Semen characteristics
WHO
1980
WHO
1987
WHO
1992
WHO
1999
WHO
2010a
Volume (ml)
ND
≥2
≥2
≥2
1.5
Sperm count (106/ml)
20-200
≥20
≥20
≥20
15
Total sperm count (106)
ND
≥40
≥40
≥40
39
Total motility (% motile)
≥60
≥50
≥50
≥50
40
Progressive motilityb
≥ 2c
≥25 %
≥25 % (grade a)
≥25 % (grade a)
32 % (a + b)
Vitality (% alive)
ND
≥50
≥75
≥75
58
Morphology (% normal forms)
80.5
≥50
≥30d
(14)e
4f
Leukocyte count (106/ml)
<4.7
<1.0
<1.0
<1.0
<1.0
5.3 Semen Collection
Semen must be collected after a standardized period of abstinence, usually 3 days (2–4 days), and the period must be indicated in the laboratory report. The time of collection, when the semen was liquefied, must be reported, as a delay of longer than an hour may adversely affect sperm motility (World Health Organization 2010). The standardization is essential to minimize fluctuations in semen quality, especially sperm count and sperm motility, due to short/long abstinence.
5.4 Macroscopic Evaluation of Semen
Semen samples can show substantial variation in physicochemical properties including color and odor. Pathologically, seminal discoloration may be due to fresh blood, drugs (pyridium), jaundice, or contamination of semen with urine (e.g., bladder neck dysfunction). The complete semen analysis includes analysis of the semen volume, pH, liquefaction or non-liquefaction, and viscosity.
Volume
The normal volume of ejaculate after 2–7 days of sexual abstinence ranges from 2 to 6 ml. However, there are other possibilities as follows (Vasan 2011; Bornman and Aneck-Hahn 2012):
- 1.
Aspermia: No ejaculate after orgasm.
- 2.
Hypospermia: Less than 0.5 ml of semen. This can be due to improper collection, hypogonadism, partial retrograde ejaculation, and congenital bilateral absence of the vas deferens (CBAVD), and obstruction of lower urinary tract may yield low volume.
- 3.
Hyperspermia: More than 6 ml of semen. This can be attributed to prolonged abstinence or excessive secretion from the accessory sex glands and also occurs in cases of male accessory gland infection (World Health Organization 2010).
pH
The main component of semen is a coagulated alkaline fluid that comes from the seminal vesicles. This fluid along with the sperm from the vas deferens empties through the ejaculatory duct. Prostatic fluid, the second largest component of seminal volume, generally has a relatively acidic pH of 6.5 and combines with the seminal fluid and sperm in the urethra. Prostatic fluid does not traverse the ejaculatory ducts. Normal semen pH is in the range of 7.2–8.2 and it tends to increase with time after ejaculation. Changes in pH of semen are usually due to inflammation of the prostate or seminal vesicles. A low volume sample with measured pH below 7.0 indicates obstruction of the ejaculatory ducts.
Liquefaction
Liquefaction of semen depends on coagulation of proteins found in the seminal fluid as well as the liquefaction by prostate-specific antigen, a proteolytic enzyme, secreted by the prostate. This process may take up to 60 min. If complete liquefaction does not occur after 60 min, it should be noted. Exact liquefaction time is of no diagnostic importance unless > 2 h elapse without any change. The clinical significance of abnormalities in liquefaction is still controversial (Keel 1990). Failure to liquefy is usually a sign of inadequate secretion of the proteolytic enzymes – fibrinolysin, fibrinogenase, and aminopeptidase – by the prostate (Amelar 1962). On the other hand, absence of coagulation may indicate ejaculatory duct obstruction or congenital absence of seminal vesicles.
Semen Viscosity
Viscosity measures the resistance of the seminal fluid to flow. High viscosity may interfere with determination of sperm motility, concentration, and antibody coating of spermatozoa. Normally, semen coagulates upon ejaculation and usually liquefies within 15–20 min. Semen that remains a coagulum is termed non-liquefied, whereas that which pours in thick strands instead of drops is termed hyperviscous. Importantly, liquefaction should be differentiated from viscosity, as abnormalities in viscosity can be the result of abnormal prostate function and/or the use of an unsuitable type of plastic container. Viscosity of semen is noted after liquefaction, although the clinical significance of hyperviscous semen is controversial. There is no correlation between seminal hyperviscosity and semen cultures, leukocytes, or presence of sperm antibodies; however, worse outcomes after in vitro fertilization (IVF) with seminal hyperviscosity have been observed (Munuce et al. 1999; Esfandiari et al. 2008). Sperm processing prior to intrauterine insemination (IUI) can be considered, if there is a clinical concern for hyperviscosity.
5.5 Microscopic Sperm Analysis
Sperm Concentration
Concentration of sperm in unstained preparations of fresh/washed semen sample is determined using, preferably, a phase-contrast microscope with volumetric dilution and hemocytometry. Sperm count is typically reported as concentration (millions of sperm per milliliter) as well as total sperm count (sperm concentration × ml of semen) in the ejaculate. Normozoospermia, oligozoospermia, and azoospermia are diagnosed based upon total sperm count. Azoospermia refers to the absence of sperm in the seminal plasma. Prior to the diagnosis of azoospermia, the sample should be centrifuged and the pellet examined for the presence of sperm. Oligozoospermia (also often called oligospermia) refers to seminal plasma concentration < 15 million/ml. This finding can accompany a variety of defects and has implications for the type of assisted reproductive options that can be utilized, as there are significant reductions in pregnancy rates (Smith et al. 1977).
Motility
The efficient passage of spermatozoa through cervical mucus is dependent on rapid-progressive motility, that is, spermatozoa must have a forward progression of a minimum of 25 μm/s (Björndahl 2010; Lindholmer 1974). Reduced sperm motility can be a symptom of disorders related to male accessory sex gland secretion and the sequential emptying of these glands. Rapid and slow-progressive motility is calculated by the speed at which sperm moves with flagellar movement in a given volume as a percentage (range 0–100 %) by counting 200 sperms and classified as follows:
- A.
(Rapid progressive motility: > 25 μm/s at 37 °C and > 20 μm/s at 20 °C
Note: 25 μm is approximately equal to 5 head lengths or half a tail length).
- B.
Slow or sluggish progressive motility
- C.
Nonprogressive motility (<5 μm/s)
- D.
Immotile
A normal semen analysis must contain at least 50 % grade A and B progressively motile spermatozoa (Table 5.1). If greater than 50 % of sperms are immotile, then the sperms should be checked for viability. Persistent poor motility is a good predictor of failure in fertilization, an outcome that is actually more important when making decisions regarding a couple’s treatment options (Aitken et al. 1985).
Vitality
Supravital staining differentiates between live and dead sperm and is assessed when sperm motility is < 50 %. A large proportion of vital, but immotile, sperm may indicate structural defects in the sperm tail (World Health Organization 1999) or Kartagener’s syndrome. A high percentage of immotile, nonviable (dead) sperm may indicate epididymal pathology (World Health Organization 2010). Antisperm antibodies (ASA) may also be present, if the immotile sperms are dead (Björndahl et al. 2010a).
Morphology
The staining of a seminal smear (Papanicolaou Giemsa, Shorr, and Diff-Quik) allows the quantitative evaluation of normal and abnormal sperm morphological forms in an ejaculate. Smears can be scored for morphology using the World Health Organization (WHO) classification or by Kruger’s strict criteria classification (Menkveld et al. 1990). WHO method classifies abnormally shaped spermatozoa into specific categories based on specific head, tail, and mid-piece abnormalities, which is based on the appearance of sperm recovered from postcoital cervical mucus or from the surface of zona pellucida (>30 % normal forms). In contrast, Kruger’s strict criteria classifies sperm as normal only if the sperm shape falls within strictly defined parameters of shape, and all borderline forms are considered abnormal (>14 % normal forms).
- 1.
Head defects: Large, small, tapered, pyriform, round, amorphous, vacuolated (>20 % of the head area occupied by unstained vacuolar areas) heads with small acrosomal area (<40 % of head area), double heads, or any combination of these
- 2.
Neck and mid-piece defects: Bent neck; asymmetrical insertion of mid-piece into the head; thick, irregular mid-piece; abnormally thin mid-piece; or any combination of these
- 3.
Tail defects: Short, multiple, hairpin, broken, bent, kinked, coiled tails, or any combination of these
- 4.
Cytoplasmic droplets: Greater than one-third of the area of a normal sperm head
Morphology should be used along with other parameters, and not as an isolated parameter, when determining clinical implications. It is important to realize that, in general, pregnancy is possible with low morphology scores and that both motility and morphology have demonstrated prognostic value, as do combinations of parameters (Van Waart et al. 2001; Keegan et al. 2007). The clinical implications of poor morphology scores remain highly controversial. The initial studies using rigid criteria reported that patients undergoing in vitro fertilization (IVF) who had greater than 14 % normal forms had better fertilization rates (Coetzee et al. 1998). Later studies reported that most impairment in fertilization rates occurred with morphology scores of less than 4 % (Menkveld et al. 1990).
Morphology is a particularly challenging parameter to interpret because of the subjective nature of the classification and the presence of multiple classification systems, as well as controversy about the implications of various morphological features. There are studies correlating fertilization rates with morphology scores and other studies which show no relationship between morphology scores and IVF results (Deck and Berger 2000; Schlegel 1997). As there are a number of scoring methodologies, the clinician should explore and adopt a particular methodology and reporting for their laboratory. In spite of the controversy about overall morphology scores, absence of acrosomes or globozoospermia is highly predictive of failure of fertilization (Male Infertility Best Practice Policy Committee of the American Urological Association and Practice Committee of the American Society for Reproductive Medicine 2006).
In view of these findings, it is beneficial for the physician to have a detailed analysis of the morphological defects in addition to the percentage of normal forms. In the case of globozoospermia, treatment with intracytoplasmic sperm injection (ICSI) can be more successful compared to IUI (Baker et al. 1994). For some morphology, such as pin head or short tailed, sperms fail to have pronuclei fusion leading to failure of even ICSI (Dunson et al. 2004). Overall, there is significant difficulty with defining the relationship between morphology and pregnancy rates, especially with the management of patients with low morphology scores (Abbey et al. 1992). The current evidence suggests that, in general, sperm morphology scores should not be used in isolation to make patient management decisions.
5.6 Computer-Aided Sperm Analysis (CASA)
Advances in technology and the use of fluorescent DNA stains have facilitated development of computer-aided sperm analysis (CASA). Determination of sperm concentration and concentration of progressively motile spermatozoa has been possible due to the availability of advanced tail detection algorithms (Zinaman et al. 1996; Garrett et al. 2003). CASA can be used for routine diagnostic applications when specimen is prepared with proper care and adequate quality control procedures are in place. CASA systems with semiautomated morphology units are available and can be used to measure sperm concentration, motility, kinematics, and morphology with high precision. Studies have also shown the significance of CASA sperm concentration and kinematic parameters in the determination of in vitro and in vivo fertilization rates (Garrett et al. 2003; Liu et al. 1991; Barratt et al. 1993). Progress in digital image analysis has brought about greater objectivity and improved precision to quantitative assessment of sperm morphology (Garrett and Baker 1995).
Manual semen analysis lacks the ability to measure the kinematics of sperm motion. CASA is potentially useful because of its capacity to analyze sperm motion (sperm head and flagellar kinetics), some of which have been shown to be related to IVF outcome (Fréour et al. 2010). Important kinematic parameters are as follows:
- A.
Curvilinear velocity: Curvilinear velocity (VCL) is the measure of the rate of travel of the centroid of the sperm head over a given time period.
- B.
Average path velocity: Average path velocity (VAP) is the velocity along the average path of the spermatozoon.
- C.
Straight-line velocity: Straight-line velocity (VSL) is the linear or progressive velocity of the cell.
- D.
Linearity: Linearity of forward progression (LIN) is the ratio of VSL to VCL and is expressed as percentage.
- E.
Amplitude of lateral head displacement: Amplitude of lateral head displacement (ALH) of the sperm head is calculated from the amplitude of its lateral deviation about the cell’s axis of progression or average path.
Although CASA is very accurate for determining the details of sperm kinetics, manual assessment of semen is much more accurate in discerning among debris, crystals, and immotile and dead sperm heads. Therefore, manually assessed sperm concentrations and number of immotile spermatozoa are much more reliable than corresponding data obtained by CASA, provided individual is adequately trained with appropriate internal and external quality control measures (Makler 1978; Ginsburg and Armant 1990).
5.7 Other Markers
The secretion of zinc by the prostate is androgen dependent, and a level of < 2.4 μmol/ejaculate indicates a low contribution of the fluid to the ejaculate, incomplete collection of the ejaculate, prostatic inflammation, or androgen insufficiency (Björndahl et al. 2010b). Fructose is another androgen-dependent secretion emanating mainly from the seminal vesicles, with a small contribution from the epithelial cell of the secretory epithelium in the ampulla of the vas deferens. Seminal fructose is used as a marker of the seminal vesicles and < 13.0 μmol/ejaculate is considered abnormal. This is seen in hypogonadal men after a short abstinence time, and where ejaculation or emission of fluid is impaired, such as in neuromuscular diseases, after surgery, in cases of drug use, and in obstruction in the ejaculatory ducts, or with inflammation in the vesicles or prostate that may hinder emission (Bornman and Aneck-Hahn 2012).
Infection of the male reproductive tract can directly or indirectly cause infertility (Mortimer 1994a). Pyospermia is a laboratory finding categorized as the abnormal presence of leukocytes in human ejaculate and may indicate genital tract inflammation (Anderson 1995). Polymorphonuclear (PMN) leukocytes are the primary sources of reactive oxygen species (ROS) that cause inflammation, and peroxidase staining is used to detect their presence (Wolff et al. 1992).
Presence of agglutinated clumps of moving sperm in the semen sample could hamper the passage of sperm through the cervical mucus, and zonal binding and passage (Mortimer 1994b). Such clumps are formed by the exposure of spermatozoa to systemic immune defense system, due to the release of antisperm antibodies (ASA). ASA can also cause cell death and immobilized sperm cells. Detection of ASA bound to the surface of motile sperm is carried out by the mixed agglutination reaction assay (MAR test; only for IgGs) and the immuno-bead binding assay (for IgA, IgG, and IgMs) (Jarow and Sanzone 1992).
5.8 Sperm Functional Tests
Tests of Sperm Capacitation
Capacitation is a series of biochemical and structural changes that spermatozoa go through to undergo acrosome reaction (AR) and be able to fertilize. The process takes place in the female genital tract but can be induced in vitro by incubating spermatozoa with capacitation-inducing media. It is thought to have a role in preventing the release of lytic enzymes until spermatozoa reach the oocyte (Tesarik 1989). One of the signs of capacitation is the display of hyper-activation by spermatozoa. At the present time, the clinical value of sperm capacitation testing remains to be determined.
Tests of Hemizona and Zona Pellucida Binding
The interaction between spermatozoa and the zona pellucida is a critical event leading to fertilization and reflects multiple sperm functions (i.e., completion of capacitation as manifested by the ability to bind to the zona pellucida and to undergo ligand-induced AR) (Oehninger et al. 1994; Liu and Baker 2003; Consensus workshop on advanced diagnostic andrology techniques. ESHRE (European Society of Human Reproduction and Embryology) Andrology Special Interest Group 1996). The most common sperm-zona pellucida binding tests currently utilized are the hemizona assay (or HZA) and a competitive intact-zona binding assay (Quintero et al. 2005; Fénichel et al. 1991). The HZA which uses non-fertilized oocytes is useful to determine the cause, in couples who have failed to fertilize during regular IVF. As the binding is species-specific, human zona must be used, thus limiting the utility of these assays (Fénichel et al. 1991; Cross et al. 1986). The induced AR assays appear to be equally predictive of fertilization outcome and are simpler in their methodologies. The use of a calcium ionophore to induce AR is currently the most widely used methodology (Henkel et al. 1993; Katsuki et al. 2005).
Sperm Penetration Assay
This assay is also called as sperm capacitation index or zona-free hamster oocyte penetration assay. The concept of the sperm penetration assay was introduced by Yanagamachi (1972; Yanagimachi et al. 1976). It yields information regarding the fertilizing capacity of human spermatozoa by testing capacitation, AR, sperm/oolemma fusion, sperm incorporation into the ooplasm, and decondensation of the sperm chromatin during the process. However, penetration of the zona pellucida and normal embryonic development are not tested. The spermatozoa penetration assay (SPA) utilizes the golden hamster egg, which is unusual in that removal of its zona pellucida results in loss of all species specificity to egg penetration. Thus, a positive SPA does not guarantee fertilization of intact human eggs nor their embryonic development, whereas a negative SPA has not been found to correlate with poor fertilization in human IVF (Yanagimachi et al. 1976). The acrosin assay, an indirect measure of sperm’s penetrating capability, measures acrosin, which may be responsible for penetration of the zona pellucida and also triggering the AR (Rogers and Brentwood 1982). Measurement of acrosin is thought to correlate with sperm binding to and penetration of the zona pellucida (Cross et al. 1986; Cummins et al. 1991).
Tests of Sperm DNA Damage
Mammalian fertilization involves the direct interaction of the sperm and the oocyte, fusion of the cell membranes, and union of male and female gamete genomes. Although a small percentage of spermatozoa from fertile men also possess detectable levels of DNA damage, which is repaired by oocyte cytoplasm, there is evidence to show that the spermatozoa of infertile men possess substantially more DNA damage that may adversely affect reproductive outcomes (Evenson et al. 1999; Zini et al. 2001). There appears to be a threshold of sperm DNA damage which can be repaired by oocyte cytoplasm (i.e., abnormal chromatin packaging, protamine deficiency) beyond which embryo development and pregnancy are impaired (Ahmadi and Ng 1999; Cho et al. 2003).
- A.
DNA damage – direct tests
- (a)
Terminal deoxynucleotidyl transferase-mediated deoxyuridine triphosphate (dUTP) nick end-labeling (TUNEL) assay
- (b)
COMET assay
- (a)
- B.
DNA damage – indirect tests
- (a)
Sperm chromatin structure assay (SCSA)
- (b)
Sperm chromatin dispersion assay
- (c)
Sperm fluorescence in situ hybridization analysis (FISH)
- (a)
Overall, studies suggest that there is no significant relationship between sperm DNA damage and fertilization rate or pregnancy outcomes at IVF or IVF/ICSI (Bungum et al. 2007; Payne et al. 2005; Zini et al. 2005; Borini et al. 2006; Benchaib et al. 2007). However, there is evidence to suggest that sperm DNA damage is associated with poor pregnancy outcome after standard IVF (Lin et al. 2008; Frydman et al. 2008). Sperm FISH analysis may be useful in (a) infertile men with sex chromosome numerical anomalies, prior to ICSI; (b) infertile men with structural chromosome anomalies, prior to ICSI; (c) infertile men with severe oligozoospermia, prior to ICSI; and (d) couples with a history of recurrent miscarriages and trisomic pregnancies.
Assessment of Reactive Oxygen Species
Reactive oxygen species, (ROS) also referred to as free radicals, are formed as a by-product of oxygen metabolism. Contaminating leukocytes are the predominant source of ROS in these suspensions (Aitken et al. 1989a, b). They can be eradicated by enzymes (e.g., catalase or glutathione peroxidase) or by nonenzymatic antioxidants, such as albumin, glutathione, and hypotaurine, as well as by vitamins C and E. Small amounts of ROS may be necessary for the initiation of critical sperm functions, including capacitation and AR. On the other hand, a high ROS level produces a state known as oxidative stress that can lead to biochemical or physiologic abnormalities with subsequent cellular dysfunction or cell death. Significant levels of ROS can be detected in the semen of 25 % of infertile men, whereas fertile men do not have a detectable level of semen ROS (Aitken et al. 1989b, 1991; Agarwal et al. 2006). Sperm ROS can be measured by using cellular probes coupled with flow cytometry by the detection of chemiluminescence (Marchetti et al. 2002). Briefly, this is done by incubating fresh semen or sperm suspensions with a redox-sensitive, light-emitting probe (e.g., luminol) and by measuring the light emission over time with a luminometer. The clinical value of semen ROS determination in predicting IVF outcome remains unproven, but identifying oxidative stress as an underlying cause of sperm dysfunction has the advantage for suggesting possible therapies. Administration of antioxidants has been attempted in several trials with mixed results. Currently, there are no established semen ROS cutoff values that can be used to predict reproductive outcomes (Agarwal et al. 2005, 2008).
Sperm Proteomics
Sperm proteomics, an experimental technique, used extensively in several branches of medicine, may identify some of the molecular targets implicated in sperm dysfunction (Aitken and Baker 2008). Sperm proteomics allows comparison of protein structure of normal and defective spermatozoa (Aitken 2010).
5.9 Pre-2010 WHO Guidelines
Prior to 2010, semen analyses were performed mainly according to the WHO guidelines (World Health Organization 1992) to obtain volume, pH, sperm concentration, motility, and morphology. Sperm concentration was determined with the use of a Makler counting chamber. Motility was expressed as the percentage of motile spermatozoa and their mean speed, or motility quality (on a scale of 1–6, where 1 stands for immotile and 6 for very fast progressive motile, i.e., 100 μm/s). For sperm morphology evaluation, two slides were prepared of each sample after incubation of the semen samples with trypsin (10 min at room temperature); one slide was used for routine morphology evaluation by WHO criteria and the other for strict criteria evaluation. For evaluation according to WHO criteria, smears were flame-fixed and stained with methylene blue/eosin. At least 100 cells were examined per slide, with a final magnification of x1000. Each slide was evaluated independently by two technicians. There should not be any statistically significant difference (by Pearson’s correlation matrix analysis) between the results of the two observers. The slides for evaluation by strict criteria were stained according to the Papanicolaou method and evaluated (Menkveld et al. 1990). In addition to the morphology evaluation according to strict criteria, the acrosome index (AI) and teratozoospermia index (TZI) were also determined (World Health Organization 1992; Menkveld and Kruger 1996).
Teratozoospermia Index
The TZI is an indication of the number of abnormalities present per abnormal spermatozoon. According to the 1992 WHO manual (World Health Organization 1992), each abnormal spermatozoon can have one to four abnormalities, viz., a head abnormality, a neck/mid-piece abnormality, a tail abnormality, or the presence of a cytoplasmic residue. These abnormalities can occur as a single defect or in a combination of two, three, or all four abnormalities simultaneously. The classification of spermatozoa for the TZI is recorded simultaneously, on a five-key laboratory counter, with the recording of spermatozoa as normal or abnormal, in specific classes. The total number of abnormalities recorded are added together and divided by the total number of abnormal spermatozoa, i.e., 100 minus the percentage of morphologically normal spermatozoa.
Acrosome Index
Sperm acrosomal morphology was evaluated by light microscopy at ×1250 oil magnification based on acrosomal size and form as well as staining characteristics (Menkveld and Kruger 1996). Results were expressed as the AI (% normal acrosomes). For the evaluation of acrosome morphology, the same principles as for the evaluation of normal sperm morphology according to strict criteria are applicable. For an acrosome to be regarded as normal, the acrosome must have a smooth normal oval shape, with the same dimensions as for a normal spermatozoon. Acrosomes must be well-defined and comprising about 40–70 % of the normal-sized sperm head. The post-acrosomal part of the sperm head can be abnormal, but the rest of the spermatozoon must be normal; thus no neck/mid-piece and tail abnormalities and no cytoplasmic residue may be present. If the spermatozoon is classified as normal, the acrosome must always be classified as normal. The acrosome evaluation can be performed simultaneously with the routine morphology evaluation and the TZI, with the use of two laboratory counters. As with the normal sperm morphology, at least 100 spermatozoa are evaluated. The repeatability of the AI is be determined and should be within acceptable limits.
Reference Intervals
Reference intervals are the most widely used tool for the interpretation of clinical laboratory results. Reference interval development has classically relied on concepts elaborated by the International Federation of Clinical Chemistry Expert Panel on Reference Values during the 1980s. These guidelines involve obtaining and classifying samples from a healthy population of at least 120 individuals and then identifying the outermost 5 % of observations to use in defining limits for two-sided or one-sided reference intervals. Pre-2010 WHO guidelines were based on data obtained from laboratories that used different methodologies and examined different male populations, not supported by standardized methods or without the definition of fertile population. The male population studied included men without proven paternity, patients of human reproduction clinics that sought treatment, semen donors, and vasectomy candidates. Semen donors can be fertile and vasectomy candidates are very likely to be fertile, although there is no data about how long it took for their partners to get pregnant (Cooper et al. 2010). The cutoff point of 20 × 106/ml was suggested as the lower normal value for sperm concentration in an ejaculate (World Health Organization 1999). However, there are studies indicating sperm concentrations of subfertile men to be less than 13.5 × 106/ml (Guzick et al. 2001) and 31.2 × 106/ml for fertility status (Nallella et al. 2006). Therefore, caution must be exercised with interpretation of the semen analysis based upon the reference values as men may be infertile with “normal” semen parameters or alternatively can be fertile with markedly “abnormal” semen profiles. There is likely no upper limit of semen morphology, motility, or count as pregnancy rates appear to generally increase with increasing numbers as well as improved sperm morphology and motility (Garrett et al. 2003).
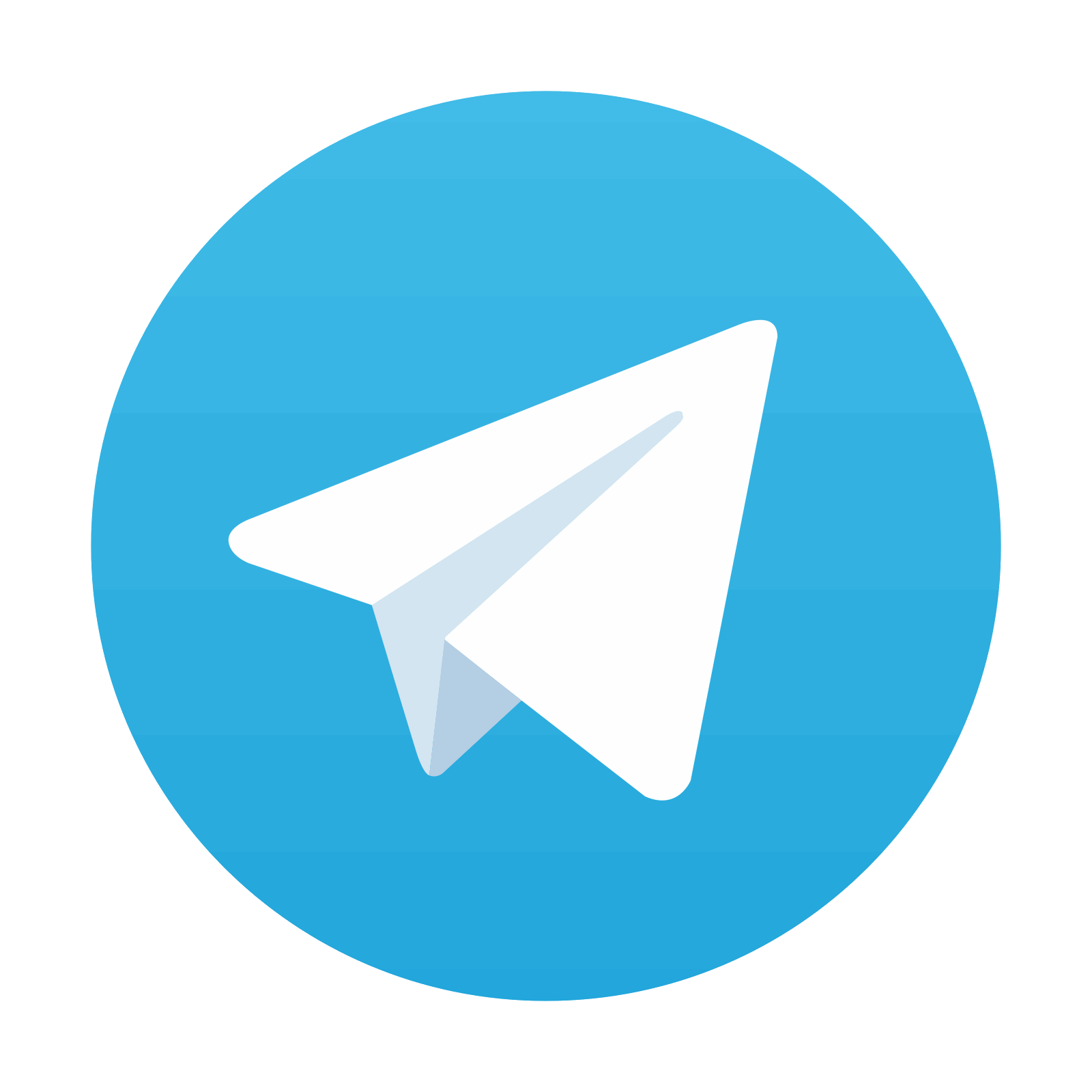
Stay updated, free articles. Join our Telegram channel

Full access? Get Clinical Tree
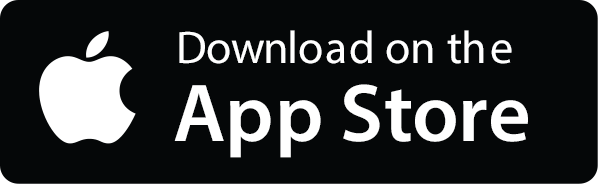
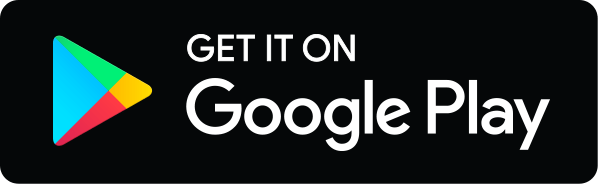