC26A3) and pendrin (SLC26A4). The SLC26A6 gene is composed of 21 exons and 21 introns, and spans 2217 bp of chromosome 3q21.3. cDNA of SLC26A6 encodes a protein of 738 amino acids with a predicted molecular mass of 84 kDa. The amino acid sequence of SLC26A6 protein exhibits 50% homology to SLC26A3 and SLC26A4, together with a large number of conserved residues. Human SLC26A6 gene was also found to have three alternatively spliced variants, named SLC26A6 a, c, and d. With regard to their tissue distribution, RT-PCR studies indicated that SLC26A6a but not c or d is the spliced variant expressed in the human small intestine and colon.
57.1.2.3.1
Membrane Topology, Expression Profile, and N-Glycosylation Sites
The protein product of SLC26A6 gene is predicted to contain 11 transmembrane helices and an intracellular NH2 and COOH terminus. Human PAT1 isoforms a, c, and d comprise of 12-, 8-, and 12-membrane spanning domains, respectively. Similar to other SLC26 family members, the C-terminal cytoplasmic tail of SLC26A6 isoform a and c harbors a PDZ interaction motif, which is identical to that of CFTR (TRL, Threonine-Arginine-Leucine). In vitro studies have shown that PAT1 a and c isoforms interact with the first and the second PDZ domains of NHE3 regulatory proteins NHERF1 and E3KARP via the TRL motif. The STAS domain of SLC26A6 encloses the CAII-binding site and facilitates the interaction of SLC26A6 with R domain of CFTR. SLC26A6 exhibits an inverse expression pattern as compared to that of SLC26A3 in the mouse gastrointestinal (GI) tract, that is, a higher level of expression in the small intestine compared to the colon. In the small intestine, SLC26A6 is abundantly expressed on the apical membrane of intestinal villi, extending from the duodenum to jejunum and ileum. Northern blot studies showed widespread pattern of expression of SLC26A6 transcript in tissues other than intestine with highest abundance in kidney and pancreas. Immunohistochemistry studies showed expression of SLC26A6 both in the apical and basolateral membranes of kidney tubules and in the brush border of pancreatic duct. PAT1 expression is also noted in liver, heart, skeletal muscle, stomach, esophagus, and placenta, with relatively lower expression in lungs and brain.
SLC26A6 has been identified as ~ 85–90 kDa band in brush border of the villus cells. The size of the SLC26A6 protein in the lysates of stably transfected MDCK cells varied from 84 kDa to > 200 kDa, suggestive of strong glycosylation of SLC26A6 in these cells. Potential N-glycosylation sites (-NXS/T-) in human SLC26A6 are located at the amino acid positions 167, 172, and 614. Studies utilizing site-directed mutagenesis in transfected HEK cells demonstrated that SLC26A6 utilizes N167 and N172 located in the extracellular loop 2 as the acceptor sites for N-glycosylation. Additionally, it was shown that N-glycosylation influences the efficacy of the trafficking process of SLC26A6 to the plasma membrane and is crucial for enhancing the stability of the transporter at the cell surface. A comparative study analyzing the glycosylation of endogenous SLC26A6 in human Caco-2.BBe cells and mouse duodenum, jejunum, and kidney revealed that degree of glycosylation of SLC26A6 is highly tissue specific and also appeared to be important for optimal activity.
57.1.2.3.2
Functional Role of SLC26A6
SLC26A6a and its spliced variants SLC26A6c and d were shown to function as anion exchangers mediating DIDS-sensitive Cl − and SO 4 2 − transport. With respect to anion specificity, SLC26A6 has been shown to function in Cl − /oxalate, Cl − /HCO 3 − , SO 4 2 − /oxalate, SO 4 2 /Cl − , Cl − /formate, and Cl − /OH − exchange modes. Several in vitro and knockout mouse model studies suggest that dominant modes of transport by SLC26A6 include Cl − /HCO 3 − exchange (predominantly detected in the proximal portion of the small intestine) and Cl − /oxalate exchange (predominantly detected in the kidney proximal tubule and ileum). These two modes are described in detail in the following sections:
57.1.2.3.2.1
Role in Cl − Absorption and Bicarbonate Secretion
SLC26A3 and A6 are the candidate genes mediating apical Cl − /HCO 3 − exchange. In this regard, it has been shown that both mouse and human SLC26A6 function as Cl − /HCO 3 − exchanger, when expressed in Xenopus oocytes. Also, a decrease in net Cl − and Na + fluxes observed in jejunum of SLC26A6 KO mice further attested that SLC26A6 couples with NHE3 in mediating electroneutral absorption in the small intestine. Therefore, NHE3 couples with both SLC26A3 and SLC26A6 to mediate electroneutral NaCl absorption ( Fig. 57.3 ). However, function and expression studies suggest that these two exchangers do not exhibit parallel activities but rather show predominance over one another in different regions of the intestine. For example, measurement of apical Cl − /HCO 3 − exchange showed dominance of SLC26A6 activity over SLC26A3 activity in the duodenal upper villus epithelium, whereas; dominant activity of SLC26A3 was observed in mouse jejunal villus epithelium with little contribution by SLC26A6. Also, in proximal jejunum with high CO 2 tension and HCO 3 − concentration, SLC26A6 is involved in Na + HCO 3 − absorption and acid/base balance, and SLC26A3 mainly mediates the absorption of Cl − . Therefore, both SLC26A3 and A6 have distinct roles in basal and CO 2 /HCO 3 − -stimulated fluid and electrolyte absorption in mouse jejunum, thereby ensuring the absorption of Cl − and HCO 3 − under varying luminal concentrations of these anions. This further highlights the physiological relevance for the presence of two anion transporters with similar ion exchange activity in the same cellular location. These studies together with the mild expression of SLC26A3 in the mouse small intestine compared to the colon, suggest that SLC26A6 is important for the apical Cl − base exchange in the upper GI tract and SLC26A3 in the lower GI tract. Intriguingly, unlike diarrheal phenotype of SLC26A3-null mice, mice with deletion of SLC26A6 appeared normal at steady state. This may be due to compensatory upregulation of other anion exchangers in knockout mice. Of note, the mRNA expression of DRA remained unaltered in the small intestine of SLC26A6 knockout mice. In addition, it is suggested that the loss of SLC26A6 may lead to a significant phenotype in pathophysiological states. Thus, it appears that although SLC26A6 is involved in mediating Cl − absorption, it is not directly coupled to water movement as seen with SLC26A3.
Besides Cl − absorption, SLC26A6 also mediates bicarbonate secretion. As mentioned before, duodenal bicarbonate secretion (DBS) plays an important role in preventing mucosal injury by the acidic chyme secreted from the stomach. Both SLC26A3 and A6 are involved in DBS. Role of SLC26A6 in DBS was further confirmed with reduced baseline bicarbonate secretion and apical Cl − /HCO 3 − exchange activity in the duodenum of SLC26A6-null mice. However, it should be noted that a significant component of HCO 3 − secretion was still intact in knockout mice suggesting the involvement of other anion exchangers such as SLC26A3. Unlike SLC26A3, SLC26A6 has been shown to directly bind CAII via the binding site located within the STAS domain ( Fig. 57.3 ). This coupling facilitates the SLC26A6-mediated transmembrane HCO 3 − flux by maximizing the HCO 3 − concentration at the SLC26A6 transport site. Functionally active CFTR is a prime requirement for SLC26-mediated HCO 3 − secretion. However, this does not hold true for SLC26-mediated Cl − absorption. Therefore, it can be concluded that both SLC26A3 and A6 work in conjunction with NHE3 in Cl − absorptive mode and require the exit of Cl − from the cell via CFTR to function in HCO 3 − secretory mode ( Fig. 57.3 ). Structural interaction of SLC26A6 and CFTR has been shown to result in reciprocal activation of both transport proteins in vitro.
57.1.2.3.2.2
Role in Oxalate Transport
SLC26A6 serves as a major oxalate-secreting pathway in the small intestine. Mice deficient in SLC26A6 exhibit enhanced oxalate absorption in the small intestine, with subsequent increase in urine oxalate excretion and kidney oxalate stone formation. Additionally, the role of SLC26A6 in oxalate transport was analyzed in Caco-2.BBe monolayers utilizing siRNA knockdown technology. These studies demonstrated that SLC26A6 contributed to about 50% of the apical oxalate exchange. Further, SLC26A6-mediated vectorial transport of oxalate was shown to be net secretory or absorptive (apical oxalate efflux or influx) depending on the magnitude and direction of transepithelial Cl − gradient. Renal tubule microperfusion studies and renal brush-border vesicles isolated from SLC26A6-null mice demonstrated that Slc26a6 contributes to all oxalate-dependent NaCl transport and a component of formate-dependent NaCl transport. It is interesting to note that mice and humans show differences in susceptibility to nephrolithiasis with mice being highly resistant to nephrolithiasis, whereas humans show significant susceptibility. A recent study demonstrated that the human SLC26A6 and mouse Slc26a6 have divergent anion transport properties that might underlie these variations in susceptibility to oxalate kidney stones. Studies utilizing oocytes injected with the mouse or human RNA demonstrated that mouse Slc26a6 has more affinity for extracellular chloride over the human SLC26A6 in exhibiting oxalate efflux in exchange for chloride influx. The oxalate influx pathways in the intestine and their relevance to kidney stone formation are described elsewhere ( Section 57.4 ).
57.1.2.4
Electrogenicity vs. Electroneutral Mode of SLC26A3 and A6
With respect to electroneutral vs. electrogenic modes, the SLC26A3 and A6-mediated exchange is controversial. For example, SLC26A6 and SLC26A3 were found to be electrogenic with opposite apparent stoichiometries. Xenopus oocytes expressing SLC26A6 hyperpolarized on removal of extracellular Cl − in the presence of HCO 3 − , whereas, marked depolarization was seen in SLC26A3-injected oocytes. These studies demonstrated that SLC26A3 mediates anion exchange process with a Cl − -HCO 3 − ratio of ≥ 2. SLC26A6 activity was found to be voltage regulated and occurred at a HCO 3 − -Cl − ratio ≥ 2. The expression of SLC26A3 and SLC26A6 working at opposite stoichiometries in the same cell, therefore, might result in an apparent electroneutral Cl − /HCO 3 − exchange process. However, this model may not fit in the GI tract because of differential expression of SLC26A3 and SLC26A6 in different regions of the intestine. In this regard, many other studies demonstrated that SLC26A3 may function as an electroneutral rather than electrogenic transporter. Species differences in electrogenic vs. electroneutral pathway have also been reported. For example, mouse oxalate/chloride exchange mode via SLC26A6 was demonstrated to be electrogenic, whereas the exchange mode via human SLC26A6 was suggested to be apparently electroneutral. More in-depth studies are needed in different regions of the intestine to resolve these discrepancies that may accurately fit an electroneutral model of NaCl absorption mediated via SLC26A3 or A6 coupled with NHE3.
57.1.3
Regulation of Intestinal Cl − /HCO 3 − Exchangers
Under normal physiological conditions, the movement of solutes, particularly sodium and chloride, across the intestinal epithelium sets up an osmotic gradient along which water is absorbed. An increase in electrolyte secretion or a decrease in the absorption of electrolytes, such as Na + and Cl − , leads to water retention in the intestinal lumen resulting in diarrhea. Mounting evidence now suggests that decreased electrolyte absorption plays a critical role in the pathophysiology of diarrhea.
Besides its role in CLD, recent studies have established a critical role of SLC26A3 in several diarrheal diseases including infectious and inflammatory diarrhea. A number of studies have now demonstrated that SLC26A3 undergoes extensive regulation by acute (posttranslational), long-term (transcriptional) and posttranscriptional mechanisms. However, the role of SLC26A6 and its relevance to diarrheal diseases in the intestine as well as its regulation is poorly defined. The mechanisms and regulation of intestinal chloride absorption in the normal physiology and its potential modulation under normal physiological conditions and their potential modulation in intestinal and colonic disorders are described below and are summarized in Table 57.1 :
Regulatory Factor | SLC26A3 | SLC26A6 |
---|---|---|
Bioactive agents and transcription factors | ||
NPY | Increase in Cl − /HCO 3 − exchange activity via enhanced association with lipid-raft fractions | NI |
LPA | Increase in surface levels via PI3-kinase/AKT pathway and expression (long-term) with the involvement of LPA2 receptor and PI3-kinase/AKT signaling pathways in a c-fos-dependent manner | No change in surface levels and expression |
S1P | Increase in expression (long-term) with the involvement of S1P-receptor 2 and PI3-kinase/AKT signaling pathways in a YY1 dependent manner | No change in expression |
ATRA | Increase in surface levels via PI3-kinase/AKT pathway and increased expression (long-term) with the involvement of RARβ and HNF1β | No change in expression |
Butyrate | Increase expression via YY1 and GATA transcription factors | NI |
HNFs | Regulation of basal promoter activity and expression | NI |
Dexamethasone | Increase in promoter activity and expression | No change in expression |
PKC | NI | Decrease in surface levels |
cAMP | Decrease in surface levels via clathrin pathway | Increase in activity |
Ca 2 + | Inhibition of activity via NHERF3 | NI |
Micro RNAs | Decrease in protein expression by miR494 | NI |
Gut microbes (probiotics and pathogens) | ||
Lactobacillus acidophilus | Increase in surface levels via PI3-kinase dependent pathway, increase in Cl − /HCO 3 − exchange activity via enhanced association with lipid-raft fractions via PI3-kinase/Rac-1 pathway (short-term) and increase in expression (long term) | No change in expression |
Bifidobacterium sp. | Increase in function and expression via ERK1/2 MAPK pathway | No change in expression |
Oxalobacter formigens | NI | Increase in activity |
Enteropathogenic E. coli | Decrease in surface levels via microtubule disruption | No change in surface levels |
Citrobacter rodentium | Decrease in expression | Decrease in expression |
Helicobacter | No change in expression | Decrease in expression |
Protein-protein interactions | ||
CFTR | Activation via PDZ interactions | Activation via PDZ interactions |
NHERF4 | Decrease in surface expression | NI |
Cytoskeletal elements | ||
Keratin 8 | Decrease in expression | No change in expression |
Myosin5b | Decrease in expression | NI |
Intestinal inflammation and proinflammatory cytokines | ||
IL-1β | Decrease in expression | NI |
IFNγ | Decrease in expression and promoter activity via JAK-1/STAT-1 | Decrease in expression and promoter activity via IRF-1 |
TNFα | Decrease in expression and promoter activity via NF-κB pathway | No change in expression |
Inflammation (DSS) | Decrease in expression | No change in expression |
57.1.3.1
Short-Term Regulation of Cl − /HCO 3 − Exchangers
Acute regulation of ion transporters occurs via phosphorylation/dephosphorylation, membrane trafficking events, lipid-raft mediated mechanisms or via accessory proteins that cause rapid regulation of the preexisting transporters in the intestinal epithelial cells without altering their total cellular expression. During the last decade, significant advances have been made in understanding the acute regulation of apical SLC26 exchangers especially SLC26A3 in the mammalian intestine that have enhanced our understanding of the pathophysiology of early diarrhea induced by enteric infections or diarrhea associated with inflammatory bowel diseases. These studies have further identified potential proabsorptive agents that could be used as effective antidiarrheals. The current knowledge pertaining to the acute regulation of apical Cl − /HCO 3 − exchangers in the mammalian intestine is summarized below:
57.1.3.1.1
Bioactive Agents
57.1.3.1.1.1
Hormones and Neuropeptides
Modulatory effects of hormones and neuropeptides on the electrolyte and water absorbing capacity of intestine in various species including human, rat, rabbit, and dog have been reported. For example, an increase in NaCl absorption has been shown to occur in response to catecholamines, glucocorticoids, thyroxine, and the peptide PYY, whereas serotonin was shown to inhibit electroneutral NaCl absorption. Serotonin (100 nM for 1 h) was demonstrated to inhibit apical Cl − /HCO 3 − exchange as well as Na + /H + exchange activity in Caco-2 cells. The results of this study indicated that the inhibition of DIDS-sensitive 36 Cl − uptake by serotonin was mediated via 5-HT3 and 5-HT4 receptors, activation of c-Src kinase and PKCδ-pathway ( Fig. 57.5 ). Whether serotonin inhibits apical Cl − /HCO 3 − exchange by modulating SLC26A3 or SLC26A6 has not yet been delineated.

In contrast to inhibitory effects of serotonin, both in vitro and in vivo studies have shown that proabsorptive hormone NPY increases intestinal Cl − /HCO 3 − exchange activity via ERK1/2 pathway. This stimulation in response to NPY was attributed to increased association of SLC26A3 with lipid-raft fractions with no alteration in its total plasma membrane levels. With respect to the effect of prostaglandins on SLC26A3 or SLC26A6, a recent study demonstrated that Lubiprostone, a prostaglandin E1 derivative increased the apical expression of SLC26A6 in rat jejunum, ileum, and proximal colon, whereas apical SLC26A3 expression was downregulated in these tissues. A significant reduction in the surface levels of NHE3 in rat ileum and colon was also demonstrated in this study. These data indicated that Lubiprostone, suppressed fluid absorption and, therefore, was beneficial for the treatment of chronic constipation.
A number of other molecules, for example, neurotensin, vasoactive intestinal peptide, acetylcholine, guanylin, histamine, bradykinin, leukotrienes, adenosine, and platelet-activating factor have also been shown to be pro-secretory and antiabsorptive in nature. However, direct studies evaluating the effects of these molecules on Cl − /HCO 3 − exchange activity or SLC26A3 and SLC26A6 expression in the mammalian intestine are warranted.
57.1.3.1.1.2
Nitric Oxide and Reactive Oxygen Metabolites (ROMs)
Nitric oxide (NO) is a free radical that plays an important role in regulating several physiological functions of the GI tract, for example, regulation of motility, mucosal permeability, and intestinal ion transport. However, excessive production of nitric oxide and reactive oxygen metabolites including hydrogen peroxide has been implicated in the pathophysiology of inflammatory bowel disorders. With respect to effects on ion transport, NO is shown to exhibit differential effects under normal physiological conditions vs. pathophysiological conditions. NO has been suggested to be a proabsorptive molecule, whereas under pathophysiological conditions it has been shown to stimulate net secretion and may underlie diarrhea associated with IBD. Inhibition of constitutive nitric oxide (cNO) was shown to stimulate crypt cell Cl − /HCO 3 − exchange activity with no effect on the villus cell Cl − /HCO 3 − exchange activity. In contrast, high concentrations of NO were shown to inhibit the apical membrane Cl − /HCO 3 − (OH − ) exchange activity in Caco-2 cells via both cGMP/PKG and PKC signaling pathways ( Fig. 57.5 ). In parallel studies, apical NHE3 activity was also shown to be inhibited by NO in Caco-2 cells by cGMP but PKC-independent pathways. Whether SLC26A3 or A6 is inhibited by NO has not been investigated and would be of interest. Similar to NO, H 2 O 2 was found to acutely inhibit the Cl − /HCO 3 − exchange activity in Caco-2 cells via the involvement of c-Src family kinases including fyn and p60Src, PI3K, and PKCα-mediated pathways. The decrease in Cl − /HCO 3 − exchange activity was independent of alterations in the surface levels of both SLC26A3 and A6. Whether SLC26A3 or A6 are modulated by phosphorylation or lipid rafts mechanism(s) in response to ROM is not yet known.
57.1.3.1.1.3
Bile Acids
Bile acids have been shown to induce diarrhea in various pathological conditions such as inflammatory bowel disease, ileal resection, radiation enteritis, celiac disease, small intestinal bacterial overgrowth (SIBO), chronic pancreatitis, and idiopathic bile acid malabsorption (BAM). Mechanisms underlying diarrhea induced by bile acids are not fully understood and appear to involve a decrease in NaCl absorption with a concomitant increase in Cl − secretion in the intestine. For example, in ex vivo studies with short-circuited rabbit colon, addition of taurochenodeoxycholic acid (TCDC) from the mucosal side resulted in 50% decrease in net sodium flux and almost complete loss of net chloride flux. In contrast, addition of TCDC to the serosal compartment abolished net sodium flux and stimulated electrogenic Cl − secretion. Further, TDC (taurodeoxycholic acid) and GCDC (glycochenodeoxycholic acid) were shown to reduce the apical Cl − /HCO 3 − exchange in Caco-2 cells via multiple signal transduction molecules, including cAMP, Ca 2 + , PI3K, and PKC-β1-dependent pathway ( Fig. 57.5 ). Perfusion studies performed in human colon showed induction of water and electrolyte secretion by dihydroxy bile acids. Also, a decrease in Na + /H + exchange (as a result of inhibition of NHE1, 2 and 3) with a parallel decrease in Cl − /HCO 3 − exchange activity has been recently demonstrated in colonic crypts from patients having ileum resection/cholecystectomy-associated diarrhea. In this study, impaired ATP production and elevated intracellular Ca 2 + concentration were shown to inhibit NHE activity and Cl − /HCO 3 − exchange in response to acute treatment of chenodeoxycholic acid to colonic crypts isolated from the control subjects. Therefore, strategies to upregulate NaCl absorption offer new avenues for the treatment of diarrhea associated with BAM.
57.1.3.1.1.4
Protein Kinase C
A number of signaling pathways involving different members of PKC subfamily are implicated in inhibition of Cl − /HCO 3 − exchange by various stimuli. For example, phorbol 12-myristate 13-acetate (PMA), an in vitro PKC agonist was shown to inhibit directly the apical membrane Cl − /HCO 3 − exchange activity via the activation of PI3-kinase and its downstream effector, PKCε. Also, Cl − /HCO 3 − exchange is inhibited by PKCδ in response to serotonin as well as by PKCβ1 and PKCα in response to bile acids and H 2 O 2 , respectively ( Fig. 57.5 ). Thus, it is becoming apparent that the apical Cl − /HCO 3 − exchangers are directly modulated by protein kinases by complex mechanisms but their direct effects on SLC26A3 in human intestinal epithelial cells (IECs) have not yet been investigated. In this regard, PMA activation had no effect on SLC26A3 function in HEK293 cells. Direct phosphorylation/dephosphorylation of SLC26A3 by protein kinases in intestinal epithelial cells will be of relevance in future studies. Interestingly, mutation of tyrosine residue Y756F has been shown to increase the basal activity of SLC26A3, suggesting a possible role for tyrosine phosphorylation in inhibition of hDRA activity, although signaling pathways have not yet been identified.
Very few studies are available with respect to SLC26A6 regulation. SLC26A6 has been shown to be negatively regulated by adrenergic stimulation and angiotensin II, via PKC. The mechanism of this inhibition by PKC involved displacement of binding of CAII to A6 and consequent disruption of the HCO 3 − transport metabolon. Another study demonstrated that PKC activation by PMA inhibits different modes of SlC26A6-mediated transport including Cl − /formate exchange, Cl − /oxalate exchange, and Cl − /Cl − exchange in Xenopus oocytes via PKC. These studies also demonstrated redistribution of SLC26A6 from plasma membrane to intracellular compartments by PKC. The observation that PKC activation by PMA inhibits Cl − /oxalate exchange via PKCδ was reconfirmed by another recent study in T-84 cells. These results also validated the use of T-84 cells as a model to study the regulation of SLC26A6 under various stimuli. This study also demonstrated that cholinergic stimulation with carbachol inhibited SLC26A6-mediated Cl − /oxalate exchange, via the muscaranic acid receptor-3 (M 3 ), via the involvement of PLC, PKCδ and c-Src ( Fig. 57.6 ). Furthermore, it was shown that carbachol treatment resulted in decreasing the cell surface levels of SLC26A6, whereas total SLC26A6 levels remained unaltered indicating modulation of trafficking of SLC26A6 to the cell surface. In addition, inhibition of Cl − /oxalate exchange in response to ATP and UTP treatment in Caco-2.BBe cells mediated via purinergic receptor P2Y 2 was also demonstrated. Both ATP and UTP decreased oxalate transport by reducing surface expression of SLC26A6 through activation of Phospholipase C (PLC) and PKCδ ( Fig. 57.6 ). More studies in the intestinal epithelial cells are needed to examine the detailed membrane trafficking events modulating SLC26A6 and their relevance in oxalate homeostasis, HCO 3 − secretion as well as NaCl absorption.

57.1.3.1.1.5
cAMP and Ca 2 +
Similar to NHE3, cAMP-mediated regulation of SLC26A3 appears to involve interaction with the PDZ adapter proteins such as NHERF2 and NHERF3. SLC26A3 is either activated or inhibited by cAMP based on its mode of transport and cofunctioning proteins such as NHE3 or CFTR. For example, SLC26A3 is activated by cAMP, when it is expressed in a complex with CFTR and NHERF3. In contrast, SLC26A3 is inhibited by cAMP in parallel with NHE3 inhibition during NaCl absorption. Since, both NHE3 and SLC26A3 bind to second PDZ domain of NHERF2, it is possible that SLC26A3 inhibition occurs secondary to NHE3. This inhibition may occur via functional coupling by the intracellular pH or may involve endocytosis pathways. In this regard, recent studies utilizing Caco2.BBe monolayers demonstrated that SLC26A3 undergoes endocytosis in response to both cAMP or Ca 2 + . Moreover, detailed trafficking mechanisms modulating SLC26A3 will require in-depth studies utilizing NHE3 and NHERF knockout mice. With respect to SLC26A6, studies have shown that cAMP stimulates Cl − /base exchange activity in Xenopus oocytes and HEK-293 cells, coexpressing CFTR with SLC26A6. Ca 2 + -mediated inhibition of SLC26A3, however, was shown to be independent of regulation of CFTR and NHE3 and required interaction of SLC26A3 with NHERF3. Similar studies on regulation of SLC26A6 in intestinal epithelial cells are lacking.
57.1.3.1.1.6
LPA
Lysophosphatidic acid (LPA), a potent bioactive phospholipid has been implicated as a proabsorptive and potential therapeutic agent in diarrheal conditions. LPA has been shown to stimulate NHE3 function and inhibit CFTR dependent Cl − secretion in the intestine. Recent studies demonstrated that stimulation of SLC26A3 might also underlie antidiarrheal effects of LPA. This study showed that LPA via LPA2 receptor and activation of PI3Kinase, upregulated the Cl − /HCO 3 − exchange activity with a parallel increase in the plasma membrane levels of SLC26A3. However, SLC26A6 levels on the plasma membrane remained unaltered. LPA-induced increase in NHE3 and SLC26A3 function (via distinct mechanisms) may underlie the potential proabsorptive effects of LPA.
57.1.3.1.1.7
All Trans-Retinoic Acid (ATRA)
ATRA, a major active metabolite of vitamin A, is shown to exert antiinflammatory and antidiarrheal effects. Majority of these effects of ATRA are receptor mediated where retinoic acid receptors (RARs-α, β and γ) and/or retinoid X receptors (RXRα, β and γ) dimerize and transactivate the target genes at the transcriptional level. In addition to the classical genomic effects, ATRA has recently been shown to exhibit rapid nongenomic effects via modulation of signal transduction pathways. In this regard, in vitro studies in Caco-2 cells have shown that ATRA exerts nongenomic effects on SLC26A3. This study demonstrated that ATRA acutely upregulates Cl − /HCO 3 − exchange activity and surface levels of SLC26A3 via RAR-β activation and PI3K dependent pathway. This nongenomic stimulatory effect of ATRA on SLC26A3 could be exploited in pathophysiological conditions where SLC26A3 function and trafficking are impaired.
57.1.3.1.2
Gut Microbes
57.1.3.1.2.1
Probiotics
Lactobacillus acidophilus : Probiotics have been used in clinical trials for the prevention and treatment of various forms of diarrhea such as acute infectious diarrhea, antibiotic-associated diarrhea, and diarrhea-predominant irritable bowel syndrome. Lactobacilli , one of the predominant commensal bacteria in the gut microflora, are most commonly used probiotics for the prevention and treatment of diarrheal disorders. In vitro studies have shown that treatment of Caco-2 cells with L. acidophilus (LA) as well as culture supernatants derived from live bacteria stimulated SLC26A3 by increasing its surface membrane levels via a PI3K-dependent pathway ( Fig. 57.7 ). As an extension to these observations, another study demonstrated that heat stable soluble factors of low molecular mass (3–10 kDa) present in LA-culture supernatant resulted in upregulation of SLC26A3 function and surface levels involving PDZ protein NHERF2, PI3 kinase, and Rac-1. Additionally, this stimulation in response to LA-CS was attributed to increased association of SLC26A3 with lipid-raft fraction at the plasma membrane. Further studies are needed to evaluate the effects of other probiotics such as Saccharomyces on both SLC26A3 function and expression in the human intestine.

Oxalobacter formigenes : This anaerobic bacterium inhabits the mammalian colon and utilizes dietary and endogenous oxalate as its sole energy source. The probiotic potential of this bacteria is associated with lowering the risk of developing calcium oxalate stones by degrading oxalate in the colon and hence reducing its excretion in the urine. A recent study identified that conditioned medium (CM) from O. formigenes significantly stimulated oxalate uptake in Caco2.BBe cells via a PKA-dependent pathway ( Fig. 57.6 ). The bioactive factors responsible for stimulating oxalate transport were demonstrated to be heat labile protein/peptide with a molecular mass of 10–30 kDa. This CM-induced upregulation of oxalate transport appeared to be due to an increase in the intrinsic activity of SLC26A6 transporter present on the membrane with no changes in the trafficking of the transporter or its gene expression. Thus, O. formigenes -derived bioactive factors hold therapeutic potential for prevention and/or treatment of nephrolithiasis.
57.1.3.1.2.2
Pathogens-Enteropathogenic E. coli
EPEC, a food-borne pathogen, is a major cause of infantile diarrhea worldwide that is associated with high rate of morbidity and mortality. Unlike prototype enteric pathogens such as Vibrio cholerae , EPEC does not produce toxins. Therefore, the pathophysiology of early diarrhea associated with EPEC infection remained elusive for many decades. However, it is now evident that coupled decrease in both SLC26A3 and NHE3 activities in response to EPEC infection appears to underlie the pathophysiology of EPEC-induced early diarrhea. EPEC infection results in a decrease in Cl − /HCO 3 − exchange and NHE3 via type 3 secretion system (T3SS) of EPEC (responsible for injection of bacterial virulence factors into the host cells). Studies utilizing different deletion mutants of EPEC-secreted proteins (Esp) showed that EPEC via its virulence factors espG1 and G2 (involved in microtubule network disruption) decreased the level of SLC26A3 but not SLC26A6 on the apical membrane of enterocytes. With respect to the trafficking events in response to EPEC infection, a recent study demonstrated that EPEC-mediated decrease in SLC26A3 function and surface expression in Caco-2 cells involved decreased exocytosis and increased endocytic recycling ( Fig. 57.7 ). This study also demonstrated that EPEC infection resulted in redistribution of SLC26A3 from lipid rafts to detergent soluble nonraft membrane fractions.
57.1.3.1.3
Protein-Protein Interactions
57.1.3.1.3.1
CFTR
Several lines of evidence suggest that SLC26 transporters may interact with CFTR. For example, CFTR was shown to upregulate SLC26A3 (DRA) and SLC26A6 (PAT1) function in cultured pancreatic duct cells and activation of SLC26A3 and A6 by CFTR was demonstrated in coexpression studies conducted in HEK-293 cells. CFTR-dependent activation of SLC26 transporters is an important mechanism in which CFTR can switch “on and off” the HCO 3 − secretion in CFTR expressing cells. In this regard, previous studies suggested that HCO 3 − secretion defect seen in CF patients might be partly due to the downregulation of apical Cl − /HCO 3 − exchange activity mediated by SLC26A3 or SLC26A6. For example, stimulation of CFTR by cAMP, resulted in activation of Cl − /HCO 3 − exchange activity in submandibular gland (SMG) ducts prepared from wild-type mice but not in CFTR knockout mice. Also, SLC26A6 a and c isoforms have a PDZ domain in their C-terminal regions by which they interact in vitro with the first and the second PDZ domains of NHE3 regulatory proteins, NHERF1 and NHERF2. These studies suggested that on pancreatic stimulation, a complex formation of CFTR-PAT1-NHERF1 might stimulate CFTR-directed luminal HCO 3 − secretion. SLC26 transporters and CFTR were shown to be mutually regulated, that is, SLC26A3- or A6-activated CFTR by increasing its overall open probability (NP o ) and reciprocally, SLC26A3- or A6-mediated transport was stimulated by CFTR. This regulation involved interaction between the STAS domain of SLC26 transporters with the regulatory domain (R) of CFTR and was facilitated by their PDZ domains ( Fig. 57.3 ). The interaction between STAS domain of SLC26A3 with R domain of CFTR was further validated in a recent study by detailed biophysical characterization of the binding profiles of phosphorylated and dephosphorylated states of R region to human SLC26A3 STAS domain. In agreement with previous report, this study also revealed that phosphorylation of R domain of CFTR enhances the interaction between the two proteins. Whether such an interaction occurs in the native intestine is not yet known and will require extensive studies.
57.1.3.1.3.2
PDZ Adapter Proteins
Previous studies have shown that under basal conditions a small fraction of SLC26A3 is associated with lipid rafts, where it also interacts with NHE3 regulatory factor (NHERF) family of PDZ adaptor proteins such as E3KARP (NHERF2), PDZK1 (NHERF3), and IKEPP (NHERF4). In this regard, a recent study identified direct protein-protein interaction between the PDZ-binding motif of SLC26A3 and the third PDZ domain of NHERF4 in vitro and in vivo in mouse intestinal tissue. Interaction with NHERF4 was shown to stimulate rapid internalization of SLC26A3 from the plasma membrane leading to reduced surface expression and anion exchange activity. In contrast, siRNA-mediated knockdown of NHERF4 in HT-29 cells upregulated the apical Cl − /HCO 3 − exchange activity. Of note, phosphorylation at serine329 in third PDZ domain of NHERF4 weakened the SLC26A3-NHERF4 interaction. Similar studies exploring the membrane trafficking events modulating the function and expression of SLC26A6 are warranted.
57.1.3.1.3.3
Basal Trafficking to the Plasma Membrane
Membrane proteins, channels, and ion transporters undergo basal recycling by exo and endocytosis to maintain optimal levels at the apical membrane. Aforementioned studies suggest that function and surface expression of SLC26A3 are regulated by membrane insertion/removal, redistribution into lipid rafts, microtubule integrity, and interaction with PDZ adapter proteins. In this context, important role of lipid rafts in exocytic trafficking of SLC26A3 was shown to be via a PI3K-dependent mechanism. Disruption of lipid raft integrity results in reduction of its activity with a modest decrease in its surface expression. A recent study revealed that the presence of ETKF PDZ interaction motif facilitated the movement of SLC26A3 from subapical pool of early endosomes to recycling endosomes followed by delivery to the plasma membrane to maintain steady-state surface levels. It was also shown that absence of ETKF motif resulted in reducing the half-life of the mutant to about 2 h, as compared to wild-type SLC26A3 with apparent half-life of 4 h. Importantly, endocytosis of SLC26A3 from the plasma membrane was found to be independent of the PDZ-binding motif and was suggested to be caveolin or clathrin mediated. Role of clathrin-mediated pathway governing recycling of SLC26A3 under basal conditions was also recently identified in Caco-2 cells. The optimal SLC26A3 function was also shown to be dependent on the integrity of intact microtubules. Therefore, intact microtubules are important for basal exocytic (but not endocytic) recycling and apical expression of SLC26A3.
57.1.3.2
Long-Term Regulation of Cl − /HCO 3 − Exchangers
Regulation of ion transporters by long-term mechanisms occurs via alterations in gene expression levels by either changing the transcription rates or stability of mRNA/protein. Following sections will discuss the current knowledge on the long-term regulation of SLC26A3 and A6 by various agents:
57.1.3.2.1
Bioactive Agents/Transcription Factors
57.1.3.2.1.1
Bioactive Lipids
Beside the acute modulation of SLC26A3, LPA also stimulates the Cl − /HCO 3 − exchange activity by regulating SLC26A3 gene expression at the transcriptional level. Long-term LPA treatment to Caco-2 cells was shown to upregulate SLC26A3 mRNA levels and promoter activity through LPA2 receptor via activation of PI3K/AKT pathway and an increase in c-fos expression. Parallel to this, another study demonstrated that Sphingosine-1-phosphate (S1P), a sphingolipid metabolite, stimulated SLC26A3 expression and function in Caco-2 cells via S1P-receptor 2 (S1PR2), PI3K/AKT activation and binding of transcription factor YY1 (Yin Yang 1) to SLC26A3 promoter. However, no change in expression of SLC26A6 was observed with both LPA and S1P. Collectively, these studies suggest that bioactive lipids such as LPA and S1P can stimulate chloride absorption by modulating the transcription of SLC26A3 via their respective G-protein coupled receptors (GPCRs) and stimulation of PI3/AKT-dependent pathways. Therefore, LPA and S1P may be of benefit as potential therapeutic agents in the treatment of infectious or inflammatory diarrhea where SLC26A3 expression is decreased.
57.1.3.2.1.2
ATRA
Antidiarrheal potential of vitamin A and its metabolites is widely established, albeit the underlying mechanisms are poorly understood. A recent study demonstrated that ATRA upregulated SLC26A3 gene expression in Caco-2 cells via retinoic acid receptor-β (RAR-β). It was further shown that ATRA stimulated the promoter activity of SLC26A3 via a RAR-β and HNF-1β-dependent pathway leading to an increase in SLC26A3 mRNA and protein expression ( Fig. 57.8 ). Besides RARs, other nuclear receptors like FXR (farnesoid-X-receptor) have also been shown to affect SLC26A3 gene expression. These studies indicate that RAR or FXR agonists could be of benefit in diarrheal diseases.

57.1.3.2.1.3
Dexamethasone
Glucocorticoids are effective antiinflammatory agents widely used in therapeutic approaches for the treatment of IBD. Previous studies have shown that dexamethasone stimulates NHE3 function and expression. Recent studies showed that dexamethasone increased SLC26A3 expression via transcriptional mechanisms in Caco-2 cells. This study demonstrated that dexamethasone treatment increased SLC26A3 promoter activity, mRNA levels, and protein expression. However, the expression level of SLC26A6 remained unaltered. These findings were further supported by in vivo studies where administration of dexamethasone to mice increased colonic SLC26A3 mRNA levels with a parallel increase in SLC26A3 protein expression.
57.1.3.2.1.4
Short-Chain Fatty Acids (SCFAs)
SCFAs, the most abundant anions in the colonic lumen, have long been known to stimulate NaCl absorption and inhibit Cl − secretion in rabbit and rat distal colon. Indeed, increased production of SCFA by addition of starch that is relatively resistant to amylase digestion has been shown to improve the effectiveness of oral rehydration solution (ORS) in the treatment of acute diarrhea in children. Previous studies have demonstrated that butyrate stimulates SLC26A3 expression and promoter activity in LS174T cells via the binding of Yin Yang 1 (YY1) and GATA transcription factors to their respective cis -elements in the promoter region of SLC26A3. More mechanistic studies elucidating the role of butyrate in regulating the expression of SLC26A3 and A6 are needed.
57.1.3.2.1.5
Hepatocyte Nuclear Factors (HNFs)
HNFs constitute a group of phylogenetically unrelated transcription factors that regulate transcription of a diverse group of genes. HNF1α and HNF1β are closely related atypical homeodomain transcription factors, whereas HNF4α belongs to the nuclear receptor superfamily with two zinc fingers as DNA-binding domain that makes it distinct from HNF1 proteins. In the intestine, HNFs have been shown to regulate the expression of genes involved in differentiation, cell fate, barrier function, ion transport, and metabolism. A recent study demonstrated that the expression of SLC26A3 was substantially reduced in ileum of HNF1α −/− mice. However, the expression of ileal and colonic SLC26A3 was completely abolished in mice deficient in both factors (HNF1α −/− /HNF1β ∆ intestine ; double mutant). This correlated with signs of severe dehydration in double knockouts compared to control littermates or those deficient in one of the HNFs. Further, direct binding of HNF1α and 1β to the putative-binding sites present in the proximal promoter and in the eighth intron of SLC26A3 genomic sequence was also demonstrated. Regulation of basal SLC26A3 promoter by HNF4α has also been demonstrated in LS174T cells. These studies suggest that HNFs play a critical role in modulating SLC26A3 expression in the normal intestine. It is interesting to note that HNF4α expression was shown to be significantly decreased in patients with IBD parallel to a decrease in DRA expression. Therefore, strategies to ameliorate HNFs downregulation in inflammation could be of therapeutic importance in attenuating diarrhea associated with IBD.
57.1.3.2.1.6
Micro-RNAs
miRs are groups of small noncoding RNAs (21–23 nucleotides) that negatively regulate gene expression at posttranscriptional level by binding to the 3′-untranslated regions (3′UTRs) of their target mRNAs. Recently, miRs have also been implicated in modulating expression of genes involved in intestinal transport and barrier function. A recent study reported the posttranscriptional regulation of SLC26A3 by miR 494 via its 3′UTR, resulting in translational repression. Transient transfection of miR-494 mimic into Caco-2 and T-84 cells significantly decreased the DRA protein expression with no change in its mRNA levels. Interestingly, reports have also shown posttranscriptional regulation of CFTR by miR-494. As SLC26A3 is functionally coupled to CFTR in the upper GI tract, identification of miR-494 as a common regulator of both these transport proteins may have physiological and clinical significance. More in-depth studies are needed to unravel the significance of this finding.
57.1.3.2.2
Cytoskeletal Elements
57.1.3.2.2.1
Keratin 8 (K8)
Keratins are the cytoskeletal intermediate filament proteins of epithelial cells. The principal keratins in the intestinal epithelia are K8, K18, and K19. Intermediate filaments are part of the essential components of the cellular cytoskeleton and play roles in cell polarization, migration, and cell’s response to mechanical and oxidative stress. Recent studies have shown that K8 knockout mice develop colitis and exhibit diarrheal phenotype with decreased NaCl absorption. A significant downregulation of SLC26A3 mRNA and protein levels in the colon and cecum of K8 knockout mice has recently been demonstrated. Parallel to the in vivo data, siRNA-mediated knockdown of K8 in Caco-2 cells also caused a significant decrease in SLC26A3 protein expression, suggesting a direct effect of K8 on SLC26A3 expression. Interestingly, no difference in NHE3 mRNA or protein levels and protein localization were observed in the knockout mice. Also, SLC26A6 and CFTR expression remained unaffected in knockout mice. These data suggest that cytoskeletal proteins such as intermediate filaments play an important role in regulation of DRA expression, and down regulation of DRA was a key contributor to diarrhea in K8 KO mice.
57.1.3.2.2.2
Myosin Vb
Myosin Vb (Myo5b) is a motor protein implicated in the apical endosome recycling and organization of cell surface polarity in epithelial cells. Loss-of-function mutations in Myosin Vb (Myo5b) are considered as the most common genetic cause of microvillus inclusion disease (MVID) characterized by substantial loss of the enterocyte brush border, presence of intracellular vacuoles with microvilli (microvillus inclusions), and intestinal villus atrophy, associated with intractable life-threatening watery diarrhea. Recently, an in vitro model of MVID was developed in which Myo5b was silenced by utilizing shRNA in C2BBe cells (Myo5bKD cells). Interestingly, Myo5b knockdown in C2BBe cells resulted in reduced mRNA levels and total protein expression of both NHE3 and DRA. A concomitant decrease in apical membrane localization of both the transporters with a significant reduction in Na + /H + and Cl − /HCO 3 − exchange activity in Myo5bKD cells was also seen. These results highlighted that (i) Myo5b altered the function and gene expression of SLC26A3 and NHE3 in the enterocytes and (ii) the functional impairment of these ion transporters may be important in the pathophysiology of MVID-associated diarrhea.
57.1.3.2.3
Intestinal Inflammation and Proinflammatory Cytokines
Increasing number of in vitro and in vivo reports now indicate a generalized downregulation of SLC26A3 in gut inflammation. Recent studies have shown that in patients with ulcerative colitis (UC), both sodium and chloride absorption are dramatically reduced. Cl − /HCO 3 − exchange activity was also decreased in surface of the colonic crypts in UC patients compared to normal, parallel to a decreased expression of SLC26A3. Additionally, significant reduction in DRA mRNA expression and complete absence of DRA protein expression in the inflamed surface epithelium of UC patients has also been reported. These data suggest downregulation of SLC26A3 function and/or expression as one of the critical features that underlies the pathogenesis of diarrhea associated with UC. However, information on abnormalities in DRA function and expression in Crohn’s disease patients is still very limited. Beside the role of SLC26A3 in IBD-associated diarrhea, recent evidence now suggests that reduced SLC26A3 expression may constitute a risk factor for the development of ulcerative colitis. For example, a two-stage GWAS (Genome Wide Association Studies) conducted in Japan using 1384 UC patients and 3057 control individuals; identified single-nucleotide polymorphism in SLC26A3 gene, which was associated with lower DRA expression. However, direct correlation of genetic deficiency in inducing inflammatory diarrhea needs further investigation.
Further, in vitro studies using human colonic Caco-2 cell line demonstrated that SLC26A3 mRNA was reduced in response to the proinflammatory cytokine, IL-1β. In addition, downregulation of both SLC26A3 and A6 expression in response to treatment of Caco-2 monolayers with proinflammatory cytokine interferon-γ (IFNγ) was also reported. Deletion and mutation studies utilizing the promoter regions of A3 and A6 revealed distinct mechanisms of inhibition by IFNγ. For example, interferon regulatory factor-1 (IRF-1) transcription factor was involved in mediating IFNγ-mediated inhibition of A6 promoter, whereas the inhibition of A3 promoter occurred via a JAK (Janus kinase)/STAT-1 pathway ( Fig. 57.8 ). These studies indicate that repression of both SLC26A3 and SLC26A6 promoter activities along with NHE3 promoter by IFNγ may lead to decreased NaCl absorption in the ileum and colon. Another recent study reported a significant decrease in promoter activity of SLC26A3, with a concomitant decrease in DRA mRNA and protein expression in response to direct TNFα treatment in HT-29 cells. This study further showed the involvement of TNFα-induced activation of NFκB pathway in inhibiting DRA expression at the transcriptional level, which may partly contribute to IBD-associated diarrhea ( Fig. 57.8 ). However, TNFα treatment did not alter the mRNA levels of SLC26A6. Significant reduction in SLC26A3 mRNA expression has also been shown in murine inflammatory models such as HLA-B27/β2m transgenic rats and IL-10 knockout mice. Substantial reduction in SLC26A3 mRNA and protein expression associated with a diarrheal phenotype have also been reported in mouse model of colitis induced by dextran sulfate sodium (DSS). Further, a pronounced decrease in SLC26A3 mRNA and protein expression in ileum and mid distal colon has been reported in TNF +/ΔARE mice (TNFα overexpressing mouse model that closely resembles CD and displays strong expression of TH1 cytokines). Significant decrease in Cl − /HCO 3 − exchange activity with no change in DRA mRNA and protein expression was recently reported in rabbit model of chronic ileitis. In general, most of these studies suggest that during intestinal inflammation, a loss of function and expression of SLC26A3 appear to be a common event leading to diarrhea.
57.1.3.2.4
Gut Microbes
57.1.3.2.4.1
Probiotics
Lactobacillus acidophilus : Consistent with the short-term effects of Lactobacillus on SLC26A3 function as described above, recent studies utilizing both in vitro and in vivo mouse models demonstrated that LA upregulated SLC26A3 mRNA and protein expression ( Fig. 57.7 ). There was no effect of LA on SLC26A6 expression. Further, SLC26A3 promoter activity was upregulated by LA indicating the involvement of transcriptional mechanisms. The effects of LA were mimicked by the culture supernatant obtained from live bacteria indicating that LA-secreted soluble effector molecules modulated SLC26A3 function and expression. Since probiotics-based therapies are generally administered for a period of several days to weeks, it could be speculated that involvement of both short-term and long-term (transcriptional) mechanisms may serve to exert beneficial effects via dual mechanisms: (i) increasing the total cellular levels (transcriptional) and (ii) increased plasma membrane levels (posttranslational) via a PI3K-dependent trafficking pathway.
Bifidobacteria : Similar to LA, probiotic strains of Bifidobacterium such as Bifidobacterium breve , Bifidobacterium infantis , and Bifidobacterium bifidum were also shown to upregulate SLC26A3 expression at the transcriptional level. Treatment of Caco-2 cells with culture supernatants from various Bifidobacterium species enhanced Cl − /HCO 3 − exchange activity, with a concomitant increase in DRA mRNA and protein expression via activation of ERK1/2 MAPK pathway ( Fig. 57.7 ). In addition, an induction of colonic SLC26A3 mRNA and protein levels (but not in SLC26A6) in response to administration of Bifidobacterium species in mice was also demonstrated. This study further highlighted the benefits of Bifidobacterial formulations as potential antidiarrheal agents and warrants detailed investigation of the soluble factors generated by these species responsible for upregulation of SLC26A3.
57.1.3.2.4.2
Pathogens
Citrobacter rodentium : C. rodentium is an attaching and effacing murine pathogen. It is the mouse counterpart to model human pathogen EPEC. A recent study showed that infection with C. rodentium caused a significant increase in fecal chloride levels, hypochloremia, and fatal diarrhea. This was attributed to extensive fluid loss along with almost complete loss of SLC26A3 expression. Substantial increase in pro-inflammatory cytokines such as IL-1β, IFNγ and CXCL-l together with activation of STAT3 has also been reported in colon of C. rodentium-infected mice. This study also reported a significant decrease in colonic expression of SLC26A3, SLC26A6, and NHE3 after C. rodentium infection. These results further suggest that inhibitory effects of C. rodentium on SLC26A3 expression could at least in part be due to inflammation. Of note, probiotic L. acidophilus (LA) resulted in an attenuation of C. rodentium -induced inflammation and inhibition of NHE3 and DRA expression. Identification of molecular mechanisms involved in pathogenesis of C. rodentium -induced diarrhea and their manipulation by probiotics such as LA need further investigation.
Helicobacter : Previous studies have shown that duodenal bicarbonate secretion (DBS) is diminished in murine model of H. pylori infection and in patients with H. pylori -associated duodenal ulcers. In this regard, a recent study showed reduced DBS associated associated with a significant decrease in mRNA and protein expression of SLC26A6 and CFTR in duodenal biopsies of H. pylori infected patients. However, SLC26A3 expression remained unaffected. Further, H. pylori eradication resulted in restoring the function and expression of both CFTR and SLC26A6. As, DBS serves as a defense mechanism against mucosal injury, these data indicate that downregulation of SLC26A6 and CFTR function and expression underlie the pathology of H. pylori -associated duodenal ulcers. These studies also indicate that SLC26A6 may play a predominant role in DBS compared to SLC26A3.
57.1.3.2.5
Circadian Regulation of DRA Expression
The functional clock genes in the myenteric plexus and epithelial cells in the GI tract govern the diurnal rhythms of many physiological functions such as cell proliferation, motility, digestion, and transport processes. Circadian regulation of transporters such as, NHE3, SGLT1, GLUT2, GLUT5, and PEPT1 in the murine intestine has been reported. In this regard, a recent study reported circadian rhythmicity in the gene expression of DRA in rat colon. This study also demonstrated that restricted feeding significantly advanced the time for DRA gene expression in the circadian clock. Mechanistic studies identifying the effects of direct binding of clock gene components at DRA promoter are required to further elucidate the mechanism(s) of circadian regulation of DRA expression.
57.1.3.2.6
SLC26A3 in Tumorigenesis
Role of transmembrane transporters and ion channels as tumor suppressors/promoters has been recently demonstrated. SLC26A3 was in fact identified as a putative tumor suppressor gene, downregulated in colon carcinoma. This has been supported by the observations such as expanded proliferative zone in the colon of SLC26A3 knockout mice (suggestive of hyperplasia) and induction of growth suppression in cancer cell lines after ectopic expression of the transporter. Moreover, colon tumor progression has been positively correlated with reduced expression of SLC26A3 mRNA and protein in patients with colorectal carcinoma. The tumor suppressor role of SLC26A3 is also highlighted in a recent study suggesting it as a prognostic marker in colon cancer. For example, The Cancer Genome Atlas (TCGA) database analysis correlates low SLC26A3 expression with decreased overall survival in colon cancer patients, though not statistically significant. Importantly, SLC26A3 is expressed only in differentiated intestinal epithelial cells and plays an important role in regulating intracellular pH. Whether diminished SLC26A3 expression in carcinogenesis is an adaptive mechanism of the tumor cells to promote growth favored by reduced cellular acidity or is a feature of undifferentiated tumor cells is not yet defined. Thus, SLC26A3 appears as a promising diagnostic and therapeutic target in cancer, but the precise role for the transporter in the pathogenesis of colon cancer has yet to emerge.
57.1.4
SLC4 Gene Family of Anion Exchangers
The SLC4 transporter family, or the bicarbonate-transporter family, which includes the products of 11 human genes (SLC4A1–5; A6, A7–11) is composed of Na + -independent anion exchangers (AEs) SLC4A1 (AE1), SLC4A2 (AE2), and SLC4A3 (AE3) and Na + -coupled bicarbonate transporters (NBCs). NBCs are further categorized into (i) Na + /bicarbonate cotransporters (NBCs) that are either electrogenic, SLC4A4 (NBCe1 or NBC1), SLC4A5 (NBCe2 or NBC4) or electroneutral SLC4A7 (NBCn1), SLC4A10 (NCBn2) and (ii) the electroneutral Na + -driven Cl − -bicarbonate exchanger SLC4A8 (NDCBE). The roles of the other three members of the human SLC4 family remain poorly described and least functionally understood. SLC4A9 (AE4) is a Na + -independent transporter, SLC4A11 (BTR) is an electrogenic Na + -dependent borate transporter in mammalian cells and SLC4A6 is a pseudogene. There are structural and functional similarities between all transporters of the SLC4 gene family. Another similarity observed among all members of the SLC4 family is the inhibition of their transport (functional) activity by disulfonic stilbenes, DIDS, and SITS.
In the intestine, Na + -HCO 3 − cotransporters are expressed on the basolateral membranes of duodenal and colonic epithelial cells in the human and rabbit and are known to mediate the entry of HCO 3 − into the cells followed by its secretion to the intestinal lumen. Many members of the SLC4 anion exchangers (AEs) are expressed in the small intestine and colon. SLC4A1 (AE1) has been shown to be expressed in the rat small intestine and distal colon, while its expression is very low in the human colon and not detected in any region of the human small intestine. Previous studies in rats have suggested the direct involvement of AE1 in chloride absorption due to its localization to the luminal membrane of intestinal epithelial cells. However, in the humans, AE1 is not involved in Cl − absorption. SLC4A9 (AE4) polypeptide was found to be expressed on the apical membrane of gastric mucous cells and duodenal epithelial cells in mouse, rabbit, and human, suggesting that AE4 is an important member of the SLC4 gene family as it is expressed on the luminal surface of intestinal epithelial cells. However, the role of AE4 in chloride absorption and HCO 3 − secretion in gastric and duodenal epithelial cells is also not clear. Thus, additional studies are needed in the future to ascertain the function of AE4. SLC4A2 (AE2) has been shown to be expressed on the basolateral membranes of ileal and colonic epithelial cells in rabbit, mouse, and human, where it may be involved in vectorial chloride transport and other cellular functions, for example, maintenance of intracellular pH. In the stomach, AE2 protein is localized basolaterally in gastric parietal cells, where it plays an important role in acid secretion. SLC4A3 (AE3) also functions as a Cl − /HCO 3 − exchanger and was shown to be expressed predominantly on the basolateral but not the apical membrane of the epithelial cells in the human intestine. As mentioned above, anion exchangers (AE1, 2, 3, and 4) do not seem to be important in vectorial Cl − absorption in the human intestine. Therefore, this chapter will not focus on the structure/function characteristics and regulation of these anion exchangers.
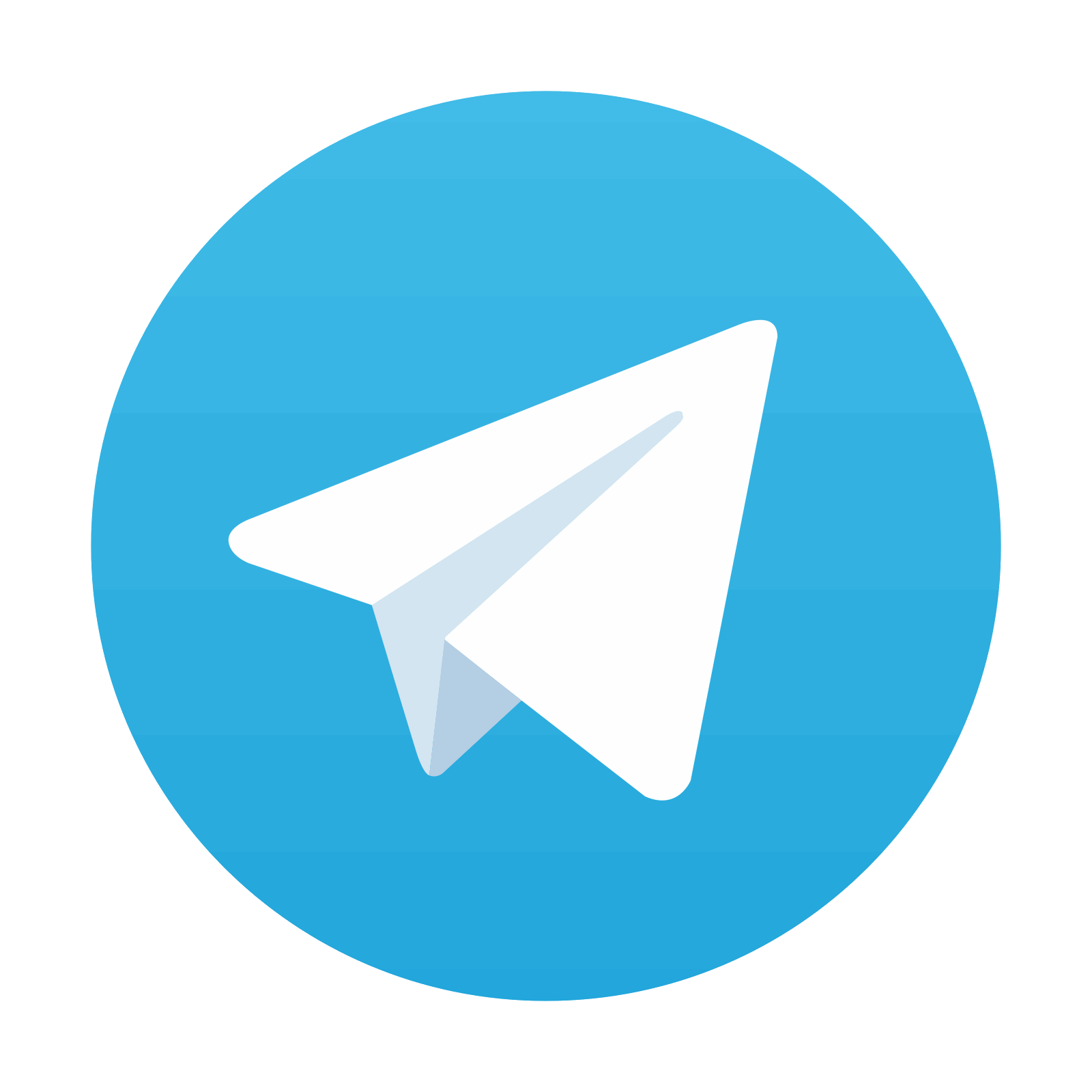
Stay updated, free articles. Join our Telegram channel

Full access? Get Clinical Tree
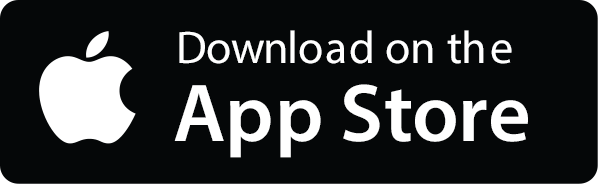
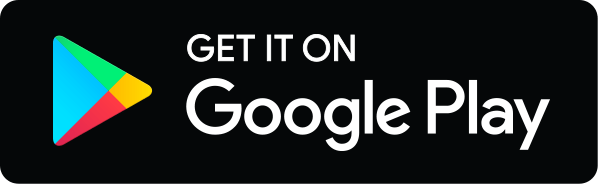